Graphical abstract

Abstract
Objectives
Candida albicanscolonizes biomaterial surfaces and are highly resistant to therapeutics. Graphene nanocoating on titanium compromises initial biofilm formation. However, its sustained antibiofilm potential is unknown. The objective of this study was to investigate the potential of graphene nanocoating to decrease long-term fungal biofilm development and hyphae growth on titanium.
Methods
Graphene nanocoating was deposited twice (TiGD) or five times (TiGV) on grade 4 titanium with vacuum assisted technique and characterized with Raman spectroscopy and atomic force microscope. The biofilm formation and hyphae growth of C. albicans was monitored for seven days by CFU, XTT, confocal, mean cell density and scanning electronic microscopy (SEM). Uncoated titanium was the Control. All tests had three independent biological samples and were performed in independent triplicates. Data was analyzed with one- or two-way ANOVA and Tukey’s HSD (α = 0.05).
Results
Both TiGD and TiGV presented less biofilms at all times points compared with Control. The confocal and SEM images revealed few adhered cells on graphene coated samples, absence of hyphae and no features of a mature biofilm architecture. The increase in number of layers of graphene nanocoating did not improve its antibiofilm potential.
Significance
The graphene nanocoating exerted a long-term persistent inhibitory effect on the biofilm formation on titanium. The fewer cells that were able to attach on graphene coated titanium were scattered and unable to form a mature biofilm with hyphae elements. The findings open opportunities to prevent microbial attachment and proliferation on implantable materials without the use of antibiotics.
1
Introduction
Peri‐implantitis is highly prevalent and may affect up to 14% of the patients (crestal bone loss >2 mm) after 9 years of loading [ ]. Under certain circumstances, the surgical removal of the implant from the infected site is the only solution [ ]. Hence, implant-associated infections are a significant clinical problem.
Candida albicans is the most common fungal pathogen in humans causing both mucosal infections as well as systemic mycoses [ ]. C. albicans is able to adhere and colonize biomaterials and is hence considered a major pathogen for implant and device-associated infections [ ]. Once adhered, C. albicans gradually forms a surface-attached community known as a biofilm [ ]. Concerns have been increasing about the contribution of fungal species to dental implant diseases as these microorganisms have been identified on implant surfaces and in 31% of peri-implantitis sites [ , ]. Candida species have been identified at least five times more frequently in biofilms from patients suffering from peri-implantitis compared with healthy individuals [ , ]. Notably, C. albicans was present in 80% of patients with peri-implantitis where yeast species have been identified [ ]. In addition, there is a concern that dental implants may be a potential reservoir for C. albicans associated infections [ ].
Candida albicans biofilms are highly resistant to antifungal treatments and directly associated with therapeutic failure and mortality [ , ]. Considering this clinical problem, there have been numerous efforts to develop surface coating strategies to prevent biofilm formation on biomaterials. For instance, coatings containing silver present antimicrobial activity but are susceptible to oxidative dissolution and are not specific in damaging microorganisms only [ , ]. Hydroxyapatite and chitosan doped with antibiotics can decrease infection on titanium [ , ] but these materials have low mechanical properties and the use of antibiotics raises concerns over antimicrobial resistance [ ]. Therefore, surface coating materials with tunable properties that reduce microbial adhesion and biofilm formation are preferred over antimicrobial agents.
Graphene is an one-atom thick two-dimensional material made of carbon atoms arranged in a honeycomb structure. It has very high mechanical strength, stiffness and impermeability [ , ]. Previously, we have shown that graphene nanocoating produced by chemical vapor deposition is cytocompatible and promotes osteoblastic differentiation and bone formation on titanium [ , ]. Moreover, we found it is an effective barrier against the oxidation and corrosion of Ti-6Al-4V alloys [ ]. The nanocoating can be deposited on different biomedical materials without the use of hazardous chemicals maintaining high structural stability and quality upon exposure to water, biomolecules, cells and extremely harsh acidic environments [ , ]. Hence, graphene nanocoating emerges as a promising material for improving implants [ , ].
