Applied Anatomy and Physiology
Introduction
An integrated approach to the anatomy, physiology and, to some extent, the pharmacokinetics of the drugs as they relate to sedation is essential to establish a basis for safe clinical practice. Sound clinical practice must be based on a solid foundation of basic medical science and the following sections address the relevant anatomy, physiology and general pharmacokinetics of sedation. (The pharmacology of the individual agents used for sedation is found in Chapter 4.)
All sedatives produce their effects by acting on the brain. The mode of action of a drug is referred to as its pharmacodyanamics, and these are the results of the activity of the drug on the central nervous system. They are essentially the same whether a drug is given orally, intravenously or by inhalation. It is, therefore, important to have an understanding of applied cardiovascular and respiratory anatomy and physiology relevant to conscious sedation.
Cardiovascular System
The cardiovascular system is a circulatory system comprising the heart, blood vessels and the cells and plasma that make up the blood. The blood vessels of the body represent a closed delivery system, which transports blood around the body, circulating substances such as oxygen, nutrients and hormones to the organs and tissues. The circulatory system also acts to remove metabolic wastes such as carbon dioxide and other unwanted products. The heart is a specialised muscle, the principal function of which is to act as a pump to maintain the circulation of blood within the blood vessels. The three main types of blood vessel are arteries, veins and capillaries.
Arteries
The afferent blood vessels carrying blood away from the heart. The walls (outer structure) of arteries contain smooth muscle fibres that contract and relax in response to the sympathetic nervous system.
Veins
The efferent blood vessels returning blood to the heart. The walls (outer structure) of veins consist of three layers of tissues that are thinner and less elastic than the corresponding layers of arteries. Veins include valves that aid the return of blood to the heart by preventing blood from flowing in the reverse direction.
The basic structure of the vessel wall (see Figure 2.1) is similar in all blood vessels with the tunica intima or endothelium lining the vessel’s lumen. Externally is a connective tissue, the tunica adventitia which is slightly thicker in arteries. The middle layer is a layer of smooth muscle, the tunica media, which is much thicker in arteries and which is largely responsible for the peripheral control of blood pressure. The endothelial lining of veins is enveloped to form valves which, with external muscle influence, assist in propelling blood back to the heart (valves are rarely taken into consideration during venepuncture, but they can be used to benefit or to hinder successful cannulation of a vein).

Figure 2.1 Transverse section of an artery and vein. A, Artery, lined with nucleated endothelium (e). Underneath the endothelium is the elastic lamina muscle layer (m). The muscle layer is surrounded by connective tissue fibres, the adventitia (a). V, Vein, has thin endothelial lining (e), under which is a very thin muscle layer (m). The adventia (a) is similar to the artery.
Capillaries
These are narrow, thin‐walled blood vessels (approximately 5–20 µm in diameter) that connect arteries to veins. Capillary networks exist in most of the tissues and organs of the body, and the narrow cell walls allow exchange of material between the contents of the capillary and the surrounding tissue. The networks are the site of gas, nutrient and waste exchange between the blood and the respiring tissues.
The Heart
The heart is composed of cardiac muscle; involuntary muscle tissue only is found within this organ. It is a small but complex organ. The left side of the heart delivers oxygenated blood, via the aorta, to the systemic circulation. The right side of the heart receives deoxygenated blood (Figure 2.2).

Figure 2.2 Cross‐section of the heart illustrating the flow of blood through the chambers and large vessels.
Cardiac Cycle
The cardiac cycle is defined as the sequence of pressure and volume changes that take place during cardiac activity. The time of a cycle in a healthy adult is approximately 0.9 seconds, although it varies considerably, giving an average pulse rate of around 70 beats per minute (bpm). There are two elements of the cardiac cycle:
- Systole: rapid contraction of heart, 0.3 sec
- Diastole: resting phase, 0.5 sec.
Heart Rate (HR)
The number of ventricular contractions occurring in one minute.
Stroke Volume (SV)
The volume of blood ejected in one ventricular contraction, approximately 70 ml.
Cardiac Output (CO)
The amount of blood ejected from one ventricle during one minute (i.e., stroke volume × heart rate). The cardiac output of the right ventricle passes through the lungs, while the output from the left ventricle passes into the aorta and is distributed to the organs and tissues.
The cardiac output is a product of stroke volume and heart rate described by the following equation: CO = SV × HR and is directly affected by three factors:
- Filling pressure of the right side of the heart
- Resistance to outflow (peripheral resistance)
- Functional state of the heart‐lung unit.
Conduction System
The aim of the conduction system is to enable atrial and ventricular contraction to be coordinated efficiently. Contraction or depolarisation of the heart is initiated via impulses generated in the sinoatrial node (SAN) and conducted through adjacent atrial muscle cells, causing systole in both atria. The depolarisation continues on to the atrioventicular node (AVN). These two nodes have their own inherent rhythm of: SAN 80 bpm and AVN 40 bpm. The AVN conducts the impulse on via the Bundle of His to the ventricles. These nerves divide into Purkinje fibres throughout the ventricles, and the result is to depolarise the whole ventricle (Figure 2.3).

