‘Respiratory disease’ describes a broad range of pathological conditions that affect the respiratory system including chronic obstructive pulmonary disease (COPD), asthma, infections such as pneumonia, lung cancer and others. With global deaths in 2016 attributable to COPD at 2.93 million and lung cancer at 1.70 million alone, respiratory diseases taken together are amongst the leading causes of deaths worldwide1.
Amongst the various links between periodontitis and systemic diseases, there is a potential association with respiratory disease (Table 6-1). A number of plausible mechanisms exist to explain how periodontitis may be associated with certain respiratory diseases:
Table 6-1 In vivo human studies investigating the association between periodontitis and specific respiratory diseases
Respiratory disease | Overall outcome (summary) | Studies | Study design |
---|---|---|---|
COPD | Low-level evidence provides some corroboration of an association between periodontitis and COPD. There is insufficient evidence to suggest periodontal interventions would alter COPD pathogenesis or progression. | Scannapieco et al2, Azarpazhooh and Leake3, Zeng et al4, Shi et al5 | Meta-analysis |
Zhou et al6 | Interventional | ||
Hayes et al7, Russell et al8, Kowalski et al9, Wang et al10, Deo et al11, Prasanna12, Si et al13, Tan et al14, Bomble et al14a | Case-control | ||
Zhou et al15, Scannapieco et al16, Garcia et al17, Scannapieco et al18, Hyman and Reid19, Katancik et al20, Leuckfeld et al21, Harland et al22, Winning et al22a | Cross- sectional | ||
Takeuchi et al23 | Cohort | ||
COPD (alpha-1 anti-trypsin deficiency) | Insufficient available evidence to draw conclusion. | Peterson and Marsh24, Scott et al25, Wallin-Bengtsson et al26, Hobbins et al27 | Case-control |
Sapey et al27a | Cross- sectional | ||
Asthma | Studies investigating a relationship between periodontitis and asthma have provided inconsistent results. There may be some evidence for an association; however, a lack of longitudinal and interventional type studies prevents any conclusion on the significance of reported associations to be made. | Moraschini et al28 | Meta-analysis |
Hyyppa et al29, Hyyppa30, Bjerkeborn et al31, Laurikainen and Kauusisto32, McDerra et al33, Shulman et al34, Shashikiran et al35, Yaghobee et al36, Mehta et al37, Stensson et al38, Ferrazzano et al39, Gomez-Filho et al40, Lee et al41, Khassawneh et al42, Soledade-Marques et al43 | Case-control | ||
Friedrich et al44, Gómez Real et al45, Rivera et al46, Shen et al47 | Cross- sectional | ||
Pneumonia | RCTs of interventions that improve oral hygiene have been shown to have positive effects on the prevention of pneumonias. However, there is insufficient evidence to directly associate periodontitis with pneumonias. | Scannapieco et al2, Azarpazhooh and Leake3, Sjogren et al48, Labeau et al49, Kaneoka et al50, El-Rabbany et al51, Jerônimo et al51a | Meta-analysis |
Pugin et al52, Yoneyama et al53, DeRiso et al54, Fourrier et al55, Genuit et al56, Bergmans et al57, Houston et al58, Adachi et al59, Yoneyama et al60, Fourrier et al61, Bopp et al62, Koeman et al63, Segers et al64, Seguin et al65, Tantipong et al66, Bellissimo-Rodrigues et al67, Panchabhai et al68, Scannapieco et al69, Pobo et al70, Ćabov et al71, Berry et al72, Grap et al73, Jacomo et al74, Yao et al75, Kusahara et al76, Meinberg et al77, Özçaka et al78, Sebastian et al79, Lorente et al80 | Interventional (oral hygiene) | ||
Gomes-Filho et al81 | Case-control (periodontitis) | ||
Iwasaki et al82 | Cohort (periodontitis) |
COPD = chronic obstructive pulmonary disease; RCT = randomised controlled trial.
● The role of the neutrophil is central in the pathophysiology of both periodontitis and certain respiratory diseases such as COPD. Features such as dysfunctional neutrophil behaviours, sustained neutrophilic inflammation, and connective tissue loss could represent a shared pathophysiology83.
● Periodontitis represents a source of low-grade chronic inflammation that contributes to the cumulative systemic inflammatory burden84. Systemic inflammation has been shown to be related to longitudinal decline in spirometric lung volumes85,86.
