* The authors would like to acknowledge the contributions of David Witherspoon for his involvement with previous editions.
Although nonsurgical endodontic treatment is a highly predictable option in most cases, surgery may be indicated for teeth with persistent periradicular pathoses that have not responded to nonsurgical approaches. Surgical root canal therapy, including root-end resection, has been practiced since at least the mid-1800s. In 1906, Schamberg described using radiographs to assist diagnosis and the use of surgical burs to perform a rapid osteotomy and root-end “ablation.”
Perhaps the single most important development in dental practice in the early 20th century was the introduction of safe, effective local anesthesia, which allowed for more meticulous and comfortable surgical treatment. Formal recognition of endodontics as a specialty in 1963 ushered in a new era of basic and clinical research focused on the prevention and treatment of pulpal and periradicular pathosis.
Since the 1990s, periradicular surgery has continued to evolve into a precise, biologically based adjunct to nonsurgical root canal therapy. The parallel development of new instruments and materials, along with a better understanding of the biology of wound healing, has made surgical treatment a viable alternative to extraction and tooth replacement, rather than a treatment of last resort.
Periradicular surgery, when indicated, should be considered an extension of nonsurgical treatment, because the underlying etiology of the disease process and the objectives of treatment are the same: prevention or elimination of apical periodontitis. Surgical root canal treatment should not be considered as somehow separate from nonsurgical treatment, although the instruments and techniques are obviously quite different. Surgical treatment accounts for about 3% to 10% of the typical endodontic specialty practice. A web-based survey found that 91% of active endodontists perform some type of root-end surgery, and almost all are using a dental operating microscope and ultrasonics. Endodontists perform almost 78% of surgical root canal treatments; general dentists and other specialists perform 15.5% and 6.6%, respectively. Although appropriately trained general dentists and other dental specialists may perform periradicular surgery, individuals with advanced training in endodontics have developed most of the current periradicular surgery techniques and science presented in this chapter. We believe that endodontists must continue to include periradicular surgery as a routine part of clinical endodontic practice and not abrogate this treatment option to others, who may not possess the same background, skills, or values. When patient preferences and quality of life measures are considered, patients can be expected to place high value on endodontic treatment and the retention of a natural tooth.
Indications for Periradicular Surgery
Etiology of Persistent Periradicular Disease
The first and arguably most important step in treatment decision making is attempting to determine the cause of persistent periradicular disease. Treatment then is directed at eliminating the etiology, which most often is the presence of bacteria and other microbial irritants in the root canal space. Nonsurgical retreatment, when possible, often is the first choice for attempting to correct obvious deficiencies in the previous treatment (see Chapter 8 ). However, microorganisms can survive even in apparently well-treated teeth in dentinal tubules, canal irregularities, deltas, and isthmus areas. If residual microorganisms remain completely entombed in the root canal system, periradicular healing should occur. Sealing off all potential routes of microbial escape from the root canal system is the goal of both nonsurgical and surgical treatment. When microorganisms of sufficient pathogenicity and number gain access to the periradicular tissues, pathosis develops.
Enterococcus faecalis is commonly isolated from failing root canal–treated teeth and is known to be especially difficult to eliminate with standard instrumentation and irrigation techniques. Unlike primary endodontic infections, which predominantly are associated with mixed anaerobic microbiota, it has been commonly accepted that treatment failures are more frequently associated with one or two microorganisms. However, research using more sophisticated techniques (16S ribosomal RNA gene clone library analysis) has determined that multiple, previously uncultivated phylotypes can be identified in the majority of teeth with persistent apical periodontitis. Fungi and viruses have also emerged as potential causes of root canal failure and may play either a primary or secondary role in persistent periradicular pathosis.
Established extraradicular colonies of microorganisms also may be a reason for failure of some teeth to respond to nonsurgical treatment. When microorganisms are able to arrange in an extraradicular biofilm, they may be particularly resistant to elimination by host defense mechanisms and antimicrobial agents. Persistent extraradicular root surface colonization cannot be diagnosed by noninvasive methods but may be suspected in well-treated cases that are refractory to nonsurgical treatment. Although the presence of extraradicular microbial colonies has been somewhat controversial, studies using DNA-DNA hybridization techniques have confirmed the persistence of microorganisms in the periradicular tissues of some root canal–treated teeth.
Overextended filling materials may contribute to treatment failure, presumably as a result of a chronic inflammatory response. Although this is possible and even likely with certain toxic materials (e.g., pastes containing formaldehyde ), the role of relatively inert materials such as gutta-percha and set sealer is less clear, and these materials probably become a significant contributing factor only if microorganisms are present. If the root apex is close to the buccal cortical plate, apical fenestration may occur, leading to persistent symptoms, especially tenderness to palpation over the root apex. Some have suggested that overextension of filling materials may contribute to endodontic failure because certain dental materials may induce periodontal ligament (PDL) cell apoptosis. This specific interaction between filling materials and periradicular tissues is not fully understood and deserves further research. The poorer prognosis often reported with overextended root canal fillings also may be related simply to lack of an adequate apical seal and subsequent egress of microorganisms from the root canal space. Regardless, minor overextension of filling material is rarely a sole indication for surgery except when symptoms or periradicular pathosis develops. Significant overextension of filling material, especially when important anatomic areas and possibly toxic materials are involved, is an indication for referral to an endodontist or oral surgeon for evaluation and, possibly, treatment.
The presence of periradicular cholesterol crystals may interfere with healing after nonsurgical root canal treatment. Although relatively uncommon, true periradicular cysts (completely enclosed, epithelium-lined cavities) may not be expected to resolve after nonsurgical treatment. As with other extraradicular causes of failure, surgery is indicated, because definitive diagnosis and treatment require an excisional biopsy and removal of the periradicular tissue.
Vertical root fractures are a significant cause of failure and may be difficult to diagnose in the early stages. Exploratory surgery often is required to confirm a root fracture. Cone-beam computed tomography (CBCT) is a promising tool for noninvasive diagnosis of root fractures and is discussed in greater detail later in this chapter and in Chapter 2 . Although several promising approaches to the management of vertical root fractures have been proposed, the prognosis generally is believed to be poor. Extraction usually is the treatment of choice, especially if appropriate tooth replacement options are available (see Chapter 21 ). Root amputation or hemisection may be considered in a multirooted tooth if the remaining tooth structure is uninvolved and sufficient periodontal support is present.
The relationship between systemic disease and periradicular healing is not completely understood. The possible influence of certain systemic medications on wound healing is discussed later in the chapter. Compromised host healing capacity may be a contributing factor in delayed healing and failure of some root canal–treated teeth. For example, complete healing after nonsurgical root canal treatment is less likely in diabetic patients with preoperative periradicular pathosis. Patients undergoing immunosuppressive therapy may be at greater risk for delayed healing, treatment failure, or acute exacerbation of a subclinical infection, although two studies involving subgroups of immunocompromised patients (bone marrow transplant and acquired immunodeficiency syndrome [AIDS]) did not find these patients to be at higher risk for complications related to endodontic treatment.
Rationale for Surgical Treatment
Although nonsurgical retreatment generally is believed to be the preferred first approach in the management of persistent apical periodontitis, periradicular surgery is indicated when nonsurgical retreatment is impractical or unlikely to improve on the previous result (see Chapter 8 ). In particular, a surgical approach may be the first choice for managing teeth with long posts or irretrievable separated instruments, non-negotiable ledges and canal blockages or transportation, hard cement filling materials, failure of previous nonsurgical retreatment, and suspected vertical root fracture, or when a biopsy is indicated ( Figs. 9-1 to 9-4 ). Even when surgical treatment is the likely definitive approach, nonsurgical therapy before the procedure may be recommended to help reduce the number of microorganisms in the root canal system and ensure a more favorable long-term prognosis. On the other hand, surgery may be the first choice even if a tooth can be treated nonsurgically if the risks and costs of retreatment are considered excessive. For example, disassembling a recently restored bridge abutment tooth to allow endodontic retreatment may be technically possible but not economically feasible. One study found that endodontic microsurgery may be the most cost-effective option for management of persistent periapical disease, compared to nonsurgical retreatment, extraction and placement of a fixed partial denture, and extraction and placement of a single tooth implant. Case-specific variables and clinical judgment are key elements in the decision-making process, as current evidence supports the contention that the prognosis for surgical treatment is approximately the same as that for nonsurgical retreatment.




Clinical Decision Making
Clinical decision making is a process that combines the best available evidence, clinical judgment, and patient preferences. Treatment choices are always made under conditions of at least some uncertainty. Surgical root canal treatment rarely is the only possible choice. Clinicians and patients must weigh the relative benefits, risks, and costs of two or more acceptable alternatives. Patients and clinicians can be expected to hold different attitudes about the value of potential treatment outcomes. In a prognosis study of single-tooth dental implants, Gibbard and Zarb reported that factors considered significant by health care professionals may not be important to patients. Even among groups of dentists and dental specialists, the threshold for treatment varies widely and treatment recommendations may depend more on personal values and experience than objective analysis of treatment costs, prognosis, risks, and alternatives.
Shared decision making, in which the patient and clinician consider outcome probabilities and patient preferences and agree on the appropriate treatment, is preferable to a clinician-directed treatment decision. Such a two-way exchange allows the clinician to provide the best available current evidence and case-specific clinical judgment while encouraging a decision that considers the patient’s personal values and preferences. This decision-making model has been shown to increase patient knowledge about and satisfaction with the treatment choice. In general, most patients prefer to be actively involved in the decision-making process but want to leave the specifics of the treatment to the clinician. That is, the risks, benefits, and costs of treatment alternatives are important to patients, but the details of the procedure usually are not. Shared decision making is particularly relevant in light of the current trend in some areas to recommend implants as an alternative to root canal treatment.
Comparative probabilities for a successful outcome after surgical root canal treatment, nonsurgical retreatment, or extraction and replacement with a fixed prosthesis or implant are difficult to project. Many treatment variables are complex and not easily quantified, such as the affected location in the mouth, bone quality, practitioner’s skill, possible influence of systemic disease on healing, periodontal support, bulk of the remaining tooth structure and resistance to fracture, quality of the coronal restoration, patient susceptibility to recurrent caries, materials used, and other factors. In addition, the definition of success varies considerably and is inconsistent from study to study.
Boioli and colleagues performed a meta-analysis of implant studies and reported a 93% survival rate at 5 years. The 5- to 10-year success rate for implant retention routinely is reported to be 90% to 97%, depending on the location in the mouth and other variables. Surgical root canal treatment was previously reported to have a lower success rate than implant placement. However, most of these older studies would be considered weak by today’s evidence-based standards and, more important, do not reflect the use of many of the newer surgical materials and techniques. With careful case selection, surgical skill, and the use of materials and techniques described later in this chapter, many studies have demonstrated success rates over 90% for surgical root canal treatment. von Arx reported that the 5-year success for teeth receiving endodontic microsurgery was 8% less than observed at 1 year. A similar study found an 85% success rate after 10 years. A systematic review and meta-analysis comparing traditional root-end surgery to modern endodontic microsurgery (ultrasonic root-end preparation; root-end filling with IRM, Super EBA, or MTA; and high power [> ×10] magnification and illumination) found that the outcome for endodontic microsurgery using current materials and techniques was 94%, compared to 59% success with traditional root-end surgery.
In broad terms and under ideal conditions, the prognoses for nonsurgical retreatment, surgical treatment, and implant placement should be roughly equal. The choice of treatment should be based on the best available evidence, case-specific clinical judgment, and the patient’s preferences. As a stronger evidence base continues to develop, we predict that algorithms encompassing multiple patient and treatment variables will be devised to assist clinical decision making.
General Biologic Principles of Wound Healing
Wound healing varies from region to region in the body and depends on several factors, including the type of tissue, the type of wound, and the type of healing. In periradicular surgery, the tissues include free and attached gingiva, the alveolar mucosa, periosteum, bone, the periodontal ligament, and cementum. The wound may be intentional surgical trauma, which includes incision, blunt dissection, and excision (surgical), or pathologic or traumatic wounds. Healing occurs by primary or secondary intention.