Figure 2.3 Conduction system of the heart: 1 – Sinoatrial node; 2 – Atrioventricular node; 3 – Bundle of His; 4 – Bundle branches; 5 – Purkinje fibres.
The SAN is considered to be the heart’s pacemaker and is under the influence of the sympathetic and parasympathetic nervous systems. The parasympathetic system (via the Vagus nerve) acts to slow the heart while the sympathetic system increases the heart rate and volume intensity.
As well as the nervous and chemical stimulation, there are hormonal influences on the cardiovascular system. The kidneys produce renin which converts to angiotensin II, which is an extremely powerful vasoconstrictor. In addition, the adrenal medulla can produce central release of catecholamines, which simulate the action of the β receptors and induce sympathetic stimulation of the heart. Finally, there is a hormone released by the vessel endothelium known as endothelium‐derived relaxing factor (EDRF) which causes vasodilatation.
Thus, control of the cardiovascular system can be seen to consist of a highly complex series of mechanisms that can easily be disturbed by external factors such as sedation. In young and healthy individuals, the compensatory mechanisms are more than adequate to deal with this, but in the frail and elderly cardiovascular problems develop much more readily and allowance should always be made for this. This may also be true for those recovering from serious illnesses or who may be debilitated for any other reason.
Heart Rate
The heart rate will vary depending on age, anxiety and the presence of systemic pathology. Average heart rates are illustrated in Table 2.1.
Table 2.1 Average heart rates.
Age | Av. HR | Lower limit | Site |
Infant <1 yr | 120 | 60 | brachial |
Child <8 yr | 100 | 50 | carotid |
Adult | 72 | 40/50 | carotid |
Tachycardia
Tachycardia refers to a rapid heart rate (>100 bpm in adults). Tachycardia may be a perfectly normal physiological response to stress or exercise. However, depending on the mechanism of the tachycardia and the health status of the patient, tachycardia may be harmful and require medical treatment.
Tachycardia can be harmful in two ways. First, when the heart beats too rapidly, it may pump blood less efficiently. Second, the faster the heart beats, the more oxygen and nutrients it requires. As a result, the patient may feel out of breath or, in severe cases, suffer chest pain. This can be especially problematic for patients with ischaemic heart disease.
Bradycardia
Bradycardia is defined as a resting heart rate <60 bpm in adults. It is rarely symptomatic until the rate drops below 50 bpm. It is quite common for trained athletes to have slow resting heart rates, and this should not be considered abnormal if the individual has no associated symptoms.
Bradycardia can result from a number of causes which can be classified as cardiac or non‐cardiac. Non‐cardiac causes are usually secondary, and can involve drug use or misuse; metabolic or endocrine issues (especially related to the thyroid), neurologic factors, and situational factors such as prolonged bed rest. Cardiac causes include acute or chronic ischaemic heart disease, vascular heart disease or valvular heart disease.
The blood is driven through the vascular system by the pressure produced on ejection of the blood from the ventricles followed by the elastic response of the major arteries (Figure 2.4).