● The anatomical continuity between the lungs and the oral cavity represents a potential gateway for pathogen exchange. Aspiration of oral pathogens into the lung, or colonisation of dental plaque by respiratory pathogens followed by aspiration, may represent possible alternative pathways explaining any association87.
Despite the biological plausibility of the outlined mechanisms, evidence evaluating the relationship between periodontitis and respiratory disease is currently limited88. Cigarette smoking is a common risk factor for periodontitis89 and many respiratory diseases, and caution should therefore be exercised when exploring potential associations. It should also be mentioned that both periodontitis and certain respiratory diseases occur within a similar spectrum of comorbid diseases.
The aim of this chapter is to review the clinical epidemiological evidence for association between periodontitis and specific respiratory diseases and provide an overview of the plausibility of the mechanisms. The association of periodontitis with lung cancer is discussed in Chapter 8 ‘Periodontitis and malignancy’.
6.2.1 COPD
6.2.1.1 Definition, prevalence and diagnosis
COPD is a common debilitating lung disease associated with local and systemic inflammation. COPD as a disease name emphasises the chronicity of the condition/s and the defining diagnostic parameter (fixed airflow obstruction). Currently, COPD is the fourth leading cause of death worldwide (over 3 million recorded deaths in 2012)90–92, but the global impact is expected to rise further in the next few decades, as an aging population will lead to higher levels of long-term exposure to risk factors for disease development93.
A recent meta-analysis estimated the global prevalence of COPD to be 7.6%94, but prevalence estimates vary significantly between populations95 due to differences in risk factor exposure, and differences in the rigour of diagnostic criteria used to define COPD across studies, which introduce the potential for misclassification bias94,96. COPD carries a significant economic burden, estimated at 6% of the European Union health care budget (costing 38.6 billion euros per annum)97. COPD symptoms typically progress slowly over time97,98, but many COPD sufferers experience acute periods where their symptoms flare up, termed exacerbations, which are usually triggered by infective organisms or environmental pollutants97. Acute exacerbations of COPD are significant medical events, often resulting in hospitalisation and occasionally, death99. COPD is also associated with a high burden of comorbidities, including a greater prevalence of cardiovascular disease, diabetes and osteoporosis than expected when considering shared socio-economic and lifestyle factors including cigarette smoke exposure, low physical activity and poverty100. Periodontitis is one such comorbidity frequently present in patients with COPD83.
There is no single diagnostic test for COPD. Making a diagnosis relies on clinical judgement based on a combination of history, physical examination and confirmation of the presence of airflow obstruction using spirometry. According to the most recent Global initiative for chronic Obstructive Lung Disease (GOLD)92 strategy for diagnosis and management of COPD, a diagnosis of COPD should be considered in any patient with symptoms of dyspnea, chronic cough or sputum production, and a history of risk factors for the disease, particularly tobacco smokin101.
Spirometry is the only accurate method of measuring the airflow obstruction in patients with COPD. A spirometer is a device for measuring timed expired and inspired volumes; hence, it indicates how quickly and effectively the lungs can be emptied and filled (Fig 6-1). Forced expiratory volume (FEV) tends to be the measure of choice for diagnosing COPD. It is reproducible and objective with well-defined normal ranges. It can be measured quickly and easily at all stages of disease. There is strong epidemiological evidence to indicate that reduced FEV in 1 second (FEV1) is a marker of cardiovascular mortality, independent of age, gender and smoking history102. The value of FEV1 is normally represented as a percentage of an individual’s predicted FEV1, which is calculated against a reference value, taking into account age, gender, height and race by applying the widely used equation of the European Community for Steel and Coal103. A residual ratio of the FEV1 to the forced vital capacity (FVC) of 0.7 or less is widely used to define airflow limitation in both clinical and epidemiological studies104. This is then further sub-classified based on the % predicted FEV1 to give a measure of severity:
Stage 1 – mild: ≥ 80% predicted FEV1 (symptoms should be present to diagnose COPD in people with mild airflow obstruction)
Stage 2 – moderate: 50% to 79% predicted FEV1
Stage 3 – severe: 30% to 49% predicted FEV1
Stage 4 – very severe: < 30% predicted FEV1.
