Pharmacology of Sedation Agents
Introduction
A sound understanding of the principles of the pharmacology of individual sedation agents is essential to the safe practice of sedation. It is important from the outset to specify exactly what is meant by a sedation agent, as there can be considerable overlap between drugs that produce both sedation and general anaesthesia. A drug used for sedation should:
- Depress the central nervous system (CNS) to an extent that allows operative treatment to be carried out with minimal physiological and psychological stress
- Modify the patient’s state of mind such that communication is maintained and the patient will respond to spoken command
- Carry a margin of safety wide enough to render the unintended loss of consciousness and loss of protective reflexes unlikely.
Current sedation practice should only use agents and techniques that satisfy the above criteria. Additionally, the agents themselves should have a:
- Simple method of administration
- Rapid onset
- Predictable action and duration
- Rapid recovery
- Rapid metabolism and excretion
- Low incidence of side effects.
Sedation agents are usually administered via inhalation, intravenous, oral or intranasal routes. The route of administration affects the timing of drug action, although ultimately all drugs arrive at their target cells in the brain via the bloodstream.
Inhalation agents have the advantage of being readily absorbed by the lungs to provide a rapid onset of sedation, followed by rapid elimination and recovery. Intravenous agents are predictably absorbed but once administered cannot be removed from the bloodstream. The therapeutic action of intravenous agents is terminated by re‐distribution, metabolism and excretion. Oral sedatives have a less certain absorption due to variability of gastric emptying and they therefore produce unpredictable levels of sedation. For drugs administered intranasally, the nasal cavity’s rich vascular plexus provides a direct route to the circulation by passage across the mucous membrane; this avoids gastrointestinal destruction and hepatic first pass metabolism and allows more drug to be rapidly and predictably available than administration via the oral route.
This chapter will primarily address the pharmacology of sedation agents currently used in inhalation and intravenous techniques. The pharmacology of the oral sedatives is not included in this chapter and will be covered in Chapter 5.
Inhalation Sedation Agents
Inhalation agents produce sedation by their action on various areas of the brain. They reach the brain by entering the lungs, crossing the alveolar membrane into the pulmonary veins, returning with the blood to the left side of the heart and then passing into the systemic arterial circulation. Thus, the two main components of inhalation sedation are:
- the entry of the inspired gas into the lungs, and
- distribution of the agent by the circulation to the tissues.
Basic Pharmacology of Inhalation Sedatives
Gas Solubility and Partial Pressure
During the induction of inhalation sedation, each breath of sedation agent raises the partial pressure of the gas in the alveoli. As the alveolar partial pressure rises, the gas is forced across the alveolar membrane into the bloodstream, where it is carried to the site of action in the brain. The gas passes down a pressure gradient from areas of high partial pressure to areas of low partial pressure (Figure 4.1). The level of sedation is proportional to the partial pressure of the agent at the site of action. After termination of gas administration the reverse process occurs. The partial pressure in the alveoli falls and the gas passes in the opposite direction out of the brain, into the circulation and then into the lungs.

Figure 4.1 Movement of nitrous oxide gas down the partial pressure gradient during induction and recovery from inhalational sedation.
The rate at which a gas passes down its pressure gradient is determined by its solubility. The solubility of a sedation agent (i.e. the blood‐gas partition coefficient) determines how quickly the partial pressure in the blood and, ultimately the brain, will rise or fall. The higher the partition coefficient, the greater the alveolar concentration of the agent needs to be to produce a rise in partial pressure in the blood and ultimately the tissues.
For the purposes of sedation, a gas with a low partition coefficient is preferred. Small concentrations of gas will produce a rapid rise in partial pressure and a fast onset of sedation. Similarly, after cessation of gas administration there will be a rapid fall in partial pressure and a fast recovery.
It is the inspired concentration of sedation agent that will determine the final level of sedation. The speed of induction of sedation is influenced by the rate of increase in gas concentration, as well as the minute volume and cardiac output of the patient. Any increase in minute volume, such as can be caused by asking the patient to take deep breaths, will increase the speed of onset of sedation.
Conversely, an increase in cardiac output will reduce the speed of induction of sedation. With a high cardiac output there is an increased volume of blood passing through the lungs. The sedation agent present in the lungs will be taken up into this larger volume of blood and the actual concentration of gas transported per unit volume of blood will be lower. Thus, less sedation agent will reach the brain and there will be a slower onset of sedation. The speed of recovery after termination of gas administration is similarly affected by the same factors.
Potency of Inhalation Sedation Agents
All sedation agents will produce general anaesthesia if used in high enough doses. The key to modern sedation practice is to ensure that the agents used have a wide enough margin of safety to render the unintended loss of consciousness unlikely. This means that there should be a considerable difference in the dose required to produce a state of sedation and the dose needed to induce general anaesthesia.
For inhalation anaesthetic agents the potency is expressed in terms of a minimum alveolar concentration (MAC). The MAC of an agent is the inspired concentration that will, at equilibrium, abolish the response to a standard surgical stimulus in 50% of patients.
