For additional ancillary materials related to this chapter, please visit thePoint.
Objectives
After studying this chapter, the student will be able to do the following:
1. Describe or define the key words and phrases.
2. Relate the physical properties of materials discussed in this chapter to their use in dentistry.
3. Define wetting. Include in the definition a drop of liquid and the contact angle formed with the surface.
4. Name the units of measure for the following properties:
- Density
- Heat capacity
- Stress
- Strain
- Modulus of elasticity
5. Define “proportional limit,” and name two other nearly equivalent terms.
6. Name the four types of stress, and provide an example of each found in everyday life.
7. Describe two situations in which dental materials are subjected to bending stresses when in function.
8. Compare the properties of “toughness” and “hardness,” and provide examples.
9. Explain the difference between stress relaxation and creep.
10. Discuss the phenomenon of stress concentration, and compare its effects on a poorly placed amalgam restoration as well as on a properly placed one.
Key Words/Phrases
abrasion resistance
bending
biologic properties
chemical properties
coefficient of thermal expansion
color
compression
creep
density
elastic deformation
elastic limit
elasticity
fatigue
force
fracture toughness
galvanic shock
Goldilocks principle
hardness
heat capacity
heat of fusion
heat of vaporization
load
mechanical properties
modulus of elasticity
percolation
permanent deformation
physical properties
plastic deformation
Poisson’s ratio
proportional limit
resilience
shade guide
shear
solubility
specific heat capacity
strain
stress
stress concentration
stress relaxation
tension
thermal conductivity
torsion
toughness
ultimate compressive strength
ultimate strength
ultimate tensile strength
vapor pressure
viscosity
water sorption
wetting
yield point
Introduction
When substituting one material for another, as in the restoration of teeth, we must be aware of the requirements for the new material. It seems obvious that the replacement material should have the same characteristics as enamel, which is very hard and strong. Should dentists restore teeth with the strongest and hardest material? In certain situations, the replacement material should have the same appearance as teeth for proper esthetics. Several other questions also need to be addressed: Is the material as good a thermal insulator as enamel and dentin are? Is the material “kind” to the pulp of the tooth? Does the material break down and release toxic chemicals?
We should also consider the cost of materials and labor when selecting restorative materials. As the list of requirements grows, we begin to realize that the criteria for an ideal restorative material are many and varied. Unfortunately, no ideal material exists, so we are always compromising in one way or another. The only sure way to eliminate this difficult dilemma is through prevention.
The important question is: what are the requirements for a particular restoration for a particular tooth and patient? Determining what is required and what will best meet the clinical situation are complex questions. Typically, a compromise is made among the many factors being considered. This chapter discusses many of these factors or properties of materials. In addition, this chapter describes the relationship between these properties and the materials science concepts previously discussed.
In everyday life, we notice that different materials have different properties. When we pick up an object, we feel its weight, which is an indication of its density. We also feel its temperature, which is an indication of its thermal “content” and its ability to transmit heat. Some objects feel strong and hard; others feel weak and flexible. Engineers have devised a variety of laboratory tests to quantify the properties of materials. Dental materials scientists use the same tests (as well as several others) to describe the physical properties of materials used in dentistry.
I. Properties of Materials
Properties of materials can be divided into three categories: physical, chemical, and biologic. Physical properties are based on the laws of physics that describe mass, energy, force, light, heat, electricity, and other physical phenomena. Color, density, and thermal conductivity are examples of physical properties. Mechanical properties are a subgroup of physical properties. Mechanical properties describe a material’s ability to resist forces. Mechanical properties are dependent on the amount of material and on the size and shape of the object. Examples are strength and stiffness. Chemical properties describe the setting reactions as well as the decay or degradation of materials. For example, gypsum products (used to make study models) set by a precipitation process, whereas dental composites polymerize. Biologic properties of materials are the effects the materials have on living tissue. For example, a crown should not irritate the gingiva, tongue, or buccal mucosa.
The chemical and biologic properties of dental materials are discussed with each group of materials presented in the chapters that follow. This chapter focuses on the physical and mechanical properties.