Fig 6-1 Depiction of lung volumes and capacities. Common measures recorded as part of spirometry investigations include: forced vital capacity (FVC), forced expiratory volume in 1 second (FEV1) and forced expiratory ratio (FER)%. FVC is the volume from full inspiration to forced maximal expiration. FVC is reduced in restrictive disease and also in obstructive disease if air-trapping occurs. FEV1 is the volume of air expelled in the first second of a forced expiration. FEV1 is reduced in both obstructive and restrictive disease. FER% is the percentage of FVC expelled in the first second of a forced expiration. FER% is reduced in obstructive disease and remains normal (or even elevated) in restrictive disease.
6.2.1.2 Clinical phenotypes of COPD
It is recognised that COPD is a heterogenous disease having both pulmonary and extrapulmonary features105. Features of COPD include damage to the small conducting airways106, epithelial damage and impaired ciliary function in the larger airways, mucous gland hyperplasia and mucus secretion, and permanent dilation of airways typical of bronchiectasis. All of these features can also be replicated by the local effects of serine proteinases107. These changes can lead to bacterial colonisation of the airways increasing local lung inflammation due to activation of the innate immune system. Lung damage can be enhanced by recurrent exacerbations due to chest infections. At present there is no consensus on the number and definition of the different COPD phenotypes. However, at least three COPD phenotypes have been validated:
● Alpha-1 antitrypsin deficiency (AATD) is a genetic condition characterised by lower levels of alpha-1 antitrypsin (AAT), an inhibitor of proteolytic enzymes. It predisposes patients to COPD and liver disease, and has been identified as a specific phenotype that may respond positively to augmentation therapy108.
● Emphysema, characterised by upper-lobe emphysema, dyspnea and poor exercise capacity, is associated with severe airflow limitation109. Emphysema, however, may be present before the spirometric criteria that define COPD110.
● Frequent exacerbators (more than two episodes per year), have poor quality of life, increased mortality and a greater decline in lung function111.
In summary, COPD is a group of pathological disorders with a common defining physiological measure. Inflammation is central to the pathophysiology, with pro-inflammatory cytokines detectable, and the condition is influenced by the local effects of smoking and bacterial colonisation. The accompanying destruction of lung architecture has long been related to the neutrophilic component of the inflammation.
6.2.1.3 Cause
The cause of COPD is predominantly cigarette smoking, although in less developed countries indoor air pollution due to burning of biomass fuels is recognised as a major cause, especially in females112. Other toxic gases, infections in childhood and poor lung growth have also been implicated, as have some genetic factors. Nevertheless, exposure to these hazards does not always lead to COPD, thereby underlining the general concept that it is caused by a gene/environment interaction in susceptible individuals resulting in dysregulated inflammation in the lung, causing structural damage.
6.2.1.4 Pathophysiology
Until the 1960s there was very little understanding of the pathophysiological processes that lead to the development of COPD. In 1963, Laurell and Eriksson113 published a series of case reports of individuals who had a plasma deficiency of AAT and the tendency to develop very severe early onset emphysema, a significant though not ubiquitous feature of COPD. Since AAT was the major plasma inhibitor of serine proteinases, it was argued that a serine proteinase normally controlled by AAT was directly responsible for the structural lung damage that was the feature of COPD. Trypsin itself was an obvious candidate, although its release into the lung was thought to be highly unlikely from its pancreatic source. A year later, Gross and colleagues, whilst trying to develop an animal model of lung fibrosis, instilled papain, a pineapple enzyme, into the lung and surprisingly found changes consistent with emphysema114. This supported the importance of local enzyme release to instigate the lung structural changes of emphysema.
By the early 1970s, the serine proteinase neutrophil elastase (NE), produced by neutrophils and with elastolytic properties, was shown to produce emphysema-like changes in the lungs of animal models115. The importance of degradation of lung elastin116 and impaired elastin regeneration117 led to a uniform hypothesis that lung elastin degraded by NE was a central process in the development of emphysema and hence COPD. Since AAT was the key plasma elastase inhibitor and an important lung inhibitor of NE, the relationship to AATD was understandable.
However, most patients with COPD have normal plasma concentrations of AAT and since this protein enters the lung mainly by simple diffusion118 the lessons from AATD seemed insightful but not critically important to COPD pathogenesis. Subsequent biochemical studies showed that AAT function could be impaired by binding of free oxygen radicals to the active site methionine, which is critical for its function and specificity119. Since cigarette smoke, the major cause of COPD, contains free radicals in abundance, it seemed logical that local inactivation of AAT produced a functional deficiency, hence being consistent with the same mechanism in the lung as seen in the genetic deficiency of AAT. Thereafter, two publications seemed to finalise the process. Firstly, Gadek et al120 lavaged the lungs of smokers and found a reduction in AAT function. Secondly, Carp et al121 purified lung AAT and identified the presence of oxidised methionine. These two observations led to the long-held belief of a central role of oxidation of AAT in inflamed tissues resulting in a failure to control NE and in excess tissue destruction.