Figure 2.4 Circulation of blood through the vascular system.
Blood Pressure
Blood pressure refers to the force exerted by circulating blood on the walls of blood vessels. It is a function of cardiac output and peripheral vascular resistance. Blood pressure is important as it maintains blood flow to and from the heart, the brain, kidneys and other major organs and tissues.
The systolic pressure is defined as the peak pressure in the arteries, which occurs near the beginning of the cardiac cycle. The diastolic pressure is the lowest pressure (at the resting phase of the cardiac cycle).
Typical values for a resting, healthy adult human are approximately 100–130 mmHg systolic and 60–85 mmHg diastolic (average 120/80 mmHg). These measures of blood pressure are not static but undergo natural variations from one heartbeat to another and throughout the day. They also change in response to stress, nutritional factors, drugs or disease. Hypertension refers to blood pressure being abnormally high; hypotension, when it is abnormally low.
Control of Blood Pressure
Blood pressure (BP) is affected by the peripheral vascular resistance (PR) and the cardiac output (CO). Peripheral resistance results from the natural elasticity of the arteries and is an essential feature of the circulatory system. When the heart contracts, blood enters the arteries faster than it can leave, resulting in the arteries stretching from the pressure. As the reverse pressure begins to exceed the ejectory pressure, the aortic valve closes and the atria refill.
The factors affecting blood pressure are many and include:
- Baroreceptor mechanism
- Carbon dioxide
- Hypoxia and chemoreceptors
- Respiratory centre
- Sensory nerves
- Higher centres
- Drugs.
Each of these will be briefly considered.
- Baroreceptor mechanism: Baroreceptors are pressure receptors found in the aortic arch and carotid sinus. Increased baroreceptor activity inhibits vasomotor centre (VMC) activity in the brain, leading to arterial vasodilatation, a lowering in PR and a consequent fall in BP. Similarly, decreased baroreceptor activity disinhibits VMC activity leading to arterial vasoconstriction, a rise in PR, with a corresponding rise in BP. Receptors can also be stimulated artificially, for example external pressure on the neck by high shirt collars.
- Carbon dioxide: Carbon dioxide (CO2) is essential for the functioning of the VMC. A decrease in CO2 leads to decreased VMC activity and a fall in BP, with an increase in CO2 having the opposite effect.
- Sensory nerves: Pain modifies the activity of the VMC, with mild pain increasing VMC activity, leading to an increase in BP. Severe pain decreases VMC activity and may lead to a drop in BP. In this situation, the body is acting in a protective way. The mechanisms by which this occurs are complex.
- Higher centres: Emotional stress or excitement often increases BP by affecting the VMC and also increases cardiac output. In emotional shock there may be a fall in BP, e.g. at the sight of blood.
- Drugs: The majority of anaesthetic and sedative drugs cause a drop in BP by reducing the brain’s ability to respond to stimuli to change BP, and the muscle relaxant effect therefore leads to a reduction in PR. It is, therefore, essential to monitor blood pressure throughout procedures involving general anaesthesia or sedation.
Irregularities in Blood Pressure
Hypertension (High Blood Pressure)
Hypertension exists when the blood pressure is chronically elevated. It is defined as values > 140 mmHg Systolic Blood Pressure (SBP) and/or > 90 mmHg Diastolic Blood Pressure (DBP).
Degrees of hypertension are recognised and classified as in Table 2.2.
Table 2.2 Definition and classification of blood pressure levels (mmHg).
Category | Systolic | Diastolic | |
Optimal | <120 | and | <80 |
Normal | 120–129 | and/or | 80–84 |
High Normal | 130–139 | and/or | 85–89 |
Grade 1 Hypertension | 140–159 | and/or | 90–99 |
Grade 2 Hypertension | 160–179 | and/or | 100–109 |
Grade 3 Hypertension | ≥180 | and/or | ≥110 |
Isolated Systolic Hypertension | ≥140 | and | <90 |
The blood pressure (BP) category is defined by the highest level of BP, whether systolic or diastolic. Isolated systolic hypertension should be graded 1,2 and 3 according to the systolic BP in the ranges indicated
Predisposing factors include:
- Age (blood pressure rises with age)
- Obesity
- Excessive alcohol intake
- Genetic susceptibility.
Hypotension (Low Blood Pressure)
Hypotension results if the systolic blood pressure falls below 90 mmHg. It often presents with the features of shock, including tachycardia and cold and clammy skin. The common symptoms of hypotension are light‐headedness and dizziness and, if the blood pressure is sufficiently low, syncope (fainting) often occurs. This situation is not uncommon in the dental surgery and is normally easily managed.
Low blood pressure in patients presenting at assessment may be due to autonomic failure as a result of drugs that interfere with autonomic function, for example tricyclic antidepressants, or drugs that interfere with peripheral vasoconstriction including nitrates and calcium antagonists.
Importance of Blood Pressure in the Dental Patient
Dental treatment is perceived as a stressful situation by many patients and in this situation blood pressure may be elevated. This becomes an issue mainly in patients with underlying cardiovascular disease and can predispose to cardiovascular events such as myocardial infarction, and stroke.
Vascular Anatomy of Upper Limb Relevant to Sedation
An understanding of the anatomy of the arm is important since the most commonly used veins for cannulation are the superficial veins of the dorsum of the hand (Figure 2.5), and the anticubital fossa (Figure 2.6).

Figure 2.5 Veins of the dorsum of the hand.