II. Physical Properties
A. Density
The amount or mass of a material in a given volume is the density of the material. A common unit of density is g/cm3. Density depends on the type of atoms that are present (as the atomic number increases, so does the density), the packing together of atoms and molecules, and the voids in the material. The high density of most metal objects makes them feel heavy. Most metals have high atomic numbers, and their atoms are packed closely together into solids. A metal maxillary partial denture will feel heavy and have a tendency to become “unseated” if it is not designed to adapt to the remaining teeth.
B. Boiling and Melting Points
Boiling and melting points are physical properties of materials. In analytical laboratories, they can be used to help identify chemicals. Mixtures often have a melting or boiling range rather than a specific melting or boiling point. Dental waxes are an example of mixtures with a melting range. When an object melts or boils, the atomic bonds between the atoms or molecules are broken by the thermal energy of the material. Some dental metals melt at very high temperatures and are very difficult to work with. Other materials do not boil or melt; instead, they decompose if heated sufficiently. Wood and cookie dough are common examples of materials that decompose.
C. Vapor Pressure
Vapor pressure is a measure of a liquid’s tendency to evaporate and become a gas. As the temperature of a liquid increases, the vapor pressure also increases. We notice that steam rises from a pot of water more and more as it is heated because the increased thermal energy allows more atoms or molecules to escape from the liquid and become vapor. Materials with a low vapor pressure, such as cooking oil, do not evaporate quickly. Materials with a high vapor pressure, such as rubbing alcohol, evaporate readily at room temperature.
Materials with a high vapor pressure are very useful as solvents in the application of viscous (syrupy) liquids, such as glue or paint. The viscous liquid is “thinned” by mixing it with a solvent. This “more runny” mixture is then applied to a surface. As the solvent evaporates, it leaves behind a thin layer of the viscous liquid. In dentistry, we use solvents to apply a thin layer of a thick liquid, such as copal varnish or a dentinal adhesive. Rubber cement, oil-based paints, and perfumes use the same process: a mixture is applied and the solvent evaporates, leaving behind a thin film of the desired substance.
Methyl methacrylate, a component of dental acrylic resins (plastics), has a high vapor pressure and can evaporate easily when a denture is processed. Porosity may result, weakening the denture. Denture-processing techniques are designed to minimize the evaporation of methyl methacrylate and the resulting porosity (see Fig. 11.3).
D. Thermal Conductivity
In the kitchen, we use a wooden spoon to stir hot liquids. We do not use a metal spoon because it conducts heat well and would quickly become too hot to hold. In dentistry, we are also interested in the thermal conductivity of materials. Thermal conductivity is the rate of heat flow through a material. Measurement of thermal conductivity depends on the distance the heat travels, the area in which the heat travels (much like the size of the pipe through which water travels), and the difference in temperature between the source and destination. Because thermal conductivity is a rate, it is measured as heat flow over time. Thermal conductivity is measured as calories/second·meter·degree. Pulpal sensitivity is likely if conductive materials, such as metals, are placed in close proximity to the pulp. If caries are deep and a metal restoration is planned, an insulating base is placed beneath the metal restoration to insulate the pulp from hot and cold stimuli (see Fig. 1.9).
E. Heat Capacity
Some objects, such as a “microwave warming trivet,” can store a lot of heat. The heat capacity of a material is a measure of the amount of thermal energy that a material can hoard. Some materials require more energy than others to heat. The specific heat capacity of a material is the amount of energy needed to raise the temperature of one unit of mass of that material by 1°C. Specific heat capacity is measured as cal/g·deg. The heat capacity of water is 1 cal/g·deg.
F. Heat of Fusion and Vaporization
The heat of fusion is the amount of energy required to melt a material. Conversely, the heat of vaporization is the amount of energy required to boil a material. Both are typically large in relation to the heat capacity. It requires 80 times more energy to melt a given quantity of ice than to increase the temperature of the same amount of water by 1°C. It takes 540 times more energy to boil that same quantity of water. The high heat of fusion allows a small amount of ice to effectively cool a much larger amount of beverage without excessive dilution as it melts. We shall see that the heat of fusion of metals must be overcome when melting gold for casting a crown. The solid metal must be heated to the melting temperature, and then, a significant amount of energy must be added to melt the metal.
G. Coefficient of Thermal Expansion
If a balloon filled with room temperature air is brought outside on a cold January day in West Virginia, the balloon shrinks. This is a visible example of what happens to nearly all materials when cooled: they shrink or contract. On the other hand, most materials expand when heated. The coefficient of thermal expansion is a measure of this change in volume in relation to the change in temperature. This concept, however, is slightly more complicated than that explanation suggests.