However, this simple biochemical interaction with cigarette smoke failed to explain why most smokers failed to develop COPD. The concept was further questioned by other studies that demonstrated that AAT function was not reduced in the lungs of smokers compared to non-smokers122–124, and that any inactivation was likely due to complexing with serine proteinases or cleavage of the carboxyl terminus by non-serine proteinases, with little evidence of oxidation of the active site methionine125. In addition, other locally produced inhibitors of NE were found to be present in the lung126, even questioning the role of AAT in the disease process. Nevertheless, the central role of oxidative inactivation of AAT in inflammatory tissue damage remains widely quoted, despite this clearly conflicting evidence. Moreover, AAT deficiency is regarded as a specific but rare form of COPD.
The pathophysiological processes in COPD have become more complex with time. Proteinase 3 (Pr3), another neutrophil serine proteinase, has the ability to cause emphysema in animal models127, as does the cysteine proteinase, cathepsin B (Cat B)128. Matrix metalloproteinases (MMPs) also have the ability to digest elastin, suggesting tissue-degrading activity in COPD. Therefore, a cascade of events relating to proteinase inhibitor activation and inactivation seems plausible: NE can activate the proenzymes of Cat B and MMPs, whilst inactivating their cognate inhibitors, enhancing their local activity106. At the same time, Cat B and MMPs can inactivate AAT by proteolytic cleavage near the inhibitory site, facilitating the local activity of NE and leading to a chicken and egg conundrum, as outlined in Fig 6-2. Nevertheless, NE and Pr3 are still likely to be the final common pathway. COPD is a neutrophilic inflammatory disease and these two enzymes are constitutively expressed and stored in the azurophil granule of the mature cell. The concentrations of both enzymes in the azurophil granule are two orders of magnitude higher than the likely prevailing concentrations of AAT and hence when released during migration into the tissues there will be an obligate area of retained enzyme activity around the neutrophil130. Indeed, the resulting tissue damage may be necessary to facilitate cell migration, and the role of AAT would mainly be to limit the subsequent damage as the enzymes diffuse and their concentrations fall.
Fig 6-2 (1) The conventional cause of lung tissue damage has been attributed to neutrophil elastase (NE). (2) Alpha-1 antitrypsin (AAT) is a key regulator of plasma and lung NE and is supported by the locally produced lung inhibitor secretory leucoprotease inhibitor (SLPI). (3) NE also activates the pro enzymes of matrix metalloproteinases (MMPs) and the cysteine protease cathepsin B, both of which have also been implicated in tissue damage in chronic obstructive pulmonary disease. (4) These enzymes are capable of inactivating AAT and SLPI, thereby facilitating NE activity. (5) Cathepsin B is locally controlled by the inhibitor cystatin C and the MMPs are controlled by tissue inhibitors of metalloproteinases (TIMPs), and in turn both of these inhibitors can be inactivated by NE leading to a cycle and cascade of protease that facilitate the overall function of NE. Modified from Stockley129.
If COPD neutrophils have an inherent abnormality associated with excess degranulation (see Section 6.3.1.1 ‘Neutrophils in health’), the release of excess neutrophil serine proteinase will initially overwhelm local inhibitors. This is likely the direct cause of tissue degradation, especially of the interstitial connective tissue that is essential to maintain normal lung structure. Activation of other proteinases and inactivation of their inhibitors in addition to stimulation of cytokine release by NE are also likely to contribute to producing a closed amplification circle. A summary of the role of the neutrophil-mediated tissue damaged in COPD is depicted in Fig 6-3. The direct role of neutrophils is further discussed in Section 6.3 ‘Cellular and molecular mechanisms’.
Fig 6-3 (1) Local exposure of the lung to irritants such as cigarette smoking stimulate resident cells including airway epithelium and resident macrophages to release a variety of pro-inflammatory cytokines including neutrophil chemo-attractants (such as interleukin-8 [IL-8] and leukotriene B4 [LTB4]). (2) This results in tissue degradation to facilitate neutrophil migration especially in alpha-1 antitrypsin (AAT) deficiency (pathway B). (3) This releases degradation products of lung connective tissue (CT) that can in themselves drive inflammation and further neutrophil recruitment. (4) Airway neutrophils release more proteases, which can in turn activate airway macrophages and epithelial cells to release more pro-inflammatory cytokines, amplifying the process. (5) Activation of local tissue macrophages may also contribute to local tissue damage through the release of matrix metalloproteinases (MMPs). Modified from Stockley131.