An incisional blunt dissectional wound, for example, can be considered to heal typically by primary intention, whereas a dissectional wound involving the resected root surface and osseous crypt heals by secondary intention. An important concept of the wound healing process in general is the difference between regeneration and repair. The goal of all surgical procedures should be regeneration, which returns the tissues to their normal microarchitecture and function, rather than repair, a healing outcome in which tissues do not return to normal architecture and function. Repair typically results in the formation of scar tissue.
The wounding process varies, depending on the types of tissue and injury. However, all wounds progress through three broad, overlapping phases in the process of healing; these are the inflammatory phase, the proliferative phase, and the maturation phase. Although these phases can be identified in healing tissue, none of them has a clear beginning or end. Furthermore, in a wound such as an endodontic surgical site, which involves more than one tissue type, these phases progress at different rates in each type of tissue.
Soft-Tissue Wound Healing
Inflammatory Phase
Broadly speaking, the inflammatory phase of healing is similar for all tissues. This phase can be broken down further into clot formation, early inflammation, and late inflammation.
Clot Formation
Clot formation begins with three events: (1) blood vessel contraction is initiated by platelet degranulation of serotonin, which acts on the endothelial cell and increases the permeability of the vessel, allowing a protein-rich exudate to enter the wound site; (2) a plug composed of platelets forms, primarily through intravascular platelet aggregation; (3) both the extrinsic and intrinsic clotting mechanisms are activated. Several other events occur simultaneously, including activation of the kinin, complement, and fibrinolytic systems and the generation of plasmin. These events stabilize hemostasis, begin the production of a number of mitogens and chemoattractants, and initiate the process of wound decontamination. The result is a coagulum consisting of widely spaced, haphazardly arranged fibrin strands with serum exudate, erythrocytes, tissue debris, and inflammatory cells. Compression of the surgical flap with sterile iced gauze immediately after surgery is designed to minimize the thickness of the fibrin clot and thereby accelerate optimal wound healing.
Early Inflammation: Polymorphonuclear Neutrophil (PMN) Organization
As a result of production of chemoattractants by the various components of the clot, polymorphonuclear neutrophils (PMNs) begin to enter the wound site within 6 hours of clot stabilization. The number of PMNs increases steadily, peaking at about 24 to 48 hours after the injury. Three key steps mark PMN migration into the wound site: (1) pavementing, in which the red blood cells undertake intravascular agglutination, allowing the PMNs to adhere to the endothelial cells; (2) emigration, in which the PMNs actively pass through the vascular wall; and (3) migration, in which the PMNs use ameboid motion, under the influence of the various chemotactic mediators, to move into the injured tissues.
The principal role of the PMNs is wound decontamination by means of phagocytosis of bacteria. The high number of PMNs in the wound site is relatively short lived; the number drops rapidly after the third day.
Late Inflammation: Macrophage Organization
About the time the PMN population is declining (48 to 96 hours after injury), macrophages begin to enter the wound site. They reach a peak concentration by approximately the third or fourth day. These cells, which are derived from circulating monocytes, leave the bloodstream under the influence of the chemoattractants in the wound site. The monocytes subsequently evolve into macrophages. Macrophages have a much longer life span than PMNs; they remain in the wound until healing is complete. Similar to PMNs, macrophages play a major role in wound decontamination through phagocytosis and digestion of microorganisms and tissue debris.
Macrophages are considerably more bioactive than PMNs and can secrete a vast array of cytokines. A key action of many of these bioactive substances is initiation of the proliferative phase of wound healing, which is accomplished by prompting the formation of granulation tissue. Two other major functions of macrophages are ingestion and processing of antigens for presentation to T lymphocytes, which enter the wound after the macrophages. Unlike PMNs, macrophages play an essential role in the regulation of wound healing. A reduction in the number of macrophages in the wound site delays healing, because the wound does not progress to the next phase. For example, the age-related reduction in healing potential appears to be partly the result of loss of estrogen regulation of macrophages in healing tissues.
Proliferative Phase
The proliferative phase is characterized by the formation of granulation tissue in the wound. Two key cell types, fibroblasts and endothelial cells, have a primary role in the formation of granulation tissue. Granulation tissue is a fragile structure composed of an extracellular matrix of fibrin, fibronectin, glycosaminoglycans, proliferating endothelial cells, new capillaries, and fibroblasts mixed with inflammatory macrophages and lymphocytes. Epithelial cells also are active during this phase of soft-tissue healing and are responsible for initial wound closure. Guided tissue regeneration (GTR) procedures are based on control of the epithelial cell growth rate during this phase.
Fibroblasts: Fibroplasia
Undifferentiated mesenchymal stem cells in the perivascular tissue and fibroblasts in the adjacent connective tissue migrate into the wound site on the third day after injury and achieve their peak numbers by approximately the seventh day. This action is stimulated by a combination of cytokines (e.g., fibroblast growth factor [FGF], insulin-like growth factor–1 [IGF-1], and 2-15 platelet-derived growth factor [PDGF]), which are produced initially by platelets and subsequently by macrophages and lymphocytes. As the number of macrophages declines and the fibroblast population increases, the tissue in the wound transforms from a granulomatous tissue to a granulation tissue.
Fibroblasts are the crucial reconstructive cell in the progression of wound healing, because they produce most of the structural proteins forming the extracellular matrix (e.g., collagen). Collagen is first detected in the wound about the third day after injury. The fibroblasts produce type III collagen initially and then, as the wound matures, type I collagen. As this network of collagen fibers is laid down, endothelial and smooth muscle cells begin to migrate into the wound. Subsequently, as wound healing progresses, the collagen fibers become organized by cross-linking. Regularly aligned bundles of collagen begin to orient so as to resist stress in the healing wound.
A focused type of fibroblast, known as a myofibroblast, plays a significant role in wound contraction, particularly in incisional-type wounds. Myofibroblasts align themselves parallel with the wound surface and then contract, drawing the wound edges together. These cells are eliminated by apoptosis after wound closure.
Endothelial Cells: Angiogenesis
Capillary buds originate from the vessels at the periphery of the wound and extend into the wound proper. This occurs concurrently with fibroblast proliferation and can begin as early as 48 to 72 hours after injury. Without angiogenesis, the wound would not have the blood supply needed for further active healing. The capillary sprouts eventually join to form a network of capillary loops (capillary plexuses) throughout the wound.
In addition to a low oxygen concentration in the wound proper, several factors have been identified as potent stimulators of angiogenesis, including vascular endothelial growth factor (VEGF), basic fibroblast growth factor (bFGF), acidic FGF (aFGF), transforming growth factor–alpha (TGF-alpha) and transforming growth factor–beta (TGF-beta), epidermal growth factor (EGF), interleukin-1 (IL-1), and tumor necrosis factor–alpha (TNF-alpha), as well as lactic acid. All of these have been shown to stimulate new vessel development.
Epithelium
The first step in epithelial healing is the formation of an epithelial seal on the surface of the fibrin clot. This process begins at the edge of the wound, where the basal and suprabasal prickle cells rapidly undergo mitosis. The cells then migrate across the fibrin clot at a remarkable rate (0.5 to 1 mm per day). This monolayer of epithelial cells continues to migrate by contact guidance along the fibrin scaffold of the clot below. Migration stops as a result of contact inhibition of the epithelial cells from the opposing wound edge. Once the epithelium from both sides of the wound is in contact, an epithelial seal is achieved. In wounds healing by primary intention, formation of an epithelial seal typically takes 21 to 28 hours after reapproximation of the wound margins.
Maturation Phase
Under ideal conditions, maturation of the wound begins 5 to 7 days after injury. A reduction in fibroblasts, vascular channels, and extracellular fluids marks the transition to this phase of healing. During the early stages of wound maturation, the wound matrix is chiefly composed of fibronectin and hyaluronic acid. As the tensile strength of the wound increases, significant upregulation of collagen fibrogenesis occurs. Collagen remodeling ensues, with the formation of larger collagen bundles and alteration of intermolecular cross-linking. The result is a conversion of granulation tissue to fibrous connective tissue and a decreased parallelism of collagen to the plane of the wound. Aggregated collagen fibril bundles increase the tensile strength of the wound. As healing progresses in the wound, the collagen gradually reorganizes; this requires degradation and reaggregation of the collagen. The degradation of collagen is controlled by a variety of collagenase enzymes. Remodeling results in a gradual reduction in the cellularity and vascularity of the reparative tissue; the degree to which this occurs determines the extent of scar tissue formation. Active remodeling of scar tissue can continue very slowly for life.
Maturation of the epithelial layer quickly follows formation of the epithelial seal. The monolayer of cells forming the epithelial seal differentiates and undergoes mitosis and maturation to form a definitive layer of stratified squamous epithelium. In this way an epithelial barrier is formed that protects the underlying wound from further invasion by oral microbes. The epithelial barrier typically forms by 36 to 42 hours after suturing of the wound and is characterized by a significant increase in wound strength.
Hard-Tissue Healing: Excisional Dentoalveolar Wound
The inflammatory and proliferative phases of healing for hard tissue are similar to those for soft tissue. A clot forms in the bony crypt, and an inflammatory process ensues that involves PMNs initially and macrophages subsequently. This is followed by the formation of granulation tissue with an angiogenic component. However, the maturation phase of hard-tissue healing differs markedly from that for soft tissues, primarily because of the tissues involved: cortical bone, cancellous bone, alveolar bone proper, endosteum, periodontal ligament, cementum, dentin, and inner mucoperiosteal tissue.
Osteoblasts: Osteogenesis
The healing of an excisional osseous wound that is approximately 1 cm in diameter is similar to that of a fractured long bone. It progresses from hematoma to inflammation, eradication of nonvital debris, proliferation of granulation tissue, callus formation, conversion of woven bone to lamellar bone and, finally, remodeling of the united bone ends. The coagulum that initially forms delays healing and must be removed to allow wound healing to progress.
A major difference between soft- and hard-tissue wound healing is found in the role of the osteoclast. Functionally, osteoclasts act as organizational units to debride necrotic bone from the wound margin, much as macrophages remove tissue debris from the clot. Granulation tissue begins to proliferate from the severed periodontal ligament by 2 to 4 days after root-end resection. This tissue rapidly encapsulates the root end. Simultaneously, endosteal proliferation into the coagulum occurs from the deep surface of the bony wound edge. The coagulum in the bony crypt is quickly converted into a mass of granulation tissue. In addition to those already discussed, several cell types migrate into the coagulum, including osteoprogenitor cells, preosteoblasts, and osteoblasts. These cells begin the formation of woven bone within the mass of granulation tissue. New bone formation is apparent about 6 days after surgery.
Bone formation can be categorized into two types, each having several phases. The phases differ, depending on which type of formation is involved. One type of bone formation is a matrix vesicle–based process, and the other type is based on osteoid secretion. In both processes, osteoblasts produce the bone matrix. They secrete a collagen-rich ground substance that is essential for mineralization. Osteoblasts also cause calcium and phosphorus to precipitate from the blood.
In the formation of woven bone, which occurs by the matrix vesicle–based process, osteoblasts produce matrix vesicles through exocytosis (the release of substances contained in a vesicle within a cell by a process in which the membrane surrounding the vesicle unites with the membrane forming the outer wall of the cell) of their plasma membranes. As hydroxyapatite crystals accrue in the vesicles, they become enlarged and eventually rupture. This process begins with the deposition and growth of hydroxyapatite crystals in the pore regions. The crystals then amalgamate to form structures known as spherulites. Union of the separate spherulites results in mineralization.
The formation of lamellar bone does not require the production of matrix vesicles; rather, it proceeds by the osteoblast secretion process. Osteoblasts secrete an organic matrix composed of longitudinally arranged collagen matrix fibrils (mainly type I collagen). Mineralization occurs by mineral deposition directly along the collagen fibrils. This stage has been associated with a rise in pH, most likely because of the enzyme alkaline phosphatase, which is secreted by osteoblasts and other cells and plays an important role in mineralization. The exact role of alkaline phosphatase during mineralization is unclear. The balance of evidence favors a positive catalytic role. Some hypothesize that alkaline phosphatase promotes mineralization through a combination of mechanisms involving various cells, extracellular matrix proteins, and elements. The interaction of alkaline phosphatase and phosphoproteins in both bone and dentin appears to be especially critical to the mineralization process.
Inhibitor molecules, such as pyrophosphate and acidic noncollagenous bone proteins, regulate mineralization. Several growth factors also have been identified as key components in the production of osseous tissue. These include TGF-beta, bone morphogenetic protein (BMP), PDGF, FGF, and IGF. One clinical study demonstrated that the addition of autologous platelet concentrate to the surgical site decreased postsurgical pain and may accelerate the healing process.