The coefficient of thermal expansion is a fractional change in volume or length. In Figure 3.1, several examples of expansion are illustrated. In Figure 3.1A, the 10-unit-long bar is extended by 1 unit (or 10%). The new bar is now 1.1 times the original length. In Figure 3.1B, the 5-unit-long bar is extended by 0.5 unit, becoming 5.5 units long. It is also 10% longer (or 1.1 times the original length). Therefore, larger objects of the same material expand more in quantity than smaller objects do, but larger objects expand by an equal amount in terms of the percentage or fractional change. In Figure 3.1C, a different material expands more, and the result is a 20% change in length. We look at fractional change of length again later in this chapter, when strain is discussed.
FIGURE 3.1. Three examples of expansion of a material in terms of percentage or fractional change in length.
Our interest in the coefficient of thermal expansion of dental materials is in relationship to those of enamel and dentin. A polymeric material, such as polymethyl methacrylate (an early tooth-colored restorative material of the 1950s), shrinks and expands seven times more than tooth structures. Compared with that filling material, the restorative materials of today more closely match the coefficient of thermal expansion of teeth. When the mismatch is great, the restoration will shrink with cold beverages, opening gaps between the restoration and the tooth. When the tooth heats up again and expands, the gap is closed. The process of heating and cooling, and the accompanying opening and closing of the gap, is called percolation. Percolation results in microleakage, tooth sensitivity, and recurrent decay. Microleakage is discussed in Adhesive Materials.
H. Electrical Conductivity
Electrical conductivity is not typically thought of as an important property, but we should know which materials are conductive. Metals are good electrical conductors. Polymers and ceramics are poor conductors and are termed insulators. During electrosurgery or electronic pulp testing, it is important to know which restorations are conductive and which are not. Electrical conductivity also affects corrosion, which will be discussed in the section on instruments. Occasionally, a new amalgam filling will hurt when it is touched with a metal fork. This galvanic shock is the result of electricity flowing from the fork to the amalgam and through the pulp.
I. Viscosity
When placing materials, the handling characteristics of those materials are important. Some materials should flow easily and wet the surface. Other materials need to be more like putty, which can be adapted or formed into a desired shape. The viscosity of a material is its ability to flow. Thick or viscous liquids flow poorly, whereas thin liquids flow easily. Viscosity is a temperature-dependent property. For example, pancake syrup pours much more easily when it is warmed. Viscosity is measured as g/m·s, or as poise (P). Water at 20°C has a viscosity of 0.01 P, or 1 centipoise (cP). Impression materials have viscosities between 100,000 and 1,000,000 cP.
A low viscosity and the ability to wet a surface are important in the use of many dental materials. Wetting a surface with an adhesive material, such as a sealant, brings the material into intimate association with the surface so that chemical and micromechanical bonding can occur. Wetting is measured by determining the contact angle of a liquid on a solid, as shown in Figure 3.2. A low-contact angle, such as that of a drop of water on a piece of ice, indicates good wetting. A high-contact angle, such as that of a drop of water on most plastics, indicates poor wetting. Another example of wetting in dentistry occurs when a gypsum product (plaster) is poured into an impression. If the mixed material wets the surface of the impression material, the fine details of the impression will be reproduced in the cast. If poor wetting occurs, bubbles will likely result in insufficient detail and an unusable cast.
FIGURE 3.2. Contact angle measurements demonstrate a liquid’s ability to wet a surface.
J. Hardness
Enamel is the hardest biologic tissue in the human body. Hard materials resist scratching and indenting by soft materials. Hardness is a property that is measured by scientific instruments that press a special tip into the surface of the test material. The tip has a characteristic shape made of a very hard material, such as steel or diamond, as shown in Figure 3.3. The size of the indentation created is then measured. Hardness is calculated based on the size of this indentation. Several different methods can be used to measure hardness. The Brinell and Knoop methods are illustrated in Figure 3.3. Two other methods are called the Rockwell and Vickers hardness tests. The Knoop hardness number (KHN) of enamel is 350, whereas the KHN of dentin is 70. Some dental materials are harder than enamel. Porcelain has a KHN of 400 to 500. Other materials are not as hard as enamel. For example, acrylic denture teeth have a KHN of 20.