Figure 2.6 Antecubital fossa illustrating the three important structures to be aware of: brachial artery, median nerve and bicipital aponeurosis.
It is important to note that in the antecubital fossa (Figure 2.6) there are three important structures that must be avoided:
- The brachial artery
- The median nerve
- Bicipital aponeurosis.
Fortunately, all three are to be found on the medial aspect of the fossa and so injections are placed lateral to the easily palpable biceps tendon in order to avoid these structures.
Route of Drug Transfer to the Brain
On injecting the IV sedation drug it travels in the venous circulation to the heart and is then distributed through the arterial system to the brain where it has its principal effect.
The actual route taken is as follows: After the drug is injected into the dorsal veins it passes into the cephalic and basilic veins to the median vein, brachial and axillary veins to reach the subclavian vein; from here it travels through the brachiocephalic vein to the superior vena cava and into the right atrium. The drug then passes through to the right ventricle and through the pulmonary artery to the lungs.
Following oxygenation of the blood in the lungs the drug will pass back through the pulmonary veins to the left atrium and into the left ventricle. Travelling through the aorta the sedation agent will reach the GABA receptors in the brain via the carotid and cerebral arteries where it has its effect.
Respiratory System
The respiratory system facilitates oxygenation of the blood with a concomitant removal from the circulation of carbon dioxide and other gaseous metabolic waste. Anatomically, the respiratory system consists of the nose, pharynx, larynx, trachea, bronchi and bronchioles. The bronchioles lead to the respiratory zone of the lungs which consists of respiratory bronchioles, alveolar ducts and the alveoli, the multi‐lobulated sacs in which most of the gas exchange occurs.
Upper Airway
The upper airway consists of the nose and pharynx. The pharynx is divided into three sections: nasopharynx, oropharynx and laryngopharynx (Figure 2.7).

Figure 2.7 Upper airway.
Lower Airway
The lower airway (Figure 2.8) consists of the:
- Larynx – The mucosa of the larynx (voice box) is very sensitive, and if irritated the cough reflex is initiated by the strong muscles surrounding the structure. This acts as a protective mechanism preventing the entry of foreign objects.
- Trachea – The trachea is a continuation of the larynx beginning at the level of the sixth cervical vertebra. It is approximately 11 cm long with a diameter of 20 mm. The trachea bifurcates into the right and left bronchi.
- Bronchi – The left bronchus emerges at an angle of approximately 45 degrees from the trachea. The right bronchus branches off at an angle of 25 degrees; it is approximately 2.5 cm in length and, for this reason, inhaled foreign bodies tend to be directed to the right lung. The main bronchi then divide into smaller branches to supply the lobes of the lungs.
- Bronchioles – The bronchioles are a continued division of the bronchi which themselves divide further into the alveolar ducts, alveolar sacs and alveoli. It is within the capillary beds of the alveoli that exchange between air and carbon dioxide in the blood occurs.

Figure 2.8 Lower airway.
Respiration
The process of respiration consists of external and internal mechanisms.
- External respiration – where there is an exchange of gases between lungs and blood
- Internal respiration – involving exchange of gases between blood and cells.
With an inhalation sedation agent, the gas must enter the lungs, cross the alveolar membranes to be absorbed into the blood, be pumped round the left side of the heart into the arterial blood before reaching the tissues of the body. There are, therefore, three aspects of this process: entry into the lungs; circulation to the tissues; and excretion or removal from the body.
Control of Respiration
Ventilation of the lungs is carried out by the muscles of respiration and is under the control of the autonomic nervous system from part of the brain stem, the medulla oblongata and the pons. This area of the brain forms the respiration regulatory centre (Figure 2.9).

Figure 2.9 The control of respiration is influenced at several points. At point A, the respiratory centre is affected by all modern sedatives. At points B and C, the phrenic nerve and neuromuscular junction respectively, the influences are less profound.
This control centre receives information from a variety of sources including other brain receptors, the lungs, the blood vessels and the respiratory muscles. In addition, the respiratory centre receives information from various chemoreceptors in the medulla which monitor the pH of the cerebrospinal fluid. Changes in pH are largely influenced by the rise and fall of carbon dioxide levels since increased carbon dioxide (CO2) availability leads to an increase in hydrogen ion availability (and a lowering of pH) as carbonic acid forms.