Three to 4 weeks after surgery, an excisional osseous wound is 75% to 80% filled with trabeculae surrounded by intensely active osteoid and osteoblastic cells. A reforming periosteum can be seen on the outer surface of the wound; it is highly cellular and has more fibrous connective tissue oriented parallel to the plane of the former cortical plate. At 8 weeks after surgery, the trabeculae are larger and denser and the osteoblasts are less active; these cells occupy about 80% of the original wound. Also, fewer osteoid cells are associated with the maturing trabeculae. The overlying periosteum has reformed and is in contact with the newly developed bone. The osseous defect typically is filled with bone tissue by 16 weeks after surgery. However, the cortical plate has not yet totally reformed. Maturation and remodeling of the osseous tissue continues for several more months.
Local healing is also influenced systemically by the endocrine system and its three general categories of hormones: polypeptide regulators (parathyroid hormone, calcitonin, insulin, and growth hormone), steroid hormones (vitamin D 3 , glucocorticoids, and the sex hormones), and thyroid hormones.
Cementoblasts: Cementogenesis
During regeneration of the periradicular tissues, cementum forms over the surface of surgically resected root ends. The exact spatial and temporal sequences of events leading to this formation of new cementum remains undefined; however, cementogenesis is important, because cementum is relatively resistant to resorption (osteoclasts have little affinity for attaching to cementum).
Cementogenesis begins 10 to 12 days after root-end resection. Cementoblasts develop at the root periphery and proceed centrally toward the root canal. Considerable evidence indicates that the cells that regulate cementogenesis are derived from ectomesenchymal cells in the tooth germ proper rather than from bone or other surrounding tissues. The migration and attachment of precementoblasts to the root surface dentin is monitored by mediators from within the dentin itself. Cementum covers the resected root end in approximately 28 days. Newly formed PDL fibers show a functional realignment that involves reorientation of fibers perpendicular to the plane of the resected root end, extending from the newly formed cementum to the woven bone trabeculae. This occurs about 8 weeks after surgery.
Systemic Medications and Wound Healing
Bisphosphonates
Bisphosphonates are commonly used for the treatment of osteopenia, osteoporosis, Paget’s disease of bone, multiple myeloma, and metastatic bone, breast, and prostate cancer. The potential association between bisphosphonate use and osteonecrosis of the jaw was first reported in 2003. The current preferred term for this condition is antiresorptive agent-induced osteonecrosis of the jaw (ARONJ) or medication-related osteonecrosis of the jaw (MRONJ). The change in terminology reflects the finding that, in addition to bisphosphonates, other antiresorptive agents can increase the risk for osteonecrosis of the jaw (e.g., denosumab). ARONJ can occur spontaneously but is more commonly associated with dental procedures that involve bone trauma. Patient variables that increase the risk for ARONJ include age (over 65 years old), chronic use of corticosteroids, use of bisphosphonates for more than 2 years, smoking, diabetes, and obesity. It is important to note that the risk for ARONJ with commonly prescribed oral bisphosphonates appears to be very low, whereas the overall benefits of this drug class for reducing morbidity and mortality related to hip, vertebral, and other bone fractures are significant. The estimated incidence for ARONJ in patients taking oral bisphosphonates ranges from zero to 1 in 2260 cases, although dental extractions may quadruple the risk of developing ARONJ. A reasonable upper estimate of the risk of developing ARONJ in a patient who does not have cancer is about 0.1%. One clinical study reported a much higher 4% risk for developing ARONJ in patients taking a specific oral bisphosphonate (alendronate sodium), although this was a retrospective study using an electronic patient record at a dental school and may not have adequately controlled for other relevant variables. The majority of ARONJ cases have been reported in patients taking IV bisphosphonates (e.g., zoledronic acid and pamidronate). According to one report, approximately 20% of patients taking IV bisphosphonates may develop ARONJ. A systematic review found that there was a strong association between cancer and risk of ARONJ, with the prevalence ranging from 0.7% to 13.3%. Interestingly, this same review found that the higher-quality studies reported the highest prevalence of ARONJ in cancer patients.
Conservative and timely management of apical periodontitis is essential to reduce the risk of ARONJ in patients taking bisphosphonates. Because periapical pathosis may exacerbate or increase the risk for ARONJ, the “no treatment” option is not a viable choice. The potential risk for ARONJ should be thoroughly discussed with all patients that are taking bisphosphonates, as well as treatment options. Nonsurgical retreatment should usually be considered the first choice, especially for patients with a history of IV bisphosphonate use or other risk factors. Even so, surgical treatment may be indicated to manage chronic or acute apical periodontitis. When the only viable treatment options for the management of persistent periradicular inflammation are surgical root canal therapy or extraction, the question of which option is more or less likely to lead to ARONJ in at-risk patients remains unanswered. In general, the procedure that could most predictably eliminate the periradicular inflammation with the least amount of surgical trauma would be preferred. Conservative surgical technique, primary tissue closure, and use of chlorhexidine mouth rinses preoperatively and during the healing stage are recommended. There is some limited evidence to support the use of prophylactic antibiotics and chlorhexidine mouth rinse to decrease the risk of ARONJ for patients undergoing surgical dental treatment. Because bisphosphonates have a substantial half-life when incorporated into bone, discontinuation of bisphosphonates prior to dental treatment is unlikely to provide any measurable benefit and may place the patient at greater risk for complications that the drug is intended to prevent.
The possibility of predicting which patients may be at greater risk for ARONJ based on serum levels of C-terminal cross-linking telopeptide of type I collagen (CTX) has been suggested based on clinical observations. This observation may provide a useful clue for future risk assessment but requires additional refinement and validation prior to widespread acceptance. In fact, one study found that although serum CTX levels were lower in patients taking bisphosphonates (as expected), there was no association between lower CTX levels and increased risk of ARONJ. This same study found that patients taking a once-a-year dose of 5 mg zoledronic acid were at no greater risk for ARONJ than a control group (1 case in 5903 patients). Once ARONJ develops, treatment options are limited. A possible treatment strategy proposed in a series of case reports is the use of teriparatide, an anabolic drug that stimulates osteoblast formation and is used for the management of osteoporosis (see also Chapter 3 ).
Glucocorticoids
Glucocorticoid therapy has been shown to induce rapid bone loss within the first 3 months of treatment. Even inhaled steroids have been implicated as a cause of bone loss. Bone formation is inhibited partly through a decrease in osteoblast life span and function, a reduction in the mineral apposition rate, and a prolonged mineralization lag time. Biochemical markers of bone formation (i.e., osteocalcin and bone-specific alkaline phosphatase) are suppressed. In addition to this primary suppressive effect, glucocorticoids cause accelerated bone resorption. The number and activity of osteoclasts increase during early glucocorticoid exposure. With continued use of glucocorticoids, the rapid rate of osteoclast-mediated bone resorption slows, but suppression of bone formation continues as the overriding skeletal activity. Bone loss therefore is progressive, because bone resorption chronically exceeds bone formation.
The adverse effects of glucocorticoids on bone are mediated by sex steroid deficiency and expression of locally produced growth factors and related proteins, such as reduced production of IGF-1 and alterations in IGF-binding proteins in osteoblasts. The direct effect on calcium metabolism through alteration of vitamin D metabolism leads to a state of secondary hyperparathyroidism.
Nonsteroidal Anti-inflammatory Drugs (NSAIDs)
Bone homeostasis is regulated by many factors, including prostaglandins (PGs). PGs are important to both normal and pathologic bone turnover. PGs modulate osteoblast proliferation and differentiated functions. The levels of prostaglandins E (PGE) and F (PGF) are elevated in the early phase of fracture healing, and administration of PGE 2 has increased the rate of osseous repair in several animal studies. NSAID inhibition of the enzyme cyclooxygenase (COX), which is involved in the synthesis of PGs, is the same mechanism by which NSAIDs control pain. By inhibiting the COX enzymes and the subsequent production of prostaglandins, NSAIDs accomplish the desired anti-inflammatory effects—but also prevent the increased production of PGs required for bone healing. In vitro studies using animal models have shown that NSAIDs inhibit osteoblast proliferation and stimulate protein synthesis. These drugs also have been shown to delay fracture healing and to affect bone formation adversely in animals and humans. In one study, the use of NSAIDs reduced the amount of bony ingrowth into an orthopedic implant.
In other studies, NSAIDs reduced bone resorption associated with experimentally induced periapical lesions, and systemic NSAIDs may play a positive role in maintaining bone height around endosseous titanium dental implants. Additional research is needed to clarify the specific influence of NSAIDs on healing after periradicular surgery.
Cyclooxygenase-2 (COX-2) Inhibitors
Although both COX-1 and COX-2 have been identified in osteoblasts, the different roles of the two cyclooxygenases in bone formation remain unclear. A study using COX-1 and COX-2 knockout mice compared the roles of the two enzymes in fracture healing. COX-2 was shown to have an essential role in both endochondral and intramembranous bone formation during wound healing. COX-2 knockout mice showed a persistent delay in cartilage tissue ossification. No difference in fracture healing was observed between the COX-1 knockout mice and the wild-type control used in this study. Additional studies have shown a persistent delay in healing with COX-2 NSAIDs ; however, this difference in effect was not apparent clinically in a study examining the healing of spinal fusion wounds.
Preoperative Evaluation of Medically Complex Patients
The preoperative assessment must take into account both the type of procedure planned and the type of patient (i.e., physical health and psychological status). Healthy patients clearly can be expected to tolerate surgical procedures better than medically complex patients (see Chapters 3 and 26 ). Clinicians should anticipate and prepare for the inevitability of treating more medically complex patients as the population ages. It is beyond the scope of this brief section to cover all possible medical considerations; rather, the most common issues that may require modification of an endodontic surgical treatment plan are presented. Relatively few absolute contraindications to periradicular surgery exist for patients well enough to seek care in an ambulatory dental office. Nonetheless, if any question arises about a patient’s ability to tolerate a surgical procedure, medical consultation is advised. A thorough medical history and assessment of vital signs are required parts of the presurgical evaluation.
The American Society of Anesthesiologists (ASA) has developed a widely used system for establishing surgical risk. Patients categorized as ASA 1 are healthy and usually require no modification of the surgical treatment plan. Patients classified as ASA 4 or ASA 5 are not treated in a dental office; these individuals have significant medical problems that take priority over dental considerations. Patients considered ASA 2 or ASA 3 are commonly seen on an outpatient basis and may require medical consultation and modification of the surgical treatment plan. Patients in the ASA 2 and ASA 3 categories have mild to moderate systemic disease and often are undergoing treatment with one or more prescription medications. The ASA classification system should only be used as a general guide because, when used alone, the ASA system is not a reliable predictor of operative risk. In addition, even experienced anesthesiologists exhibit differences of opinion when using the ASA system to classify patients. The patient’s psychological status and anticipated procedural stress should be considered in addition to the ASA classification of physical health. A stress reduction protocol may be helpful for patients who report moderate dental anxiety with concurrent mild to moderate systemic disease (see Chapter 28 ).
Surgical procedures typically require a larger amount of local anesthetic with a vasoconstrictor than nonsurgical root canal treatment. An essential part of the presurgical evaluation is an assessment of the patient’s cardiovascular status and tolerance for local anesthetics that contain epinephrine. In particular, patients with advanced cardiovascular disease, geriatric patients, and patients taking certain medications may have a reduced tolerance for local anesthetics containing a vasoconstrictor (see Chapters 3 and 4 ). Treatment-induced stress associated with surgical procedures can cause a significant increase in heart rate and systolic blood pressure when compared to nonsurgical root canal therapy. The use of local anesthetics with vasoconstrictors was addressed in the seventh report of the Joint National Committee on Prevention, Detection, Evaluation, and Treatment of High Blood Pressure (JNC 7). An updated version of this report (JNC 8) was issued in 2013. In patients with cardiovascular disease, 0.036 to 0.054 mg of epinephrine (approximately two to three cartridges of local anesthetic with 1 : 100,000 epinephrine) should be safe for most patients except those with severe cardiovascular disease or other specific risk factors. Local anesthetics with vasoconstrictors should be avoided or used with extreme caution in patients with the following cardiovascular conditions: severe or poorly controlled hypertension, arrhythmias that are refractory to treatment, myocardial infarction within the past month, stroke within the past 6 months, coronary artery bypass graft within the past 3 months, and uncontrolled congestive heart failure. Patients unable to tolerate vasoconstrictors may not be good candidates for periradicular surgery, because a local anesthetic with a vasoconstrictor is essential for obtaining adequate hemostasis and visibility during this type of procedure. Surgery can be performed using only local anesthetics without vasoconstrictors, but this is not recommended.