FIGURE 3.3. Comparison of two hardness indenter tips, the Brinell and the Knoop. A. Perspective views of the two tips. B. Cross-section of the tips in contact with test samples. C. Test material showing the indentation that is measured to calculate hardness.
K. Durometer Measurements
Some materials are soft and even spongy. One example would be the rubber grip sometimes used on an instrument’s shaft. A different kind of hardness test is used for these materials because a surface hardness indentation will not occur. A durometer measures how deep a steel ball will sink when pressed into the surface of a soft material. Durometers are used to measure the hardness of impression materials and other elastic polymers.
L. Abrasion Resistance
Harder materials tend to be more resistant to abrasion than softer materials. In dentistry, we are interested in the abrasion resistance (wear resistance) of dental restorations to food, opposing teeth, and other dental materials such as ceramic crowns or porcelain denture teeth. We are also interested in the wear of natural teeth opposing dental restorations. If a restorative material is too hard, it will wear the opposing teeth at an unacceptably accelerated pace. An example is the excessive wear of natural teeth that oppose a denture with porcelain teeth. Therefore, a restoration must be hard enough so that the restoration does not wear away, but not so hard as to excessively wear away the opposing teeth. This is a good example of what has been called the Goldilocks principle: not too hard, not too soft, just right. More often than not, a material’s properties need to fit within a particular range of values, not the maximum value, to be the material of choice.
M. Solubility
Materials placed in the oral cavity are exposed to various aqueous fluids. The solubility of materials in water is an important consideration. Solubility is the amount of a material that dissolves in a liquid, such as water. Sugar is a highly soluble material, but enamel is not. Restorative materials should not appreciably dissolve in the mouth. Additionally, some materials tend to dissolve faster in acidic environments. To measure solubility, a test sample is immersed in water. The weight of the material dissolved into the water is the solubility of that material. The solubility of some dental cements is measurable and clinically significant. Excessive solubility leads to loss of material and increases the risk of recurrent decay.
N. Water Sorption
Some materials absorb water. This property is termed water sorption. Water sorption is measured much like solubility. A test sample is immersed in water, and the weight that is gained by that sample is the water sorption. A cookie dunked in milk is an example of milk sorption. When materials absorb water, they tend to swell. Some materials both dissolve and absorb water at the same time, making measurement of one of these properties difficult. Many polymers absorb a small amount of water over time and slightly swell as a result.
O. Color
The appearance of a restoration for an anterior tooth can be a factor when choosing materials. The color and surface luster of the restoration are important. In addition, the shape and health of gingival tissues adjacent to a restoration are also noticed.
Color is a complex phenomenon that is a psychological response to a physical stimulus. The physical stimulus is the light reaching the rods and cones of the eye. Because processing of that stimulus by the brain is a psychological phenomenon, the perception of color varies between individuals. Two people may agree that two objects match in color, while a third person disagrees. In addition, the color of an object depends on the light in which the object is viewed. For all these reasons, matching restorative materials to a patient’s teeth can be difficult.
Two types of systems are used to measure color. Both use three numbers to describe a color. One system involves matching the test object to color tabs resembling those available in a paint store. It is called the Munsell color system. Each tab has hue, chroma, and value numbers assigned. Hue is the fundamental color of an object, as in red, green, or blue. Chroma is the strength or color saturation of the hue, as in pink versus red. Value is the light or darkness of the color, as in shades of gray. In dentistry, esthetic materials have their own set of color tabs or shades, called a shade guide. Some manufacturers use a standard set, such as the VITA shade guide, which is shown in Figure 3.4. Recently, handheld devices have been developed that measure the color of teeth through a digital camera. These devices provide maps of the various shades of a tooth.
FIGURE 3.4. Photograph of several shade guides, including a VITA shade guide (top right).