Although the inspired concentration is measured as a percentage, the MAC is usually expressed as a number. Equilibrium is achieved when the tissue concentration of the gas equals the inspired concentration. MAC is a useful index of potency and is used to compare different anaesthetic gases.
Gases used for sedation should preferably have a moderate or high MAC and a low solubility. This will ensure a broad margin of safety between the incremental doses used to produce sedation and the final concentration required to induce anaesthesia. It would be very easy, using an agent with a small MAC for sedation, to accidentally overdose and anaesthetise a patient.
Types of Inhalation Sedation Agents
Nitrous Oxide
Nitrous oxide is the only inhalation agent currently in routine use for conscious sedation in dental practice. It was discovered by Joseph Priestly in 1772 and first used as an anaesthetic agent for dental exodontia by Horace Wells in 1844. Nitrous oxide has been used as the basic constituent of gaseous anaesthesia for the subsequent 170 plus years, demonstrating its acceptability and usefulness. In the 1930s, nitrous oxide was used for sedation purposes in the Scandinavian countries, particularly Denmark. However, it was not until the 1960s, when Harold Langa pioneered the modern practice of relative analgesia that nitrous oxide came into widespread use as an inhalation sedation agent in dentistry.
Presentation:
Nitrous oxide is a colourless, faintly sweet‐smelling gas with a specific gravity of 1.53. It is stored in light blue cylinders in liquid form at a pressure of 750 pounds per square inch (43.5 bar).
The gas is sold by weight and each cylinder is stamped with its empty weight. For example an E cylinder of nitrous oxide weighs 5.9 kg when empty and 8.8 kg when full. As the contents of the cylinder are liquid, the pressure inside, as measured by the pressure gauge on the inhalational sedation machine, will remain constant until nearly all the liquid has evaporated. The value shown on the gauge does not decrease in a linear fashion and tends to fall rapidly immediately before the cylinder becomes empty (Figure 4.2).

Figure 4.2 The pressure in the nitrous oxide cylinder remains constant and tends to fall rapidly immediately before the cylinder becomes empty.
Thus, the only reliable means of assessing the amount of nitrous oxide in a cylinder is to weigh the cylinder and compare the value with the weight of the empty cylinder. It can also be tapped with a metal instrument by those with musical ears; the pitch of the note falls as the gas is used. In addition, after prolonged use, the evaporation of the liquid nitrous oxide causes ice crystallisation on the cylinder at the level of the liquid within, thereby providing a third indication as to the nitrous oxide volume remaining in the cylinder.
Blood/Gas Solubility:
Nitrous oxide has a low blood‐gas partition coefficient of 0.47, so it is relatively insoluble and produces rapid induction of sedation. A further consequence of the poor solubility is that, when administration is discontinued, nitrous oxide dissolved in the blood is rapidly eliminated via the lungs. During the first few minutes of this elimination, large volumes of nitrous oxide pour out of the blood and into the lungs. This can actually displace oxygen from the alveoli causing a condition known as diffusion hypoxia. This occurs because the volume of nitrous oxide in the alveoli is so high that the patient effectively ‘breathes’ 100% nitrous oxide. For this reason, the patient should receive 100% oxygen for a minimum period of 3 minutes after the termination of nitrous oxide sedation. In reality, the risk of diffusion hypoxia is minimal due to the high level of oxygen delivered by dedicated inhalation sedation machines.
Potency:
Nitrous oxide has a theoretical minimum alveolar concentration (MAC) of approximately 105%. The high MAC means that nitrous oxide is a weak anaesthetic which is readily titrated to produce sedation. Because the MAC is over 80%, it is theoretically impossible to produce anaesthesia using nitrous oxide alone, at normal atmospheric pressure, in a patient who is adequately oxygenated. However, caution should be exercised when using inhaled concentrations of nitrous oxide over 50%, because even at this relatively low percentage, some patients may enter a stage of light anaesthesia.
Sedation:
Nitrous oxide is a good, but mild sedation agent producing both a depressant and euphoriant effect on the CNS. It is also a fairly potent analgesic. A 50% inhaled concentration of nitrous oxide has been equated to that of parenteral morphine injection at a standard dose (10 mg in a 70 kg adult). It can be used to good effect to facilitate simple dentistry in patients who are averse to local analgesia and it decreases the pain of injections in those who require supplemental local anaesthesia. Nitrous oxide has few side effects in therapeutic use. It causes minor cardio‐respiratory depression, and produces no useful amnesia.
Occupational Hazards of Nitrous Oxide:
The main problems associated with the use of nitrous oxide relate not to the patient but to the staff providing sedation, and the potential hazards relating to chronic exposure. It has been shown that regular exposure of healthcare personnel to high levels of nitrous oxide over long periods of time can cause specific illnesses, the most common effects being haematological disorders and reproductive problems.
It is well known that nitrous oxide causes the oxidation of vitamin B12 and affects the functioning of the enzyme, methionine synthetase required for DNA synthesis (Figure 4.3). This in turn impairs cellular production and can lead to various health issues (Table 4.1).

Figure 4.3 Biochemical effect of chronic exposure to nitrous oxide.