The potential for multiple drug interactions is increasing because of the aging population and the introduction of many new drugs. Many elderly patients have diminished liver and kidney function and therefore cannot metabolize and excrete drugs as efficiently as younger, healthy patients. The potential for drug interactions and decreased metabolism and excretion of drugs should be considered even for commonly used agents such as local anesthetics and analgesics.
A transient bacteremia is a virtual certainty with periradicular surgery; therefore, patients at high risk for bacterial endocarditis should receive appropriate antibiotic prophylaxis as recommended by the American Heart Association. New guidelines for prevention of infective endocarditis were published in 2007 and represent a significant change from previous American Heart Association guidelines. For example, antibiotic prophylaxis is no longer recommended for patients with a history of mitral valve prolapse (with or without regurgitation), rheumatic heart disease, bicuspid valve disease, aortic stenosis, and certain congenital heart conditions. Antibiotic prophylaxis is now only recommended for patients with valvular disease associated with the highest risk of adverse outcomes from infective endocarditis. For patients in the highest risk category, antibiotic prophylaxis is recommended for dental procedures that involve manipulation of gingival tissues or the periapical region of teeth or perforation of the oral mucosa. For all other patients with valvular disease, the risks associated with routine antibiotic prophylaxis are greater than the potential benefits. Although these guidelines represent the best available current evidence, practitioners should be aware that a recent study from England found a significant increase in cases of infective endocarditis after widespread adoption of the new guidelines in 2008. However, the authors point out that their data do not establish a causal relationship and recommend adherence to the current guidelines at this time (Dayer MJ, et al. Lancet 2014).
New clinical practice guidelines for the management of patients with prosthetic joints were co-developed by the American Association of Orthopaedic Surgeons and the American Dental Association in December 2012. The working group conducted a systematic review and presented three recommendations. Recommendation 1 states that “the practitioner might consider discontinuing the practice of routinely prescribing prophylactic antibiotics for patients with hip and knee prosthetic joint implants undergoing dental procedures.” The level of evidence to support this recommendation was classified as “limited,” but that was the highest level of evidence assigned to any of the three recommendations. Two case-control studies provide evidence for this recommendation. In recommendation 2, the group was unable to recommend for or against the use of topical antimicrobial agents (grade of recommendation = inconclusive). Recommendation 3 was a consensus recommendation to support the maintenance of appropriate oral hygiene (grade of recommendation = consensus). A recent American Dental Association Council on Scientific Affairs report added further clarification by concluding that: “In general, for patients with prosthetic joint implants, prophylactic antibiotics are not recommended prior to dental procedures to prevent prosthetic joint infection.” (Sollecito TP, et al. JADA 2015).
Management of patients undergoing anticoagulant therapy depends on the type of anticoagulant, the reason for anticoagulant therapy, and the type of oral surgery planned. Warfarin (Coumadin), a commonly prescribed anticoagulant used to treat or prevent thromboembolisms, blocks the formation of prothrombin and other clotting factors. The international normalized ratio (INR) value is the accepted standard for measuring prothrombin time (PT). The desired therapeutic range for the INR usually is 2 to 3.5, depending on the underlying medical indication for anticoagulant therapy. Limited oral surgery procedures, such as simple forceps extraction of one to three teeth, may be performed safely on patients with INR values within the normal therapeutic range. Periradicular surgery, however, may present a greater challenge for hemostasis, even for patients well maintained within the therapeutic range. The clear field visibility normally required for proper surgical management of the root end may not be possible in patients undergoing anticoagulant therapy. The patient’s physician must be consulted for assistance in developing an appropriate treatment plan. Some patients may be able to tolerate discontinuation of warfarin therapy 2 days before a planned surgical procedure, which allows the INR to drift downward. In a prospective cohort study, Russo and colleagues reported that suspension of warfarin 2 days before a surgical procedure resulted in no bleeding problems and no thromboembolic events. They found that the average time spent at an INR below 2 (critical value) was 28 hours and that 90% of the patients returned to the desired therapeutic INR value within 7 days. However, this strategy may place certain patients at greater risk for a thromboembolic event; therefore, discontinuation of anticoagulant therapy would not be recommended.
In general, patients undergoing anticoagulant therapy should present minimal risk for significant bleeding during or after oral surgery, and local measures to control bleeding should be adequate. In a review prepared for the American Dental Association Council on Scientific Affairs and Division of Science, Jeske and Suchko recommended against routine discontinuation of anticoagulant therapy before dental procedures, including surgical procedures. Regardless of the management approach selected, consultation with the patient’s physician and an INR test on the day of surgery are strongly recommended. A retrospective study of an urban dental school population found that INR values for 43% of patients taking warfarin were not within the recommended therapeutic range. To underscore the challenge of maintaining anticoagulation levels within an appropriate therapeutic range, warfarin has been implicated in more emergency hospital admissions than any other medication.
Hospitalization and conversion to heparin therapy may be considered in special cases, but the patient, physician, and surgeon must carefully weigh the potential risks against the expected outcome and benefits. A new category of heparin anticoagulant, low-molecular-weight heparins (LMWHs), allows patient self-administration and may be an alternative for patients who need to maintain a high level of anticoagulation but want to reduce the cost and time required for traditional heparin conversion therapy.
Novel oral anticoagulant drugs (NOACs) have recently emerged as an alternative to warfarin for most indications (e.g., previous stroke, atrial fibrillation, and deep vein thrombosis) except mechanical heart valves. Examples include: apixaban (Eliquis), dabigratran (Pradaxa), edoxaban (Lixiana), and rivaroxaban (Xarelto). NOACs have fewer interactions with foods and other drugs, and there is no need for routine blood work monitoring. However, although NOACs are known to prolong prothrombin time (PT) by inhibiting factor X to Xa conversion, there is currently no reliable test to accurately measure level of anticoagulation. Medical consultation may be indicated to help evaluate the relative benefits and risks of discontinuing NOAC drugs for one or two days prior to endodontic surgery.
Low-dose aspirin therapy is known to increase bleeding time by irreversibly inhibiting platelet aggregation. A common practice has been to advise patients to discontinue aspirin therapy for 7 to 10 days before oral surgery. At low-dose therapeutic levels (less than 100 mg per day), aspirin may increase bleeding time and complicate surgical procedures. However, Ardekian and colleagues concluded that low-dose aspirin therapy should not be discontinued before surgical procedures and that bleeding could be controlled by local measures. Higher-dose therapy may create a greater risk of bleeding during or after surgery.
Although a patient undergoing aspirin therapy may not be at high risk for significant bleeding during or after surgery, a concern in periradicular surgery is the visibility problems created by oozing blood. The clinician should consult the patient’s physician about the medical reason for aspirin therapy and should weigh the risks and benefits of discontinuing aspirin before the proposed surgery. It should be possible to perform periradicular surgery without discontinuing aspirin therapy if necessary, but visibility during the procedure may be compromised, which may adversely affect the prognosis.
Herbs, dietary supplements, and vitamins can contribute to bleeding problems during surgery, and patients often fail to report use of these substances in the preoperative evaluation. In a survey of surgical patients, approximately one third reported using a nonprescription medication that might inhibit coagulation or interact with anesthetics. In particular, ginkgo biloba, ginger, garlic, ginseng, feverfew, and vitamin E inhibit platelet aggregation and can increase the risk of bleeding. Ingredients in over-the-counter (OTC) weight loss products can potentiate the effect of epinephrine and increase cardiac stress, although the most obvious example of this phenomenon, ephedra, has been removed from the U.S. market by a Food and Drug Administration (FDA) order.
Patients with inherited or acquired bleeding disorders are also at risk for excessive bleeding during and after periradicular surgery. Impaired liver function associated with past or current alcohol or drug abuse may also predispose a patient to excessive bleeding during surgery. A thorough medical history and patient interview should help identify these patients and, in consultation with the patient’s physician, allow for any necessary treatment modifications.
Many other medical conditions, such as recent myocardial infarction, stroke, cardiac arrhythmias, diabetes, radiation therapy for head and neck cancer, immunocompromising conditions, seizure disorders, adrenal suppression, liver or kidney disease, and pregnancy may require modification of the treatment plan. However, these conditions usually present more general problems that are not unique to a surgical procedure. The value of a thorough medical history and patient interview cannot be overemphasized.
Anatomic Considerations
Evaluating the access to the surgical site is one of the most important steps in case selection for periradicular surgery. Anatomic studies can provide some guidance, but individual variation is great, and there is no substitute for a complete clinical examination. A small oral opening, active facial muscles, shallow vestibule, and thick buccal alveolar bone all can significantly increase the difficulty of the procedure, even in cases that appear straightforward on radiographic examination.
Posterior Mandible
The primary anatomic structure of concern for periradicular surgery in the posterior mandible is the neurovascular bundle that courses through the mandibular canal and exits through the mental foramen. The relationship among the mandibular canal, the mental foramen, and the root apices of mandibular teeth has been well studied; however, anthropometric averages have limited value in the treatment of individual patients. An understanding of the typical anatomic relationships is important, but even more important is an evaluation of the individual patient to develop a case-specific risk assessment.
The depth of the vestibular fornix generally is a good predictor of the possible difficulty involved in obtaining surgical access to the mandibular posterior teeth. A shallow vestibule usually portends thicker alveolar bone and more difficult access to the root end.
The mental foramen, another key anatomic structure, usually is located between and apical to the mandibular first and second premolars ; however, this varies considerably, and the practitioner must examine each patient carefully to determine its location ( Figs. 9-5 and 9-6 ). Vertical location of the mental foramen may vary even more than horizontal location. Moiseiwitsch found that the average location was 16 mm inferior to the cementoenamel junction (CEJ) of the second premolar, although the range was 8 to 21 mm, which would place approximately 20% of the foramina at or coronal to the root apex. Fortunately, the mental foramen usually is easily visualized with standard periapical and panoramic radiographs. A vertically positioned periapical radiograph often may be more useful than a horizontally positioned one, especially in individuals with longer roots. Also, the mental foramen usually can be palpated.


When a vertical releasing incision is indicated, it typically is made at the mesial line angle of the mandibular canine. This location is always mesial to the mental foramen, because the foramen is located in the area ranging from the apex of the mandibular first premolar to slightly distal to the second premolar. The nerve bundle exits from the foramen in a distal direction. An alternative technique for gaining access to mandibular posterior teeth involves a distal releasing incision between the mandibular first and second molars. This approach may be especially useful for access to the mandibular second premolar and first molar. Care must be taken to avoid the facial artery as it crosses the level of the inferior vestibular fornix near the mandibular first molar. Inadvertent contact with the facial artery is unlikely if the incision is not extended beyond the depth of the vestibule.
Regardless of the approach selected, it obviously is important to avoid making an incision in the immediate vicinity of the mental foramen. The mental nerve is encased in a relatively tough sheath, and permanent damage can be avoided if careful blunt dissection is used in the area. A misguided vertical releasing incision, however, may sever the nerve, causing permanent injury. Trauma to the nerve from blunt dissection in this area or from pressure from a misplaced retractor may cause temporary paresthesia but is much less likely to do permanent injury. (Documentation and management of nerve injuries are discussed later in the chapter.)
The borders of the mandibular canal often are more difficult to visualize with conventional radiographic techniques. A parallel periapical radiograph, either horizontally or vertically positioned, usually can provide a reasonably accurate image of the relationship between the superior border of the mandibular canal and the root apices. However, the mandibular canal sometimes cannot be readily visualized. Such cases should be approached with extreme caution, because an increased risk of paresthesia secondary to nerve injury may be an unacceptable risk for many patients. Cone-beam computed tomography (CBCT) imaging can be very useful for identifying the location of the mandibular canal and determining its relationship to the root apices (see Chapter 2 ).