6.2.1.5 Epidemiological evidence: periodontitis and COPD
A possible link between periodontitis and COPD was first suggested in several epidemiological analyses of National Health and Nutrition Examination Survey (NHANES) data16,18, and data from the Veterans Administration Dental Longitudinal Study (VADLS)51. There appeared to be a dose effect, whereby more severe periodontitis was associated with greater loss of lung function. A later analysis of the VADLS data, after over 30 years follow-up, validated the association even after stratification for smoking17, suggesting that periodontitis is a co-factor for COPD. Following analysis of NHANES III, Hyman and Reid19 found an almost four-fold increase in COPD among current smokers with severe periodontitis (odds ratio [OR] 3.71, 95% confidence interval [CI] 1.74 to 7.89). However, this was the only significant association found and was limited to 1.3% of those studied. It was concluded that adjusting for smoking as a confounder was insufficient and that it should be treated as an effect modifier in any association between periodontitis and COPD19. A study of well-functioning adults aged between 70 and 79 years found an association between periodontitis and obstructive airway disease in former smokers, but not in never-smokers. Gingival Index and loss of attachment were significantly better in participants with normal pulmonary function compared to those with airway obstruction after adjusting for age, race, gender and field centre20. A case-control study from a hospital population in Beijing found that in analyses stratified for smoking there was no significant association between periodontitis and COPD10. Periodontal status was not associated with the frequency of exacerbations in patients with COPD132. A systematic review by Scannapieco et al2 concluded that the associations reported between periodontitis and COPD were preliminary and further studies were needed. A subsequent systematic review by Azarpazhooh and Leake3 concluded that there may be a potential association between periodontitis and COPD. The review identified four studies, two case-control and two cross-sectional studies. In general, there was inconsistency in the definition of periodontitis, with one study using alveolar bone levels, and the others using proxy measures of periodontitis (oral hygiene status). Evidence also exists from studies examining the association between periodontitis and the broader definition of reduced respiratory function at a population level. Holtfreter and colleagues133 carried out a cross-sectional study in a representative German adult population (Study of Health in Pomerania). Periodontal status, assessed by clinical attachment level (CAL), was significantly associated with reduced lung volumes (mobile dynamic and static), and airflow limitation. Similar findings to this study have also been confirmed from a cross-sectional study carried out in a group of middle-aged men in Northern Ireland134. Analysis showed that a doubling in mean CAL equated to a −3.33% (95% CI −4.80 to −1.86) decrease in lung function (% predicted FEV1) after adjustment for all other potential confounding variables. This was confirmed by Tan et al14, who showed that clinical attachment loss was greater in COPD patients than in controls. More recently, Takeuchi et al23 reported on a 5-year prospective study of 900 community-dwelling Japanese adults without COPD. During follow-up, 22 (2.4%) subjects developed COPD. Compared with no/mild periodontitis subjects, a significantly increased risk of COPD occurred among severe periodontitis subjects (relative risk [RR] 3.55, 95% CI 1.18 to 10.67). After adjustment for potential confounders, including smoking intensity, the relationship between severe periodontitis and risk of COPD remained significant (RR 3.51, 95% CI 1.15 to 10.74).
If there are common pathogenic mechanisms shared with COPD and periodontitis, COPD should be more prevalent in patients with periodontitis and vice versa. To date, the majority of epidemiological studies have supported such a relationship, finding an increased burden of one disease in the other condition; however, interpretation of the data requires some caution. To associate the two conditions strongly, studies should apply clear and standard definitions of both diseases, and common risk factors (such as age, smoking history and low socio-economic status) should be taken into account. Sadly, some of the available data have failed to do this83. A meta-analysis of 14 observational studies involving 4000 COPD patients and 23,000 controls found patients with periodontitis had a two-fold risk (95% CI 1.48 to 2.91) of having COPD4. Assessment of the individual studies within this meta-analysis demonstrated that four different assessment criteria had been applied to define periodontitis, and the definition of COPD varied from being self-reported16 to being made using the internationally accepted GOLD criteria19. When disease prevalence was assessed without considering confounding factors, the OR for risk of COPD or periodontitis has been as high as 4.50 (95% CI 1.06 to 18.99)16. When relevant confounders have been considered, the association between COPD and periodontitis is not always apparent19.
