2
Osseointegration
Osseointegration (OI) was first defined at the light microscopic level as a ‘direct structural and functional connection between ordered living bone and the surface of a load carrying implant’ (Branemark 1983). The phenomenon involves a complex interaction between the implant, bone and tissue interface (Figure 2.1). The process involves two stages with an initial biomechanical interlocking between the implant body and alveolar bone, called primary stability, followed by the second stage which involves the biological fixation of the implant through bone remodelling and apposition, called secondary stability, with each stage being influenced by various factors and steps being followed during the placement of the implant (Figure 2.2). Primary implant stability plays an essential role in successful osseointegration and is dependent on the implant geometry, the bone quality and quantity as well as the site preparation technique.

Figure 2.1 Bone to implant contact in osseointegration.

Figure 2.2 Primary (mechanical) stability vs secondary (biological) stability.
Concept
The original concept of OI was redefined at the clinical level by Zarb and Albrektsson (1983) as a ‘time dependent healing process whereby clinically asymptomatic rigid fixation of alloplastic materials is achieved and maintained in bone during functional loading’. The histologic appearance resembled a functional ankylosis with no intervening fibrous or connective tissue between bone and implant surface (Figure 2.3). The first implants, known as ‘Branemark Implants’, were made of pure machined titanium surfaces and had to be covered over by the gum flap after surgical placement for at least six months to allow the screw to integrate into the jaw bone. Today with an improved understanding of the concept and changes in implant screw designs and surface modifications, the healing times have reduced with implants being restored at the same time as placement with healing periods of 0–8 weeks. Figure 2.4 shows the different healing time lines for dental implants and their restorations. The original clinical definition of osseointegration thus needs updating with removal of the word ‘time dependent’ as a fixed period of healing before loading is no longer an absolute requirement.

Figure 2.3 Osseointegration showing the close interface of the implant surface to bone with no intervening soft tissue.

Figure 2.4 Healing time lines for implant placement and restoration.
Factors Affecting Osseointegration
Factors affecting osseointegration can be divided into implant related and environment related (Table 2.1).
Table 2.1 Factors Affecting Osseointegration.
Implant-Related Mechanical Stability = Primary Stability (Initial Period) |
Environment (Host)-Related Biological Stability = Secondary Stability |
---|---|
Materials (Biocompatiblity)
|
Status of the Host Bed
|
Design
|
Surgical Site Preparation
|
Surface
|
Healing Periods Influenced by above and need for augmentation
|
Diameter and Length
|
Loading Conditions
|
Influenced by Operator Related Knowledge, Skill and Competence |
- Implant-related factors:
These factors primarily affect the mechanical interlocking of the implant and are thus fundamental to facilitating OI. They include the material of which the implant is made (biocompatibility), the design features of the implant and the macroscopic and microscopic surface features of the implant. Progressive changes in these features have enabled reduced healing periods as a result of the improved primary stability at the time of implant placement.
- Implant Materials
The material of which the implant is made does not directly influence the mechanical stability of the implant but ensures biocompatibility.
- Commercially pure titanium (cpTI):
This material has excellent biocompatibility, and its bioactivity is related to the immediate formation of a stable and inert oxide layer on its outer surface when exposed to air. It is classified into grades 1–4 depending on the purity and processing oxygen content with Grade 1 being the most pure with the lowest oxygen content (0.18%) and Grade 4 being the least pure with the highest oxygen content (0.4%). The different grades contain varying amounts of contaminants such as iron for corrosion resistance, aluminium for increased strength and reduced density and vanadium which scavenges the aluminium thus imparting corrosion resistance. Grade 4 cpTI has the highest strength, highest passivity and a modulus of elasticity comparable to bone and is therefore the material of choice for manufacturing dental implants. Its key disadvantages are the low wear resistance and grey metal shine through in patients with thin gingival tissues contributing to compromised aesthetics.
- Titanium alloys:
The alloying of cpTI enhances the strength and wear resistance of the material.
- Titanium, Aluminium and Vanadium Alloy (Ti6Al4V):
This alloy is mainly used for dental implants and contains 6% aluminium, 4% vanadium, up to 0.25% iron and 0.2% oxygen with the rest being titanium. The material has excellent fatigue properties and corrosion resistance with a low elastic modulus leading to a ‘stress shielding’ effect due to the mismatch between the implant material and surrounding bone. The alloy releases small amounts of aluminium and vanadium ions and can be associated with allergic reactions, cytotoxic effects and neurological disorders. Implant systems made of titanium alloys usually tend to be slightly cheaper than those using cpTI.
- Titanium-Zirconium Alloy (Ti-Zr):
This alloy has been shown to be biocompatible, bioactive and mechanically stable and has comparable properties to cpTi with a reported tendency to significantly improve osteoblast adhesion to the implant surface. The ‘Roxolid’ implants manufactured by the Straumann Company are made of this alloy and have been used for more than five years with good success rates. The strength of the material enables smaller implant diameters to be made with internal connections.
- Zirconium/Ceramic
Zirconium, a ceramic biocompatible biomaterial, has gained popularity as an implant material due to its aesthetics and was first tested during the 1990s as an implant material. The oxide form of zirconium has the capacity to create OI of a lesser quality and etching techniques have been used to improve the quality and extent of the OI. There are two zirconium implants available on the market. The ‘Ceraroot’ implant is an acid-etched zirconium implant (Oral Iceberg) and Straumann Pure ZLA is also an acid-etched ceramic implant (Figure 2.5a, 2.5b).