In the buccolingual dimension, the mandibular canal commonly follows a curving pathway from the buccal half of the mandible near the distal root of the second molar to the lingual half of the mandible near the first molar, then curving back to the buccal near the second premolar as it exits the mental foramen. The average vertical distance from the superior border of the mandibular canal to the distal root apex of the mandibular second molar is approximately 3.5 mm; this increases gradually to approximately 6.2 mm for the mesial root of the mandibular first molar and to 4.7 mm for the second premolar. This relationship typically provides a greater margin of safety for surgery on the mandibular first molar compared with the second premolar and especially the second molar. Surgery on the mandibular second molar may be further complicated by relatively thick overlying buccal bone, lingual inclination of the roots, and a more buccal location of the mandibular canal. This is not to say that periradicular surgery should not be performed on mandibular second molars; rather, it points out that the relative risks and benefits must be carefully considered. Often the most prudent choice for a mandibular second molar is an intentional replantation procedure or extraction and placement of an implant.
Posterior Maxilla
The primary anatomic structure of concern in the posterior maxilla is the maxillary sinus. CBCT allows for a more precise preoperative three-dimensional evaluation of the relationship between the roots of maxillary posterior teeth and the sinus. Perforation of the sinus during surgery is fairly common, with a reported incidence of about 10% to 50% of cases. Even without periradicular pathosis, the distance between the root apices of the maxillary posterior teeth and the maxillary sinus sometimes is less than 1 mm. An inflammatory periradicular lesion often increases the likelihood of sinus exposure during surgery. Fortunately, perforation of the maxillary sinus rarely results in long-term postoperative problems. In a report of 146 cases of sinus exposure during periradicular surgery, Watzek and colleagues found no difference in healing compared with similar surgical procedures without sinus exposure. The sinus membrane usually regenerates, and a thin layer of new bone often forms over the root end, although osseous regeneration is less predictable. The general rule of placing a vertical releasing incision at least one tooth mesial and distal to the surgical site is especially important when sinus perforation is possible, because the exposure site should be completely covered with the mucoperiosteal flap to provide primary closure.
If the maxillary sinus is entered during surgery, special care must be taken to prevent infected root fragments and debris from entering the sinus. The most commonly used root resection technique involves grinding the root apex with a high-speed drill for approximately 3 mm in an apical to coronal direction; therefore, an opening could allow infected debris into the sinus. A sinus opening can be temporarily occluded with a material such as Telfa gauze, although the gauze should be secured so as to prevent inadvertent displacement into the sinus. A suture can be placed through the packing material to prevent displacement and aid retrieval. Jerome and Hill suggested securing the apical root segment by drilling a small hole in the root tip and threading a piece of suture material through the hole. The root is then resected at the appropriate level, and the root-end fragment is removed in one piece. If a root fragment or other foreign object is displaced into the sinus, it should be removed. An orascope or endoscope may be useful for visualizing the foreign object, but referral for evaluation and surgical removal may be indicated if the fragment cannot be located and removed.
The palatal roots of maxillary molars present a special challenge for surgical access. Palatal roots may be reached from either a buccal (transantral) or palatal approach. Wallace described a transantral approach in which a buccal flap is reflected, the buccal roots are resected, the osteotomy access into the sinus is enlarged to approximately 1 by 1.5 cm, and the palatal root tip is resected, ultrasonically prepared, and filled. The sinus may be packed with moist gauze to catch debris, and it should be irrigated with sterile saline upon completion of the surgery. In some cases, careful reflection and retraction of the sinus membrane may be possible, allowing access to the palatal root end without direct invasion of the sinus. Many who have tried this approach find it more challenging than it might initially seem. The enhanced illumination and magnification provided by a dental operating microscope, endoscope, or orascope are essential aids in this type of surgery.
A palatal approach to the palatal root of maxillary molars may seem more direct than a transantral approach, but it can present certain difficulties. Visibility in the surgical field is reduced and manipulation of instruments is more difficult than with most routine buccal approaches. Patients with a deep, vertical palatal vault are better candidates for this approach than individuals with a wide, shallow palate. A sinus tract or a large lesion on the palatal root may allow easier access and better visualization of the palatal root, because only limited bone removal would be required. The position of the anterior palatine artery must be carefully considered when the incision is made and the flap reflected. This artery emerges from the greater palatine foramen distal to the maxillary second molar at the junction of the vertical section of the alveolar process and the flat portion of the palate and continues anteriorly ( Fig. 9-7 ). A vertical releasing incision can be placed between the maxillary first premolar and canine, where the artery is relatively narrow and branches off into smaller arteries. If needed, a short distal vertical releasing incision may be made distal to the second molar, but it should not approach the junction of the alveolar process and roof of the palate. If the anterior palatine artery is severed, local clamping and pressure may not stop the hemorrhage, and ligation of the external carotid artery may be necessary. Because of the palatal vault’s concave shape, repositioning the flap may be challenging. An acrylic surgical stent may be fabricated before surgery to assist repositioning of the flap and to help prevent pooling of blood under the flap.

Anterior Maxilla and Mandible
Periradicular surgery on anterior teeth generally involves fewer anatomic hazards and potential complications than in posterior teeth. Nonetheless, access to the root apex in some patients may be unexpectedly difficult because of long roots, a shallow vestibule, or lingual inclination of the roots. As shown in Figure 9-5 , the root apices of maxillary central and lateral incisors can be quite close to the floor of the nose and the bony anterior nasal spine. Figure 9-8 demonstrates isolation and protection of the nasopalatine neurovascular bundle during maxillary anterior surgery. The average maxillary canine is about 26 mm long and usually presents no difficulty for surgical access; however, the combination of a shallow vestibule and a longer than average root length could complicate access to the root apex area. In such cases, the creation of an osteotomy apical to the root end may be impossible. An alternative approach for long-rooted teeth and root tips in the vicinity of critical anatomic structures is to enter the bone and resect the root at a level approximately 3 mm coronal to the apex. After the root tip is removed, the area apical to the root can be inspected and curetted as needed.

Periradicular surgery on mandibular incisors often is more challenging than expected. The combination of lingual root inclination, a shallow vestibule, and a prominent mental protuberance all can increase the degree of difficulty, as can proximity to adjacent roots and the need for perpendicular root-end resection and preparation to include a possible missed lingual canal.
Cone-Beam Computed Tomography
Radiographic examination is an essential component in all aspects of endodontic treatment from diagnosis and treatment planning to assessing outcome (see also Chapter 2 ). Information gained from conventional films and digital periapical radiographs is limited by the fact that the three-dimensional anatomy of the area is compressed into a two-dimensional image. As a result of superimposition, periapical radiographs reveal limited aspects of the three-dimensional anatomy. In addition, there may also be geometric distortion of the anatomic structures imaged. These problems may be overcome using small volume cone-beam computed tomography (CBCT) imaging techniques, which can produce three-dimensional images of individual teeth and the surrounding tissues. CBCT may be particularly useful in diagnosis and treatment planning for periradicular surgery ( Fig. 9-9 ). The term cone-beam computed tomography is often used interchangeably with cone-beam volumetric tomography (CBVT).

Differences Between CT and CBCT Imaging
The benefits of three-dimensional medical computer tomography (CT) imaging are already well established in certain dental specialties. Current CT scanners have a linear array of multiple detectors, allowing multiple slices to be taken simultaneously, resulting in faster scan times and often less radiation exposure to the patient. The slices of data are then “stacked” and can be reformatted to obtain three-dimensional images. The high-radiation dose, cost, availability, poor resolution, and difficulty in interpretation have resulted in limited use of CT imaging in endodontics. These issues may be addressed by cone-beam innovations in CBCT technology.
In 2000, the Food and Drug Administration approved the first CBCT unit for dental use in the United States. Cone-beam technology uses a cone-shaped beam of radiation to acquire a volume in a single 360-degree rotation, similar to panoramic radiography. The volume of acquired images by a CBCT is composed of voxels. Essentially, a voxel is a 3D pixel. Because the data are captured in a volume as opposed to slices, all the voxels are isotropic, which enables objects within the volume to be accurately measured in different directions. Unlike the CBCT voxel, a medical CT voxel is not a perfect cube, and measurements made in multiple planes are not accurate. In addition to increased accuracy and higher resolution, CBCT offers significant scan-time reduction, radiation dose reduction, and reduced cost for the patient. CBCT systems can be classified into two categories: limited (dental or regional) CBCT or full (ortho or facial) CBCT. The field of view (FOV) of limited CBCT ranges in diameter from 40 to 100 mm, whereas the FOV of full CBCT ranges from 100 to 200 mm. Another difference between the limited CBCT and full CBCT is that a voxel is generally smaller for the limited version (0.1 to 0.2 mm versus 0.3 to 0.4 mm). Thus, limited CBCT systems offer higher resolution and are better suited for endodontic applications. Limited volume CBCT scanners capture small volumes of data that can include just two or three individual teeth. The most important and clinically useful feature of CBCT technology is the highly sophisticated software that allows the huge volume of data collected to be reconstructed. Tomographic slices, as thin as one voxel, may be displayed in a number of ways. One option, for example, is for the images to be displayed in the three orthogonal planes—axial, sagittal and coronal—simultaneously. The axial and proximal (sagittal in the anterior, coronal in the posterior) views are of particular value, because they are generally not seen with conventional periapical radiography. The ability to reduce or eliminate superimposition of the surrounding structures makes CBCT superior to conventional periapical radiography.
Scan times are typically 10 to 40 s, although the actual exposure time is significantly less (2 to 5 s) as scans involve a number (up to 360) of separate, small, individual exposures rather than one continuous exposure. With medical CT scanners, the scanning and exposure times for the skull can be significantly longer. Most CBCT scanners are much smaller than medical CT scanners, taking up about the same space as a dental panoramic machine. They are also significantly less expensive than medical CT scanners. With the help of viewer software, the clinician is able to scroll through the entire volume and simultaneously view axial, coronal, and sagittal 2D sections that range from 0.125 to 2 mm thick. Comparing the radiation dose of different CBCT scanners with medical CT scanners may be confusing because different units of radiation dose are often used. There are three basic measurement units in radiation dosimetry: the radiation absorbed dose (D), the equivalent dose (H), and the effective dose (E). The radiation absorbed dose is defined as the measure of the amount of energy absorbed from the radiation beam per unit mass of tissue and is measured in joules per kilogram. The equivalent dose is defined as a measure that indicates the radiobiologic effectiveness of different types of radiation and thus provides a common unit. The effective dose is calculated by multiplying the equivalent dose by different tissue weighting factors, which converts all doses to an equivalent whole-body dose and allows doses from different investigations of different parts of the body to be compared. The unit remains as the Sievert (Sv) and can be used to estimate the damage from radiation to an exposed population. The reported effective doses from CBCT scanners vary but can be almost as low as those from panoramic dental x-ray units and considerably less than that from medical CT scanners. The higher effective doses from particular models of CBCT scanners is in part due to the larger size of the field of view used as well as the type of image receptor employed. The small volume scanners capture information from a small region of the jaw, approximately the same area as a periapical radiograph. The effective dose has been reported to be in the same range as 2 to 3 standard periapical radiograph exposures, whereas the effective dose for a full mouth series of periapical radiographs has been reported to be similar to the effective dose of a large volume CBCT scan. If multiple teeth in different quadrants require endodontic evaluation or treatment, a large volume CBCT scan with a large field of view may be more appropriate. If endodontic information is required from multiple teeth in one jaw, a large volume CBCT scan with the field of view only limited to the jaw of interest could be the investigation of choice. This has the advantage of reducing the effective dosage from the full large volume CBCT scan by up to 65%.
Potential Applications of CBCT in the Management of Endodontic Posttreatment Disease
Clinical radiographic examinations are usually limited to two-dimensional views captured using radiographic film or digital sensors. Crucial information related to the true three-dimensional anatomy of teeth and adjacent structures is obscured. Even with paralleling techniques, distortion and superimposition of dental structures in periapical views is unavoidable. A major reported advantage of CBCT is the accuracy of measurements in all dimensions. The ability to view thin sagittal, coronal, and axial slices effectively eliminates the problem of superimposition of anatomic structures. For example, roots of maxillary posterior teeth and surrounding tissues can be viewed without superimposition of the zygomatic buttress, alveolar bone, maxillary sinus, and other roots ( Fig. 9-10 ). CBCT enables clinicians to detect changes in apical bone density at an earlier stage when compared to conventional periapical radiographs and therefore has the potential to detect previously undiagnosed periradicular pathoses. CBCT may also prove to be a useful tool for noninvasive differentiation of periapical cysts and granulomas, a previously unattainable goal using conventional radiographic imaging.