Patients with COPD had a particularly increased prevalence of periodontitis if they had required hospitalisation for an acute exacerbation (OR 3.17, 95% CI 2.81 to 3.57) or as the number of emergency room visits increased (OR 5.09, 95% CI 4.53 to 5.72)135. This was also evidenced by a 2018 meta-analysis including 14 studies, mostly case-control studies. This analysis revealed that COPD patients suffer from worse periodontal health status than controls, indicated by deeper periodontal pockets, higher level of clinical attachment loss, worse oral hygiene, and more bleeding on probing as well as fewer remaining teeth. However, due to the low number, moderate quality and large heterogeneity of most of the included studies, the results of this meta-analysis need to interpreted with caution5. In addition, other studies have demonstrated relationships between measures of periodontitis and the physiological severity of COPD11,12,136. On the other hand, smoking has also been reported to be the major risk factor for both periodontitis and COPD19,137,138. For example, a 2018 cross-sectional study of 1474 Japanese men demonstrated that the association between COPD and smoking was stronger for men with periodontitis (OR 2.45, 95% CI 1.37 to 4.37) than for those without periodontitis (OR 1.64, 95% CI 0.91 to 2.94), after adjusting for age, number of present teeth, body mass index, alcohol intake, occupation, hypertension and diabetes22. These results suggest that periodontitis modifies the association between smoking and COPD22. Most of the disparity is likely to reflect the lack of consistency in case definitions, data collection and analysis, as outlined by Hobbins et al83. Even when COPD and periodontitis are well defined and smoking and age accounted for, the relationship may still reflect socio-economic factors that are difficult to fully define, such as poor nutrition, poor oral health, exposure to pollutants and toxins, and greater alcohol consumption.
Currently, there has been one interventional study that tested the theory that the treatment of periodontitis would improve outcomes in COPD. In 2014, Zhou et al6 randomised 60 matched patients with moderate COPD (diagnosed using GOLD criteria139) and periodontitis to either treatments for periodontitis (n = 40) or dental hygiene advice (n = 20). They found a minimal increase in predicted FEV1 of 2% over 2 years in the treatment but not in the control group. However, it is unclear if this change would be sufficient for patients to notice benefit. Moreover, no mechanistic studies were performed to elucidate why improvements in lung function were evident. Interventional studies documenting the effect of periodontal treatment on systemic inflammation have not been conclusive140.
SUMMARY
● In general, studies suggest there is a weak association between periodontitis and COPD. The association of these two diseases may in fact reflect shared common risk factors in their respective aetiologies. Until these shared risk factors are accounted for in appropriately designed studies, a causal relationship cannot be determined.
● Further well-designed studies, including interventional randomised controlled trials (RCTs) and large-scale population-based prospective cohort studies, are required to investigate the possible causal association between periodontitis and COPD.
6.2.2 Asthma
6.2.2.1 Definition and prevalence
Asthma is a chronic inflammatory disease characterised by recurrent attacks of breathlessness and wheezing, which vary in severity and frequency from person to person. It is widely believed to be an autoimmune disorder (see Chapter 11 ‘Periodontitis and autoimmunity’). Symptoms may occur several times in a day or week in affected individuals, and for some people become worse during physical activity or at night. According to World Health Organization (WHO) estimates, 300 million people suffer from asthma and it is the most common chronic disease among children141. Asthma occurs in all countries regardless of level of development; however, 80% of asthma deaths occur in low- and lower-middle income countries.
6.2.2.2 Periodontitis and asthma
An association between asthma and periodontitis has been reported to be related to inflammatory and immunological responses common to both diseases, including immunoglobulin E (IgE)-mediated mechanisms, platelet-activating factor as a mediator in periodontitis and allergic inflammatory reactions142. Cytokines present in periodontal inflammation affect the mucous membranes of airways, and mononuclear cells from periodontitis can produce interleukin (IL)-5 and IL-6. However, studies investigating a relationship between periodontitis and asthma have provided inconsistent results.