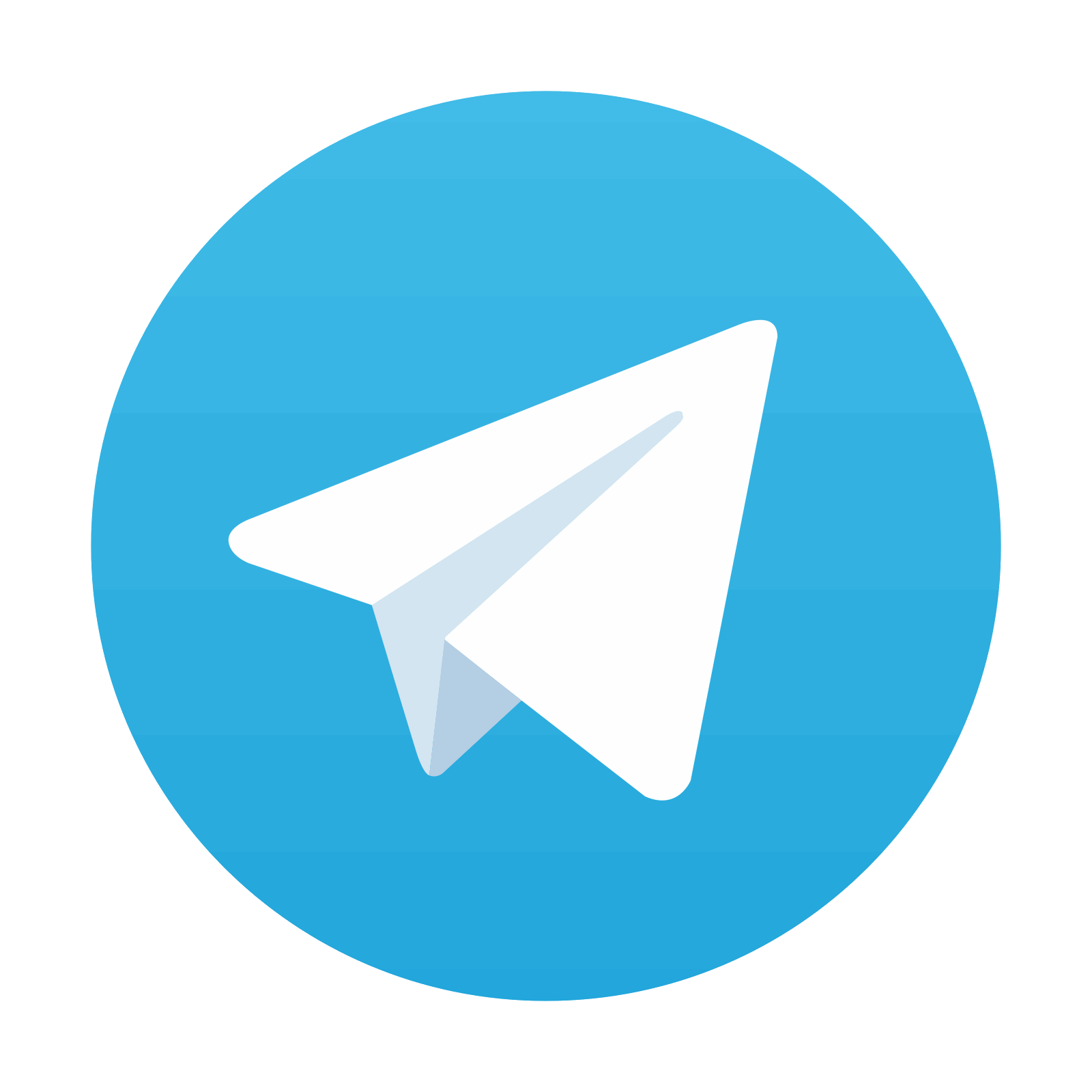
Stay updated, free dental videos. Join our Telegram channel

VIDEdental - Online dental courses
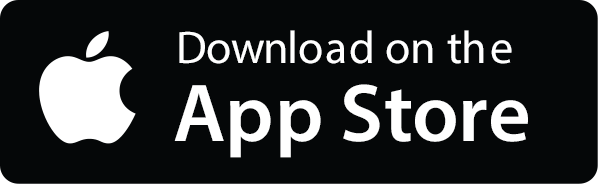
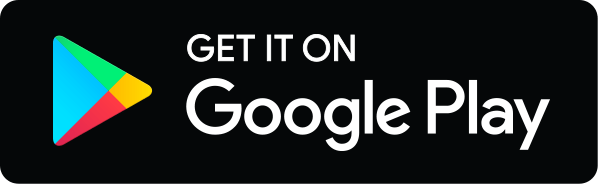