Three-dimensional imaging allows for clear identification of the anatomic relationship of the root apices to important neighboring anatomic structures such as the mandibular canal, mental foramen, and maxillary sinus ( Fig. 9-11 ). Velvart and colleagues reported that the relationship of the inferior alveolar canal to the root apices could be determined in every case when utilizing medical CT, but in less than 40% of cases when using conventional radiography. It is likely that similar results could be achieved with CBCT using considerably less radiation. Rigolone and coworkers concluded that CBCT may play an important role in periapical microsurgery of palatal roots of maxillary first molars. The distance between the cortical plate and the palatal root apex could be measured using CBCT and the presence of the maxillary sinus between the roots could be assessed. Additional information such as the thickness of the cortical plate, cancellous bone pattern, fenestrations, and inclination of the roots can be obtained prior to surgical entry. Root morphology (shape, size, curvature, and number of canals) can be visualized in three dimensions. Unidentified (and untreated) canals in root-filled teeth can be routinely visualized in axial slices. CBCT has been used to determine the location and extent of invasive external root resorptive defects.

One of the most useful potential applications of CBCT may be the assessment of endodontic treatment outcomes, both nonsurgical and surgical. When compared to two-dimensional film and digital images, CBCT is dimensionally precise and can detect smaller changes in bone density.
It is worth remembering that CBCT still uses ionizing radiation and is not without risk. It is essential that patient exposure is kept as low as reasonably practical and that justifiable selection criteria for CBCT use are developed. CBCT may be indicated when it is determined that the additional information obtained is likely to result in a more accurate diagnosis and enhanced patient safety.
Patient Preparation for Surgery
Informed-Consent Issues Specific to Surgery
The general principles of informed consent discussed in Chapter 29 form the basis for informed consent for periradicular surgery. The patient must be thoroughly advised of the benefits, risks, and other treatment options and must be given an opportunity to ask questions. The main consent issues specific to surgical procedures are closely related to the anatomic considerations discussed in the previous section. That is, major neurovascular bundles may be traumatized, and a sinus exposure may occur. Paresthesia after mandibular posterior surgery is uncommon but should be discussed with the patient because this potential complication is a risk some patients may be unwilling to assume. Postoperative swelling, bruising, bleeding, and infections are possible complications that typically are self-limiting or readily manageable. Although the incidence of serious complications related to surgical procedures is very low, patients should be advised of any risks unique to their situation. Prompt attention to any surgical complications and thorough follow-up are essential from a medical-legal standpoint.
Premedication: NSAIDs, Antibiotics, Chlorhexidine, and Conscious Sedation
Administration of an NSAID, either before or up to 30 minutes after surgery, enhances postoperative analgesia. NSAIDs generally have proved more effective in the management of postoperative oral surgery pain than placebo or acetaminophen and codeine combinations. The combination of preoperative administration of an NSAID and use of a long-acting local anesthetic may be particularly helpful for reducing postoperative pain. Many types of NSAIDs are available, but ibuprofen remains the usual standard for comparison. Ibuprofen (400 mg) provides analgesia approximately equal to that obtained with morphine (10 mg) and significantly greater than that from codeine (60 mg), tramadol (100 mg), or acetaminophen (1000 mg). The analgesic effectiveness of ibuprofen tends to level off at about the 400-mg level (ceiling effect), although a slight increase in analgesic potential may be expected in doses up to 800 mg. (See Chapter 4 for further discussion of analgesics.)
The value of antibiotic prophylaxis before or after oral surgery is controversial, and the current best available evidence does not support the routine use of prophylactic antibiotics for periradicular surgery. For most patients, the risks of indiscriminate antibiotic therapy are believed to be greater than the potential benefits. The incidence of infection after oral surgery in healthy patients is very low. Peterson reported that only 1% of patients developed infections after third molar extractions. A systematic review of the use of antibiotics to prevent complications after placement of dental implants found that evidence was lacking either to recommend or discourage the use of antibiotics for this purpose. However, the use of prophylactic antibiotics for more invasive procedures, such as orthognathic surgery, has significantly reduced the risk of postoperative infection and complications. Although routine use of prophylactic antibiotics for periradicular surgery is not currently recommended, clinical judgment is important in determining exceptions to the general rule. For example, immunocompromised patients may be good candidates for prophylactic antibiotic coverage. Certain categories of medically complex patients also may benefit from antibiotic coverage. Diabetic patients have shown impaired healing capacity after nonsurgical root canal treatment, and a like pattern of delayed or impaired healing may emerge in studies of surgical outcomes. The global problems associated with overprescription of antibiotics are significant and should prompt due caution in the decision on whether to use these drugs prophylactically.
Chlorhexidine gluconate (0.12%) often is recommended as a mouth rinse to reduce the number of surface microorganisms in the surgical field, and its use may be continued during the postoperative healing stage. Although no solid evidence supports this practice in periradicular surgery, the use of chlorhexidine follows from the general surgical principle of surface disinfection before incision and opening into a body cavity. In addition, chlorhexidine has proved to be a safe, effective adjunct in the treatment of periodontitis, and short-term use (i.e., several days) poses little or no risk. Chlorhexidine may be useful for reducing the risk of postoperative infection after oral surgery, although the evidence in this area is conflicting. Postoperative use of chlorhexidine mouth rinse can reduce bacterial growth on sutures and wound margins, but it may interfere with fibroblast reattachment to the root surface. A useful empirical regimen is to have the patient rinse for 30 seconds twice a day beginning 1 or 2 days before surgery and continuing until the sutures are removed.
Conscious sedation, either by an orally administered sedative or by nitrous oxide/oxygen inhalation analgesia, may be useful for patients who are anxious about the surgical procedure or dental treatment in general. Benzodiazepines with a short half-life are particularly useful because they generally have a wide margin of safety, good absorption after oral administration, and limited residual sedative effects. When these drugs are used in sedative-hypnotic doses, the blood pressure, pulse, and respiration must be monitored, and states differ in their requirement for additional training or certification of staff members who do this monitoring. With oral conscious sedation, pulse oximetry should be used to monitor the pulse and blood oxygen saturation during the surgery. As with all orally administered drugs, the dosage cannot be closely titrated; therefore, the effect of the agent varies somewhat. A typical protocol is a single dose at bedtime the evening before the procedure and a second dose 1 hour before the start of surgery. The patient should not drive to or from the office and should have a responsible adult for assistance as needed. In appropriate doses, benzodiazepines and similar drugs may allow for a more relaxed patient and thus a less stressful surgical experience for both patient and surgeon.
Instruments and Operatory Setup
The development of microsurgical techniques and new materials has changed the typical surgical instrument tray dramatically. Instruments have been designed to take full advantage of the increased visibility obtained with dental operating microscopes, endoscopes, and orascopes. Better visualization of the surgical site would have limited value without microsurgical instruments, such as ultrasonic tips for root-end preparation and micromirrors for inspecting the root end. Figure 9-12 shows a typical basic surgery tray arrangement. This setup is not a definitive guide to surgical armamentarium but rather an adequate, efficient starting point for most periradicular surgical procedures. Although the number of instruments can easily be doubled or even tripled, the ease of locating a specific instrument is inversely proportional to the number of instruments on a tray. Specialized instruments can be kept readily available in separate sterilized bags or trays and opened as needed. A skilled surgeon can use a wide variety of instruments ( Figs. 9-12 to 9-24 ) to achieve excellent results.













Periradicular surgery can be performed without the benefit of enhanced magnification and illumination; however, those who use microscopes, endoscopes, and orascopes report dramatically improved visualization and control of the surgical site. Studies reporting the highest surgical success rates and those cited previously in this chapter all involved some form of enhanced magnification and illumination as part of the standard operating protocol. A systematic review and meta-analysis found that the outcome for endodontic surgery performed with high magnification and illumination (microscope or endoscope) was significantly higher than surgery performed with loupes only or no magnification.
Local Anesthesia for Surgery
Local anesthesia for surgical root canal procedures differs from that for nonsurgical root canal treatment primarily in the need for localized hemostasis in addition to profound local anesthesia. In fact, use of a local anesthetic with a vasoconstrictor may be the single most important local measure to help control hemorrhage and provide a clear surgical field. Otherwise, the same regional block and local infiltration techniques used for nonsurgical treatment (see Chapter 4 ) are used for surgical root canal procedures. Infiltration of the surgical site with a local anesthetic containing 1 : 50,000 epinephrine is the technique of choice to obtain vasoconstriction and hemostasis. The local anesthetic is first slowly deposited in the buccal root apex area of the alveolar mucosa at the surgical site and extended two or three teeth on either side of the site. Usually palatal or lingual infiltration is also required, although this requires a much smaller amount of local anesthetic than the primary buccal infiltration. After the injections for anesthesia, the surgeon should wait at least 10 minutes before making the first incision.
Long-acting local anesthetics (e.g., 0.5% bupivacaine with 1 : 200,000 epinephrine) have been shown to reduce postoperative pain and analgesic use after surgical removal of impacted third molars. However, use of a local anesthetic with 1 : 200,000 epinephrine may result in greater blood loss during surgery. To maximize postoperative analgesia and minimize intraoperative bleeding, a local anesthetic can be used with higher epinephrine concentrations (1 : 100,000 or 1 : 50,000) for the primary surgical anesthesia and supplemented with one cartridge of long-acting local anesthetic immediately after surgery. Long-lasting local anesthetics are particularly beneficial in mandibular surgery but much less so for surgery in the maxillary arch.
Every effort must be made to ensure profound local anesthesia before surgery begins. Usually a minimum of 10 to 20 minutes is required from the time of injection to the start of surgery to ensure both profound local anesthesia and adequate vasoconstriction for hemostasis. The patient should be asked about the usual signs of soft-tissue anesthesia, and a sharp explorer can be used to test the surgical area for sensation. Even when the surgeon pays careful attention to local anesthetic technique, a patient sometimes has inadequate anesthesia or loss of anesthesia during the surgical procedure. Providing supplemental infiltration anesthesia is difficult after a full-thickness flap has been reflected. A supplemental block injection may be useful for mandibular teeth and maxillary posterior teeth. In the maxillary anterior area, a palatal approach to the anterior middle superior nerve may be helpful. The key to this approach is slow injection of approximately 1 mL of local anesthetic in the area of the first and second maxillary premolars midway between the gingival crest and the palatal midline. An intraosseous injection also may be used to regain lost anesthesia, but even when it is effective, the area of local anesthesia often is smaller than desired for a surgical procedure. As a last resort, the procedure can be terminated short of completion and the patient can be rescheduled for surgery under sedation or general anesthesia.
Surgical Access
The goals of periradicular surgery are to access the affected area, remove the diseased tissue, evaluate the root circumference and root canal system, and place a biocompatible seal in the form of a root-end filling that can stimulate regeneration of the periodontium. The formation of new cementum on the surgically exposed root surface and on the root-end filling material is essential to regeneration of the periodontium.
Successful endodontic surgery requires the surgeon to use several conceptual elements in planning the procedure. A vision of the immediate postoperative surgical end point (i.e., replacement of the reflected tissues) is essential for designing each phase of the surgery. Visualization of a three-dimensional image of the surgical procedure allows the surgeon to anticipate and prepare for unusual circumstances. In surgical root canal treatment, once a procedure is started, it must be completed uninterrupted within a limited time; this is a paramount difference between nonsurgical and surgical root canal treatment. For this reason, it is absolutely essential that the surgeon plan the procedure thoroughly and include alternative plans of action that anticipate unusual findings during the process of discovery.
Several general principles are important for designing the access to a diseased region: (1) the surgeon must have a thorough knowledge of the anatomic structures in relation to each other, including tooth anatomy; (2) the surgeon must be able to visualize the three-dimensional nature of the structures in the soft and hard tissue (this reduces unnecessary tissue damage); (3) the trauma of the surgical procedure itself must be minimized, which includes the preservation of tooth and supporting structures; (4) the tissue and instruments must be manipulated within a limited space with the aim of removing diseased tissues and retaining healthy tissues.