6.2.2.3 Epidemiological evidence
A systematic review and meta-analysis, involving 21 studies published between 1979 and 2017, reported significant differences in Plaque and Gingival Indices between asthmatic patients and healthy controls143. However, assessment of the studies examined by the systematic review limits the interpretation of findings. Nine of the studies did not utilise established criteria for the diagnosis of asthma. Similarly, only one of the studies used the recommended standardised case definition of periodontitis144. The majority of the studies focused on gingivitis-related indices, whereas only four studies specifically investigated an association with periodontitis32,39,46,47. This is because many of the studies investigated child cohorts, in which periodontitis rarely develops prior to 15 years of age. Confounding factors such as medication, particularly steroids used to control asthma symptoms, smoking and poor oral hygiene were not accounted for in all studies, increasing the possibility for bias. Other factors such as mouth breathing in those suffering from severe asthma may have led to a positive association between asthma severity and levels of gingival inflammation. Most of the studies included were either case-control or cross-sectional in design. No interventional studies were included in the meta-analysis. Recent studies of note include:
● Rivera et al46 investigated a cohort of 1315 asthmatic individuals (191 with asthma and 1124 without) from the San Juan Overweight Adults Longitudinal Study. Individuals were either overweight or obese, with an age range 40 to 65. Asthma was self-reported, whereas periodontitis was recorded by clinical examination using an accepted case definition144. Adjusting for gender, smoking status, age, body mass index, family history of asthma and income level revealed that the proportion with severe periodontitis was much lower among participants who reported asthma compared to those with no asthma. The findings suggest participants with severe periodontitis were less likely to have asthma, i.e. an inverse relationship. The authors cited the hygiene hypothesis145 as a possible explanation for their findings, where the decline in infectious diseases may be causally linked to an increase in immunological disorders.
However, in contrast to the Rivera et al46 study, four recent investigations found positive associations between periodontitis and asthma:
● Shen et al47 performed a retrospective analysis of National Health Insurance claims data in Taiwan. They identified 19,206 asthmatics newly diagnosed from 2000 through 2010. For each case, four age- and sex-matched individuals without history of asthma and periodontitis were randomly selected from the same population. Both cohorts were followed to the end of 2011, when the overall incidence of periodontitis was 1.18-fold greater (95% CI 1.14 to 1.22) in the asthma cohort than in the control cohort.
● Lee et al41 conducted a study of 5,976 adult participants (89 asthmatic and 5887 non-asthmatic), based on a cross-sectional analysis from the Sixth Korean National Health and Nutrition Examination Survey in 2014. Presence of periodontitis was evaluated by Community Periodontal Index (CPI; WHO), and asthma condition was defined by self-report. Current asthma conditions were five times (OR 5.36, 95% CI 1.27 to 22.68) more likely to be associated with periodontitis after adjustment for sociodemographic and lifestyle covariates.
● Khassawneh et al42 carried out a case-control study of 130 asthma patients and 130 healthy controls in Jordan. Periodontitis was diagnosed by means of periodontal pocket depth and CAL. Binary logistic regression indicated that patients with asthma were more likely to have periodontitis than controls (adjusted OR 2.91, 95% CI 1.39 to 6.11). In this study, the risk of periodontitis (OR 5.27, 95% CI 1.59 to 17.51) and clinical attachment loss ≥ 3 mm were higher in patients on oral corticosteroids compared to inhaled corticosteroids42.
● Soledade-Marques et al43 performed a similar case-control study in a Brazilian population, also with 130 participants per group. Severe asthma diagnosis was based on Global Initiative for Asthma criteria. When confounders were considered, the association between exposure to periodontitis and severe asthma was significant (OR 3.18, 95% CI 1.8 to 5.63). Individuals with periodontitis had about a threefold increased risk of severe asthma compared to those without periodontitis. The frequency of periodontitis in participants with severe asthma was greater than that of those without asthma (46.6% vs. 22.3%)43.
SUMMARY
● In general, the majority of studies suggest a weak association between periodontitis and asthma. However, conflicting results and a lack of longitudinal or interventional studies prevent a conclusive statement regarding an association.
● Further well-designed studies, including interventional RCTs and large-scale population-based prospective cohort studies, are required to investigate the possible association between periodontitis and asthma.