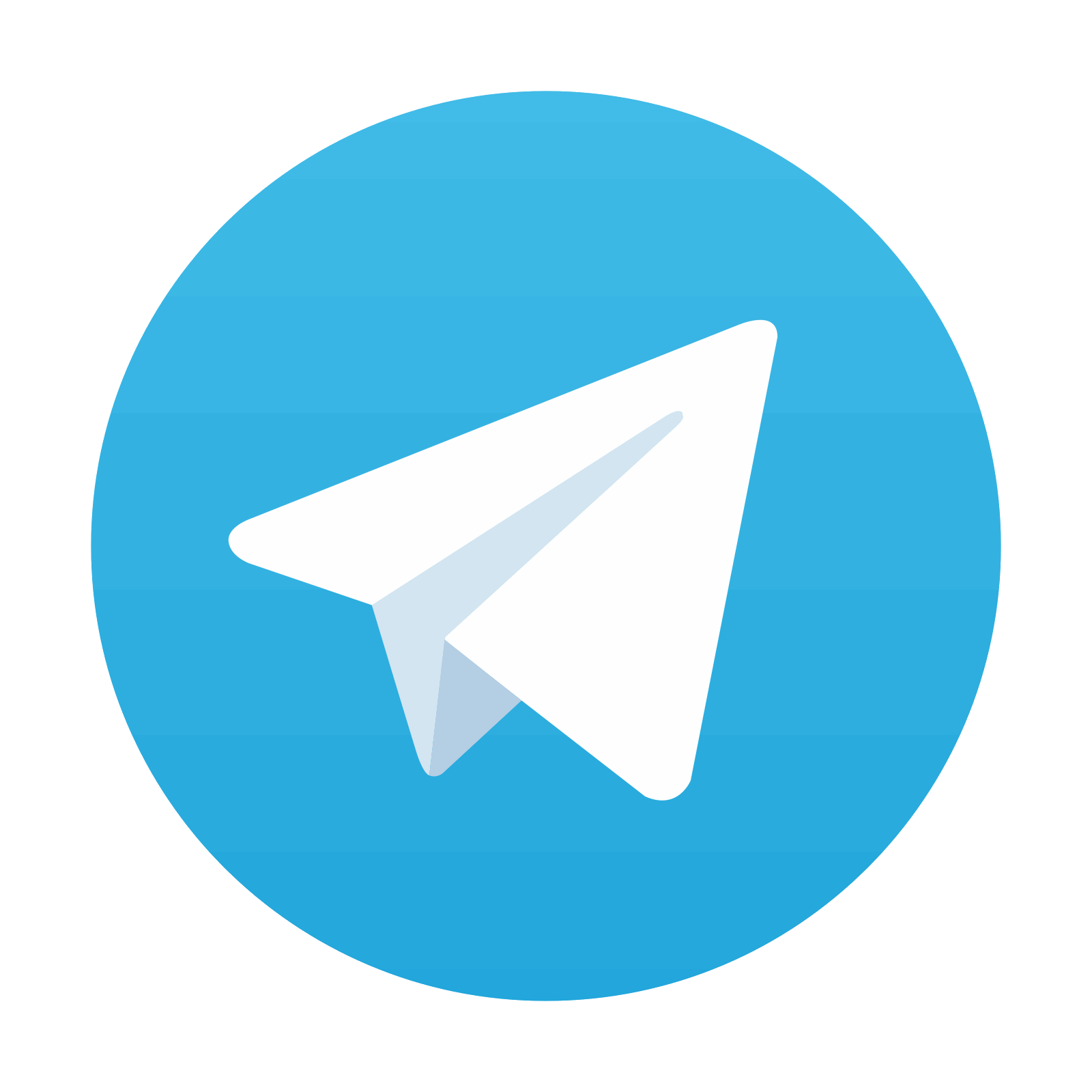
Stay updated, free dental videos. Join our Telegram channel

VIDEdental - Online dental courses
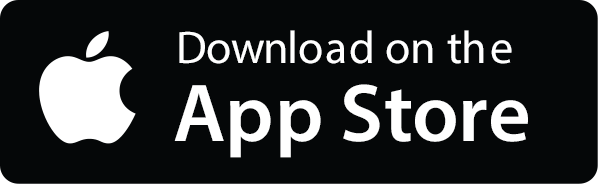
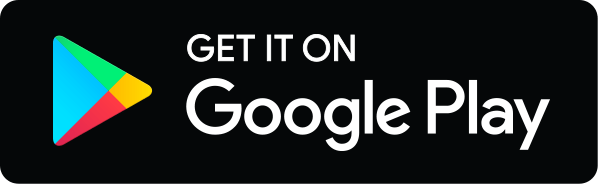