When a Candida biofilm forms in vivo , it becomes nearly imperative to remove it from the substrate it is attached to eradicate the infection. However, the removal can be impaired due to the body anatomy, implant design and location [ ]. Thus, implant surfaces that compromise biofilm formation in first place are advantageous. Increased surface hydrophobicity has been shown to hamper the adhesion of microorganisms to biomedical materials and delay biofilm maturation in vivo [ ]. The hydrophobic properties imparted to a material surface by an atom-thin graphene film have been shown to compromise the early stages of biofilm formation [ ]. Although graphene increases the hydrophobicity of substrates, it is not entirely transparent to wetting and the hydrophobicity can be magnified by increasing the number of graphene transfer procedures [ , ]. Nevertheless, the effects of number of graphene layers on long-term biofilm formation remains largely unknown.
Despite the promising potential, it remains to be determined whether graphene nanocoating provides sustainable long-term protection for implant surfaces against fungal pathogens such as C. albicans . Hence, in the present study we aimed at i) examining the properties of graphene nanocoated titanium implants in inhibiting C. albicans biofilm formation over an extended period of time and ii) evaluating the effects of the number of graphene transfer procedures on the development of C. albicans biofilms. The tested hypothesis was that graphene nanocoating prevents the biofilm formation of C. albicans regardless of the number of transfer procedures.
2
Material and methods
2.1
Sample preparation
Medical grade 4 titanium discs (Control, 12 mm diameter, 1 mm thick, Vulcanium, USA) were polished with silicon carbide paper (up to 2500 p, 150 rpm, 20 N) and washed in ultrasonic bath with acetone, isopropanol and deionized water (20 min each). Graphene as grown by chemical vapour deposition on copper by a single operator using a custom-built furnace Class 1000 clean room facility as previously described by our group [ , ]. Thereafter, the graphene coated copper foil was covered with polydimethylsiloxane (PDMS, Sylgard 184, Dow Corning Corporation, USA), spin coated at 1000 rpm and cured (135 °C, 20 min). A polyimide tape (3 M, USA) was placed on top of the PDMS and the copper foil was etched away in a 1.5% ammonium persulfate solution. The transfer tape (polyimide/PDMS/graphene) was washed in deionized water (2 h) and blow-dried with nitrogen. Afterwards, the transfer tape was placed on the titanium disc, positioned in between two prefabricated PDMS molds and placed in a vacuum chamber and the pressure for 60 s at 0.02 bar. Finally, the PDMS molds and the transfer tape were removed leaving the graphene over the titanium disc. The transfer procedures were repeated twice or five times for TiGD and TiGV, respectively. Ten samples were characterized by Raman spectroscopy (Raman Microscope CRM 200, Witec, Germany) for the identification of G (∼1580 cm −1 ), D (∼1354 cm −1 ) and 2D peaks (2680 cm −1 ). The surface features and arithmetic average of the roughness profile (R a ) were characterized by atomic force microscope (n = 5, Bruker AXS, Germany) using tapping mode with a silicon nitride tip (resonance frequency: 40−90 kHz, spring constant 0.4 N/m).
2.2
Microbial culture and biofilm formation
Standard well-characterized strain of C. albicans SC5314 was subcultured on glucose minimal medium (GMM) agar plates for routine use. C. albicans was taken from GMM agar plates, inoculated and subjected to incubation for 18 h at 30 °C in orbital shaker incubator (ES-20 Biosan, Singapore) at 80 rpm. Cell cultures were then centrifuged at 5000 rpm for 5 min at 4 °C to pellet cells. The pellets were then re-suspended in 1 ml of new medium (GMM) and adjusted to an optical density of 0.375 to 0.385 at a wavelength of 520 nm, equivalent to 10 7 cells/mL. Freshly prepared standard cell suspensions were immediately used for biofilm formation. All cultures were performed at aerobic conditions.