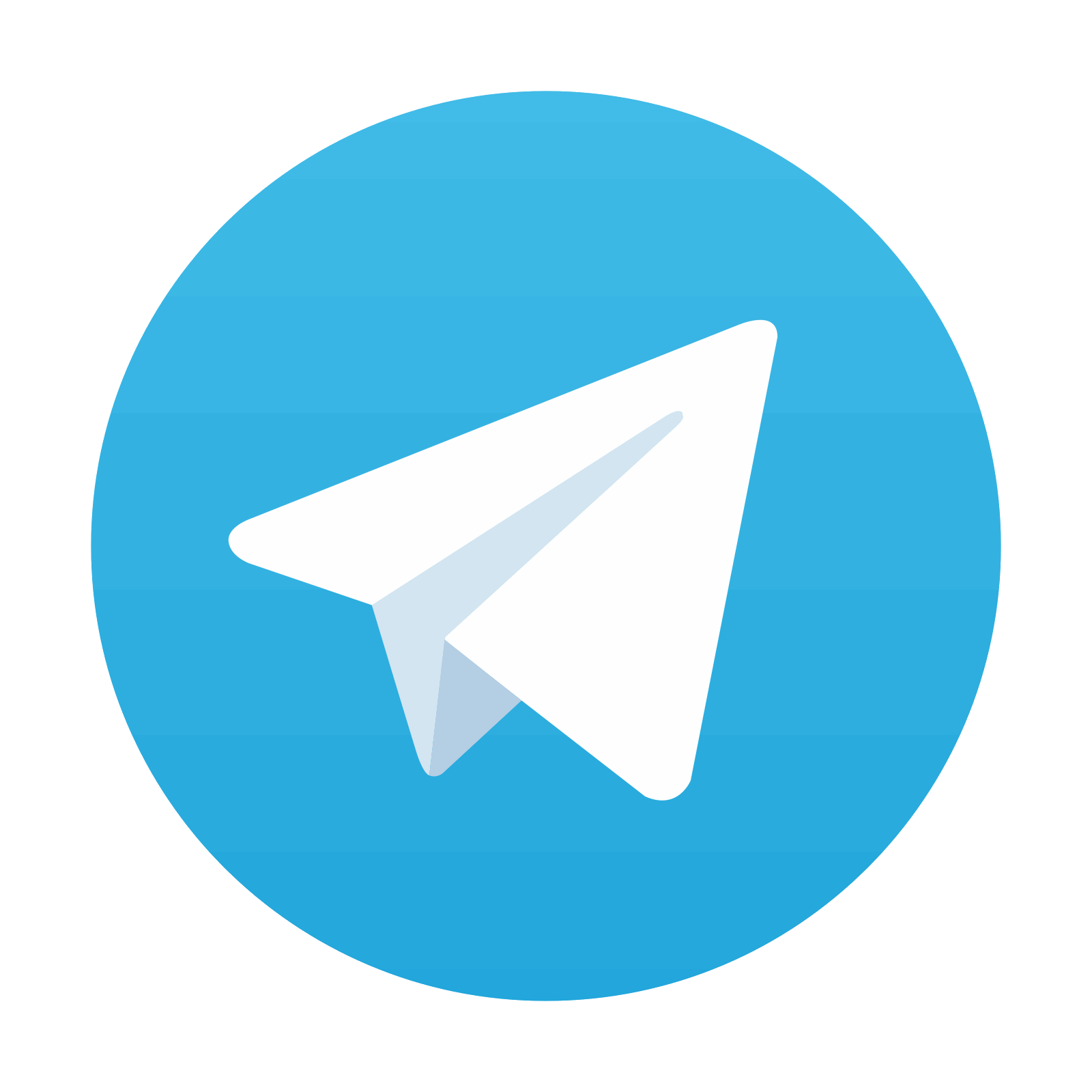
Stay updated, free dental videos. Join our Telegram channel

VIDEdental - Online dental courses
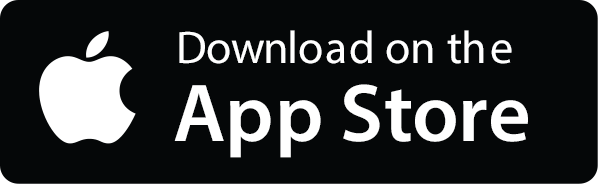
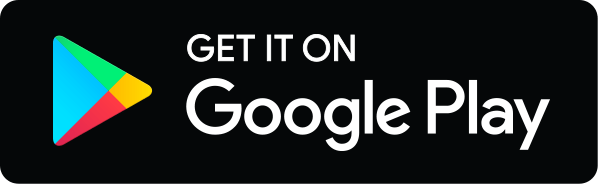