Table 4.1 Health risks of chronic exposure to nitrous oxide.
Impaired Cellular Production | Health Effects |
Haematological System | Pernicious Anaemia Impaired bone marrow function |
Neurological System | Myelinopathies including:
|
Reproductive System | Miscarriage Spontaneous Abortion Reduced fertility |
Hepatic and Renal | Impaired function Malignancy |
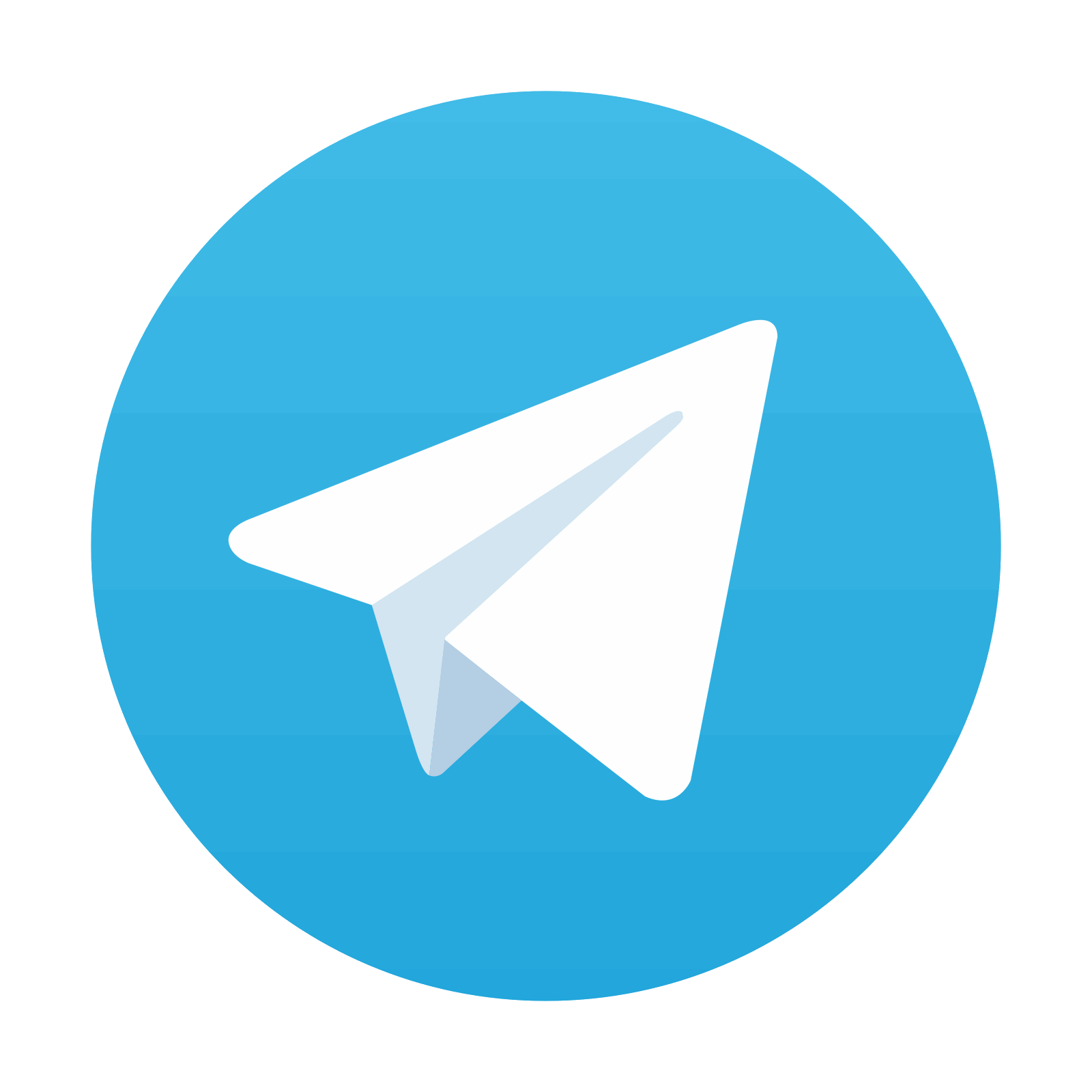
Stay updated, free dental videos. Join our Telegram channel

VIDEdental - Online dental courses
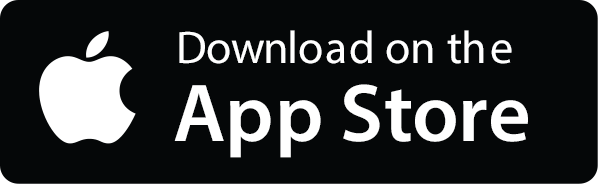
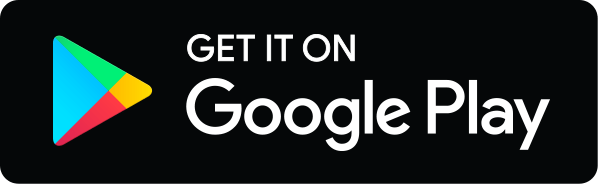