Soft-Tissue Access
In designing the soft-tissue access window to the diseased tissue, the surgeon must take into consideration various anatomic features, such as frenum-muscle attachments, the width of attached gingiva, papillary height and width, bone eminence, and crown margins. The supraperiosteal blood vessels of the attached gingiva extend from the alveolar mucosa and run parallel to the long axis of the teeth, lying in the reticular layer superficial to the periosteum. A vertical, rather than an angled, releasing incision severs fewer vessels, reducing the possibility of hemorrhage. Also, the blood supply to the tissue coronal to the incision is not compromised, which prevents localized ischemia and sloughing of these tissues. Ultimately, the result is less bleeding during the procedure and enhanced healing. For these reasons, an angled releasing incision is contraindicated in periradicular surgery.
Vertical Incision
The general principles for placement of a vertical relieving incision are as follows:
- 1.
The incision should be made parallel to the supraperiosteal vessels in the attached gingiva and submucosa ( Fig. 9-25 ).
FIG. 9-25 Intrasulcular incision with two vertical releasing incisions (rectangular flap). - 2.
No cuts should be made across frenum and muscle attachments.
- 3.
Frenum and muscle attachments should not be located in the reflected tissue, if possible.
- 4.
The incision should be placed directly over healthy bone.
- 5.
The incision should not be placed superior to a bony eminence.
- 6.
The dental papilla should be included or excluded but not dissected.
- 7.
The incision should extend from the depth of the vestibular sulcus to the midpoint between the dental papilla and the horizontal aspect of the buccal gingival sulcus.
Horizontal Incision
Three types of horizontal incisions can be used to gain access to a surgical site in hard tissue:
- •
An intrasulcular incision that includes the dental papilla. This incision extends from the gingival sulcus through the PDL fibers and terminates at the crestal bone of the alveolar bone proper. The incision then passes in a buccolingual direction adjacent to each tooth of the dental papilla and includes the midcol region of each dental papilla. Thus, the entire dental papilla is completely mobilized.
- •
An intrasulcular incision that excludes the dental papilla (papillary-based incision, Fig. 9-26 ). This technique consists of a shallow first incision at the base of the papilla and a second incision directed to the crestal bone.
FIG. 9-26 Papillary-based incision with one vertical releasing incision. - •
An incision made in the attached gingiva (a submarginal or Ochsenbein-Luebke flap). With this technique, at least 2 mm of attached gingiva must be retained to prevent mucogingival degeneration. Consequently, the incision must be placed at least 2 mm from the depth of the gingival sulcus. Extensive periodontal probing should be done to establish the depth of the gingival sulcus before the incision is made. The average width of the attached gingiva is 2.1 to 5.1 mm in the maxilla and 1.8 to 3.8 mm in the mandible. It is widest over the central and lateral incisors, narrows over the canine and the first premolar, and then widens over the second premolar and the first molar. These variances were similar for both the maxilla and mandible. Overall, this incision technique has a narrow margin of safety. It generally is recommended for use in the maxilla, especially where the aesthetics of existing crown margins are a concern.
Studies have compared the incision techniques that include and exclude the dental papilla in patients with healthy marginal periodontal conditions. These researchers found that the papilla base incision resulted in rapid, recession-free healing. In contrast, complete mobilization of the papilla led to a marked loss of papilla height. The authors suggested that use of the papilla base incision in aesthetically sensitive regions could help prevent papilla recession and surgical cleft, or double papilla.
Flap Design
Combinations of vertical and horizontal incisions are used to achieve various flap designs ( Figs. 9-27 and 9-28 ). The full mucoperiosteal and limited mucoperiosteal are the two major categories of flap design used during periradicular surgery, the main differentiating feature being the position of the horizontal incision. In each case the entire body of soft tissue is reflected as one unit and includes the alveolar mucosa, the gingival tissues, and the periosteum. The number and position of the vertical relaxing incisions therefore governs the major variation in design:
- 1.
Full mucoperiosteal (intrasulcular incision including the dental papilla or papillary based) ( Figs 9-27 and 9-28 ):
- a.
Triangular: one vertical relieving incision
- b.
Rectangular: two vertical relieving incisions
- c.
Trapezoidal: two angled vertical relieving incisions
- d.
Horizontal: no vertical relieving incision
- a.
- 2.
Limited mucoperiosteal ( Figs 9-29 and 9-30 ):
- a.
Curve submarginal (semilunar)
- b.
Freeform rectilinear submarginal (Ochsenbein-Luebke)
FIG. 9-29 Submarginal (Ochsenbein-Luebke) flap.FIG. 9-30 Clinical case involving a submarginal incision and flap. A, Periodontal probing of the entire area was performed before this type of flap was selected and the incision started. A submarginal incision often is used in aesthetic anterior areas of the mouth where postoperative gingival recession might expose the crown margins. B, The incision should be at least 2 mm apical to the depth of the sulcus. C, With the flap reflected, osteotomy and root-end resection were performed. Methylene blue dye was placed to mark the outline of the root end and to help identify cracks or fractures before root-end cavity preparation and filling. D, The flap was repositioned and sutured with 5-0 Tevdek.(Courtesy Dr. Martin Rogers.) - a.


Tissue Reflection
Elevation and reflection of the entire mucoperiosteal complex, maintaining the microvasculature in the body of the tissue flap, increases hemostatic control during surgery. Tissue reflection should begin from the vertical releasing incision at the junction of the submucosa and the attached gingiva ( Figs. 9-31 and 9-32 ). By initiating the reflection process at this point, damage to the delicate supracrestal root-attached fibers is avoided. Using a flap reflection technique that reduces reflective tissue forces in the intrasulcular incisional wound and avoids curettage of the root surface conserves the root-attached tissues and helps prevent apical down-growth of epithelium and loss of soft-tissue attachment. Force should be applied such that the periosteum and superficial tissues are reflected as a complete unit. Using a gentle rocking motion, the surgeon initially should reflect the tissue in a horizontal direction. The underlying bone of the cortical plate is irregular, and it is critical to avoid damaging the fragile tissues during elevation.


The operator should take great care not to slip during the tissue reflection process by using an appropriate instrument stabilized with adequate finger support. Slipping can result not only in puncture of the immediate overlying tissue but also damage to the surrounding structures.
As space permits, the elevator should be directed coronally, undermining the attached gingiva. As the interdental papilla is approached, a narrower instrument may be required to undermine and gently elevate the tissue in this region, to avoid crushing the delicate free gingival tissues. This process should be continued gradually until the osseous tissues overlying the diseased tooth structure have been adequately exposed. Generally, elevation of the flap 0.75 cm apical to the estimated apex of the root should allow adequate space to perform the surgical procedure.
No single instrument is essential for the flap elevation procedure, because every instrument has both advantages and disadvantages. Surgeons should familiarize themselves with the various instruments available.
Tissue Retraction
After the tissue is reflected, it must be retracted to provide adequate access for bone removal and root-end procedures. The main goals of tissue retraction are to provide a clear view of the bony surgical site and to prevent further soft-tissue trauma. Accidental crushing of the soft tissues leads to more postoperative swelling and ecchymoses.
The general principles of retraction are as follows: (1) retractors should rest on solid cortical bone; (2) firm but light pressure should be used; (3) tearing, puncturing, and crushing of the soft tissue should be avoided; (4) sterile physiologic saline should be used periodically to maintain hydration of the reflected tissue; and (5) the retractor should be large enough to protect the retracted soft tissue during surgical treatment (e.g., prevent it from becoming entangled in the bone bur). No one retractor suffices for all surgical procedures, therefore the surgeon should have a selection of retractors available for the various situations that arise during surgery. If difficulty is encountered in stabilizing the retractor, a small groove can be cut into the cortical plate to support it.
Hard-Tissue Access
Two biologic principles govern the removal of bone for hard-tissue access to diseased root ends: (1) healthy hard tissue must be preserved, and (2) heat generation during the process must be minimized.
Temperature increases above normal body temperature in osseous tissues are detrimental. Heating osseous tissue to 117° to 122° F (47° to 50° C) for 1 minute significantly reduces bone formation and is associated with irreversible cellular damage and fatty cell infiltration. Two critical factors determine the degree of injury: how high the temperature increases and how long it remains elevated. As the temperature rises above 104° F (40° C), blood flow initially increases. It stagnates at 198° F (46° C) applied for 2 minutes. Heating osseous tissue to 133° F (56° C) deactivates alkaline phosphatase. Studies using animal bone have shown that at temperatures above 109° F (42.5° C), for every 1° C elevation in temperature, the exposure time for the same biologic effect decreases by a factor of approximately 2. Temperatures above 117° F (47° C) maintained for 1 minute produce effects similar to those at 118° F (48° C) applied for 30 seconds. This correlation means that the decisive exposure time declines quickly as the temperature increases. Temperatures above 127° F (53° C) applied for less than 1 second can adversely affect osteogenesis.
Several factors determine the amount of heat generated during bone removal, including the shape and composition of the bur, the rotational speed, the use of coolant, and the pressure applied during cutting.
The round bur has the best shape for removing osseous tissue, and it should be used with a gentle brushstroke action. This type of bur also readily allows access of coolant to the actual cutting surfaces. Studies comparing the heat generated with round and fissure burs found more favorable results with the round burs. Cutting with round burs produced a wound site with less inflammation, which is more favorable for rapid wound healing. Although fissure-type burs cut efficiently on the sides, the tip of the bur is very inefficient because it allows no coolant access. The net result is increased inflammation and a reduced healing response.
Use of a diamond bur to remove osseous tissue is inefficient and retards ultimate wound healing. Because of its larger surface area, more of a diamond bur is in contact with the bone tissue. As a result, less coolant reaches the cutting surface, and the bur has a greater tendency to become clogged with residual bone fragments. The net effect is greater heat generation, increased inflammation, and reduced healing.
Use of a coolant during bone cutting is essential. If an appropriate irrigant is not used, temperatures can exceed those known to impair bone healing ; histologically, healing can be delayed up to 3 weeks. It also is critical that the coolant reach the cutting surface. Temperatures can rise above 212° F (100° C) when excess pressure is applied during cutting; this burrows the bur into the bone, where little or no irrigant can reach the cutting tip, hence the recommendation for a gentle brush-stroke technique. Favorable results are obtained with these provided the surgeon follows the basic tenet of minimizing heat generation: using a round fluted bur with coolant and a brushstroke technique. A high-speed handpiece that exhausts air from the base rather than the cutting end is recommended to reduce the risk of air embolism ( Fig. 9-33 ).

Periradicular Curettage and Biopsy
Most periradicular lesions originate in the pulp and can be classified histopathologically as granulomas or cysts. Histologically, such lesions consist mainly of granulation tissue associated with angiogenesis, fibroblasts, connective tissue fibers, and inflammatory cells. Foreign material, cholesterol clefts, and stimulated strands of epithelium also may be present. The stimulated epithelium can form into a stratified, squamous epithelium–lined cystic cavity. These periradicular lesions (granulomas and cysts) are inflammatory lesions that develop in response to irritation caused by intraradicular and extraradicular microorganisms associated with the root canal system or by foreign materials forced into the periradicular tissues.
An important aspect of periradicular surgery is the removal of diseased tissue associated with the root apex. Because a large portion of this tissue is reactionary, the focus of surgical root canal treatment is removal of the irritant or diseased tissues. Histologically, an inflammatory periradicular lesion is similar to healing granulation tissue. If the irritant can be readily identified and successfully eliminated, it is not always necessary to completely curette all the inflamed periradicular tissues during surgery. This is especially true when complete removal might result in injury to neural or vascular tissues. In addition to removing diseased tissue, periradicular curettage provides visibility and accessibility to facilitate treatment of the apical root canal system or removal of foreign materials in the periradicular tissues.
The need for a histopathologic assessment of all tissues removed from the body cannot be overstated. Although only a small percentage of periradicular lesions are associated with pathoses other than a periradicular cyst or granuloma, all lesions must be diagnosed definitively because of the potential gravity of the few rare diseases associated with periradicular lesions.