Biofilm formation was carried out in polystyrene, flat-bottomed, 24 well microtiter plate (Greiner Bio-One, Singapore) as previously described [ ]. In brief, titanium discs were placed inside each well and immersed in 1 ml of the OD adjusted cell suspensions. The biofilms were grown at 37 °C in an orbital shaker incubator at 80 rpm for 1–7 days for downstream evaluation. The media was changed every 24 h by carefully pipetting it out to remove non-adherent cells without disrupting the biofilm formed. Afterward, 1 ml of fresh media was added gently to the side wall of the well.
2.3
XTT reduction assay
XTT assay was performed to appraise the metabolic activity of C. albicans as described previously [ ]. At each time point, the biofilms were gently washed with phosphate buffer solution (PBS, Invitrogen, USA) applied on the sidewall of the well in order to remove the non-adherent cells and the discs were immediately transferred to new wells. Subsequently, 1 ml of XTT-menadione solution was added into each well and incubated at 37 °C in dark for 20 min. Afterward, 200 μl of solution was transferred to a 96 well plate and the absorbance was measured at 490 nm (Multiskan GO, Thermo Scientific, USA).
2.4
Colony forming unit assay (CFU)
Firstly, non-adherent cells were gently removed with PBS. Thereafter, the discs were immersed in PBS (10 ml) and the attached biofilms were dissociated from the discs by vigorous agitation for 3 min (Vortex Genie 2, speed 8, Labgene Scientific, Switzerland). The detachment of biofilms was confirmed by crystal violet assay (data not shown). A dilution series was prepared in PBS for the cell suspensions obtained from the respective biofilms. A volume of 100 μl from each dilution was plated out in triplicates on GMM agar for enumeration. Further, the GMM agar plates were incubated at 30 °C for 24 h and the fungal colonies were counted.
2.5
Confocal laser scanning microscopy (CLSM) and scanning electron microscopy (SEM)
C. albicans biofilms grown on Control, TiGD and TiGV were stained with calcofluor white (excitation/emission 365/435 nm) and imaged using Olympus FluoView FV1000 (Olympus, Japan) and TissueFAXS Slide Scanner (TissueGnostics, Austria). The mean cell density was calculated using Image J software (NIH, USA).
For SEM, the biofilms were fixed with 2% gluteraldehyde in PBS for 4 h in room temperature and dehydrated in an ascending ethanol gradient (5 min at each concentration). Thereafter, the samples were subjected to critical point drying with liquid CO 2 , sputter coated with gold (EM ACE600 Coater, Leica, Germany) and imaged using an Olympus FEI 650 Scanning Electron Microscope (Japan).
2.6
Statistical analysis
Control group was uncoated titanium. All groups had three biological samples and the experiments were performed in independent triplicates. Shapiro-Wilk and Lavene’s tests were performed for checking normality and homogeneity. One- or two-way ANOVA tests were used to analyze the data pertaining the R a and microbial assays, respectively followed by Tukey’s HSD with pre-set significance level of 5%.
3
Results
3.1
Sample characterization
The surfaces of TiGD and TiGV samples presented the folds and bundles typical of graphene films ( Fig. 1 A). Raman mappings revealed a coverage yield of 99% for both the TiGD and TiGV samples ( Fig. 1 B and Table 1 ). The arithmetic average of the roughness profile (R a ) of Control was significantly higher than TiGD and TiGV ( p = 0.007 and 0.005, respectively). The I 2D /I G ratio confirms the presence of more than one layer of graphene on both samples whereas the I D /I G ratio shows the existence of few defects for both graphene nanocoated groups ( Table 1 ).
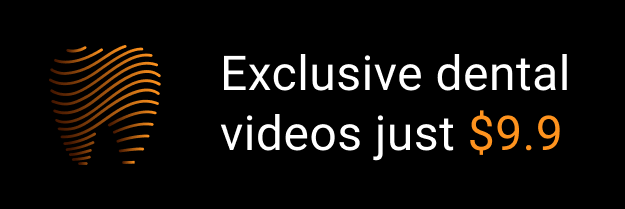