The technical aspect of removing soft tissue from the bony crypt varies among surgeons and clinical settings. Various bone and periodontal curettes are available for this purpose, and no one instrument suffices for all cases. Regardless of the instrument selected, the basic principles are the same. A sharp instrument is always preferable to a blunt instrument. The soft-tissue lesion first should be peeled away from the osseous crypt, starting at the lateral borders. This can be accomplished efficiently by using the curette with the concave surface facing the internal wall of the osseous crypt. Once the soft-tissue lesion has been separated from the osseous crypt to the point where the crypt changes its convexity, the curette can be used in a scraping manner to remove the remainder of the lesion from the medial wall of the osseous defect.
Localized Hemostasis
Localized hemostasis during periradicular surgery is essential to successful management of the resected root end. Appropriate hemostasis during surgery minimizes surgical time, surgical blood loss, and postoperative hemorrhage and swelling. The hemostatic agents used during endodontic surgery are intended to control bleeding from small blood vessels or capillaries. Localized hemorrhage control not only enhances visibility and assessment of the root structure, but it also ensures the appropriate environment for placement of the current root-end filling materials and minimizes root-end filling contamination.
Many hemostatic agents have been advocated for use during surgery, and the action of these agents, their ability to control bleeding, and their effect on healing vary considerably. They generally aid coagulation by inducing rapid development of an occlusive clot, either by exerting a physical tamponade action or by enhancing the clotting mechanism and vasoconstriction (or both). No one local hemostatic agent is ideal; each has disadvantages. Therefore, further investigation is indicated to find the ideal local hemostatic agent.
Preoperative Considerations
A thorough review of the patient’s body systems and medical history increases the likelihood of detecting an undiagnosed condition that might affect hemostasis during periradicular surgery. Review of the patient’s medications, both prescribed and over-the-counter (OTC) drugs, is essential. Many OTC drugs can affect the clotting mechanism. The patient’s vital signs (i.e., blood pressure, heart rate, and respiratory rate) should be assessed. Vital signs also can be used to monitor anxious patients. An increase in blood pressure and heart rate above a patient’s known normal values indicates increased stress or poorly controlled hypertension. Easing the patient’s anxiety before surgery reduces the possible hemostatic-potentiating effect of elevated cardiac output during surgery. Anxiety and stress can be alleviated with planning, sedation, and profound local anesthesia.
Local Hemostatic Agents
Collagen-Based Materials
Various collagen-based hemostatic agents are available for use as local hemostatic agents. The principal differences are in the microstructure and density of the collagen. Collagen can act as a mild allergen, but the problem of allergenization and unwanted tissue reaction does not occur when highly purified animal collagen is used. The mechanisms by which collagen products help achieve hemostasis involves stimulation of platelet adhesion, platelet aggregation and release reaction, activation of factor XII (Hageman factor), and mechanical tamponade by the structure that forms at the collagen-blood wound interface. Collagen shows minimal interference in the wound healing process, with a limited foreign body reaction. It does not increase the incidence of infection and only slightly delays early bone repair. Osseous regeneration in the presence of collagen typically proceeds uneventfully, without a foreign body reaction.
Collagen-based materials can be difficult to apply to the bony crypt because they adhere to wet surfaces, particularly instruments and gloves. Several collagen-based products are commercially available. They include CollaCote (Integra Life Sciences, Plainsboro, NJ) ( Fig. 9-34, A ), CollaStat (American Medical Products Corp, Eatontown, NJ), Hemocollagene (Septodont, United Kingdom) and Instat (Ethicon, Somerville, NJ). These materials act in essentially similar ways, and the surgical area undergoes a similar healing pattern. Overall, studies of wound healing with collagen-based hemostatic agents have shown favorable results.

Surgicel
Surgicel (Ethicon) is a chemically sterilized material prepared through oxidation of regenerated alpha cellulose (oxycellulose). The basic element of Surgicel is polyanhydroglucuronic acid, which is spun into threads and then woven into gauze. Surgicel has a pH of 3. If the material is maintained in the wound for up to 120 days, a pH this low could retard healing. It is primarily a physical hemostatic agent, which acts as a barrier to blood and then becomes a sticky mass that serves as an artificial coagulum. It does not enhance the clotting cascade through adhesion or aggregation of platelets. Surgicel is retained in the surgical wound, and healing is retarded, with little evidence of resorption of the material at 120 days. Use of Surgicel in extraction sockets resulted in greater postoperative pain compared with a control in a split mouth–designed study.
Gelfoam
Gelfoam (Pharmacia, Peapack, NJ) is a gelatin-based sponge that is water insoluble and biologically resorbable. It stimulates the intrinsic clotting pathway by promoting platelet disintegration and the subsequent release of thromboplastin and thrombin. The initial reaction to Gelfoam in the surgical site is a decrease in the rate of healing. Extraction sockets containing Gelfoam showed a greater inflammatory cell infiltrate, marked reduction in bone ingrowth, and a foreign body reaction at 8 days. However, these effects were transitory and did not impair long-term bone healing.
Bone Wax
Historically, bone wax has been advocated for controlling both hemostasis and debris in the bony crypt during periradicular surgery. It is a nonabsorbable product composed of 88% beeswax and 12% isopropyl palmitate. Healing with bone wax is best described as poor. The bony crypt typically contains fibrous connective tissue and has no bony or hematopoietic tissue. Bone wax retards bone healing and predisposes the surgical site to infection by producing a chronic inflammatory foreign body reaction and impairing the clearance of bacteria. The use of bone wax can no longer be recommended because it impairs healing and several good alternatives are available.
Ferric Sulfate
Ferric sulfate (Cut-Trol, Ichthys Enterprises, Mobile, AL), a necrotizing agent with an extremely low pH, is one of the few products investigated for use in periradicular surgery. In studies using a rabbit model, Lemon and colleagues and Jeansonne and colleagues reported hemostatic control for 5 minutes and near normal healing with only a mild foreign body reaction, provided the surgical wound was adequately curetted and irrigated with saline. Failure to remove ferric sulfate from the surgical wound site resulted in severely impaired healing, a foreign body–type reaction, and, in some cases, abscess formation. The possibility of acute inflammation and necrosis of the surrounding soft tissue with careless use of this solution should not be underestimated. A similar product, Monsel’s solution (ferric subsulfate), has been used to control local hemostasis in dermatologic procedures. However, the popularity of this solution has declined because application to wound sites has resulted in tissue necrosis for up to 2 weeks, differences in the degree of epidermal maturation, and tattoo formation.
Calcium Sulfate
Calcium sulfate has been used as a substitute bone graft material to fill bone defects since the late 1800s. The presence of calcium sulfate in an osseous wound does not inhibit bone formation. It is gradually removed from the site of implantation regardless of whether new bone has formed. Use of calcium sulfate during periradicular surgery does not significantly affect healing, and deposition of cementum and osseous healing proceed normally. As a hemostatic agent, calcium sulfate acts as a physical barrier. The material is placed in the bony crypt, allowed to set, and then partly carved away to allow access to the root end. The remaining material lines the crypt walls, preventing bleeding. When the root-end filling has been placed and all extraneous root-end filling material removed, the residual calcium sulfate can be removed or left in situ.
Epinephrine Pellets
Epinephrine, a sympathomimetic-amine vasoconstrictor, is frequently used to control hemorrhage during oral surgery. All granulation tissue should be removed from the root apex area prior to placement of the epinephrine pellet to assure direct contact with bone. Vasoconstrictive amines exert their effects by binding to and interacting with adrenergic receptors in various body tissues. When epinephrine is bound to alpha 1 – and alpha 2 -adrenergic receptors, a powerful vasoconstricting effect results. Racemic epinephrine cotton pellets (Racellet #3; Pascal Co., Bellevue, WA) contain an average of 0.55 mg of racemic epinephrine hydrochloride per pellet, half of which is the pharmacologically active L-form. Racellets (see Fig. 9-31, B ) provide good localized hemostasis in periradicular surgery.
Two concerns arise with the use of Racellets in the surgical site: the cardiovascular impact of the additional epinephrine and the retention of cotton fibers in the wound, resulting in impaired wound healing. A study by Vickers and coworkers examined the cardiovascular effects of epinephrine pellets and concluded that no evidence existed of cardiovascular changes (blood pressure and pulse) compared with saline-saturated pellet controls. These authors hypothesized that the vasoconstrictive effect on the capillaries is localized and immediate and that little or no systemic uptake of epinephrine occurred.
Although the cardiovascular effect appears to be of little concern, retention of cotton fibers in the surgical site could result in inflammation and impaired wound healing. Therefore, due diligence on the part of the surgeon is paramount when epinephrine pellets are used. Each pellet applied during surgery must be accounted for, and the bony crypt should be lightly curetted to remove any embedded cotton fibers before the wound is closed. The retention of cotton fibers in the crypt can be eliminated by substituting CollaCote saturated with 10 drops of 2.25% racepinephrine inhalation solution. As previously noted, CollaCote is biocompatible and does not interfere with wound healing.
Cautery/Electrosurgery
Cautery stops the flow of blood through coagulation of blood and tissue protein, leaving an eschar that the body attempts to slough. The effect of cautery in the bony crypt during periradicular surgery has not been studied to date. However, the effect of electrosurgery on alveolar bone has been studied in periodontal surgery. Tissue destruction was greater in areas exposed to electrosurgery and healing was delayed compared with surgical sites not exposed to electrosurgery. Twelve hours after surgery, a more extensive inflammatory reaction and greater destruction of periosteum were noted with electrosurgery. At 24 hours, many empty lacunae were observed in the bone associated with electrosurgery, and this necrosis was even more extensive by 48 hours. At 96 hours the electrosurgical connective tissue wounds were still lined by coagulum, whereas the scalpel wound was beginning to repair. The detrimental effect of applying heat to bone is proportional to both temperature and the duration of application.
Management of the Root End
Management of the resected root end during periradicular surgery is critical to the overall success of a case. The aim of surgery should be to create an environment conducive to regeneration of the periodontium—that is, healing and regeneration of the alveolar bone, periodontal ligament, and cementum overlying the root end and root-end filling material. The key to regeneration is the presence of appropriate inducible cell types, growth factors, and specific substances necessary for mineralization. Failure to create an environment conducive to this process results in tissue repair rather than regeneration and possibly less than ideal healing.
Determination of the Need for Root-End Resection and Filling
The basis for periradicular surgery is twofold. The first objective is to remove the etiologic factor; the second is to prevent recontamination of the periradicular tissues once the etiologic agent has been removed.
Etiologic factors typically can be categorized as intraradicular or extraradicular bacteria, intraradicular or extraradicular chemical substances, or extraradicular physical factors. The etiology cannot always be determined with complete certainty; frequently, a number of factors are involved. However, most cases involve some form of bacterial participation (e.g., bacteria within apical ramifications). The only definitive means of eradicating such an irritant is physical removal through root-end resection. The rationale for root-end resection in such cases is to establish access to and remove the diseased tissues. This ensures that the optimum environment for wound healing is established.
As mentioned, the second objective of periradicular surgery is to prevent recontamination of the tissues after removal of the etiologic agent. It follows, then, that if the remainder of the canal system cannot be verified to be irritant free, a root-end filling should be placed to seal any remaining irritants within the canal system, thus preventing recontamination of the periradicular tissues.
Root-End Resection
Two main principles dictate the extent of the root-end resection. First, the cause (or causes) of an ongoing disease process must be removed; this includes removal of the diseased tissue and, when indicated, reduction of an apically fenestrated root. Second, adequate room must be provided for inspection and management of the root end.
The anatomy of each tooth root is complex (see Chapter 5 ). The surgeon must understand the anatomy of the apical third of the root to determine the extent of a root-end resection. Approximately 75% of teeth have canal aberrations (e.g., accessory or lateral canals) in the apical 3 mm of the tooth. An apical resection of approximately 3 mm should include most accessory and lateral canals and thus eliminate most residual microorganisms and irritants ( Fig. 9-35 ). When roots with more than one main canal are resected, isthmus tissue may be present, and the preparation should be modified to include the isthmus area ( Fig. 9-36 ).
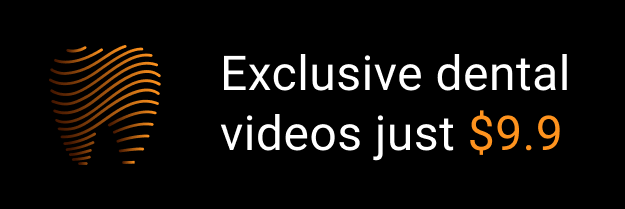