Figure 2.5 a. b: Zirconium implants. a: Straumann. b: Ceraroot.
- Implant Design
The design of an implant facilitates the primary stability during the placement of the implant and includes the shape and thread configuration of the implant. Other parameters such as diameter and length are also important and influence primary stability indirectly.
- Implant shape
The implant shape ensures there is a good approximation between the bone and implant and facilitates force distribution to the bone. Three shapes, cylindrical, parallel sided and tapered, have been used with the cylindrical form implants manufactured as unthreaded implants inserted using a ‘press-fit’ action into the prepared site (Figure 2.6). These implants are no longer available due to compromised treatment outcomes. Parallel-sided and tapered/conical screw (also called threaded) form implants have been used with the former being more popular until recently where hybrid designs combining the features of both these shapes becoming more widely used (Figure 2.7). These hybrid implants are designed to increase the contact area between the implant and bone as well as relieving stress concentrations due to the different thread designs thereby improving the stability enabling reduced healing times (Figure 2.8). Most implant systems have introduced these ‘hybrid’ versions into their portfolio whilst still retaining the standard implant designs.
Figure 2.6 Cylindrical implants used with a press fit action for placement (IMZ implant with TPS-coated surface).
Figure 2.7 Parallel-sided and tapered implants.
Figure 2.8 Hybrid-designed implant (parallel sided at the top; tapered apically).
- Implant shape
- Implant threads
The threads on a implant screw determine the degree to which the stability of the implant when placed is achieved and also facilitates the stress distribution to the bone. The degree of the stability achieved will be dependent on the profile and configuration of the threads called the thread depth and pitch (Figure 2.9). Screw form implants have threads that are designed to engage into the alveolar bone thereby improving primary stability and the sharper the thread pitch the better the stability. This is a feature that has been introduced into most implants systems as the surgical provision of implants has moved towards immediate and delayed placement. Figure 2.10 shows the different thread configurations and shapes which influence the stress concentration on the bone. Most implant systems adopt a combination of thread designs incorporating microthreads coronally with a tapering thread profile, double and triple thread configurations and increased thread width, pitch and depth. The use of microthreads coronally is deemed to increase the contact between the coronal bone and implant thus reducing the risk of coronal bone loss whereas, changes in thread pitch and depth are aimed at providing faster penetration into the bone thereby reducing risk of trauma to the bone. These changes in design have enabled improved primary stability thus contributing to faster osseointegration and healing (Figure 2.11a, 2.11b).
Figure 2.9 a, b: Different implant thread configurations. a: The machined implant on the right was the first implant and the change in the shape and design as well as the thread pitch is evident as you go from left to right. b: Different thread shapes and pitch (macrodesign).
Figure 2.10 Shows the progressive change in an implant thread design: The implant on the left shows the change in the depth and pitch of the threads, and the implant on the right shows the microthreads at the top aimed at improving the contact with the coronal bone.
Figure 2.11a The progressive change in the implant shape and thread profiles.
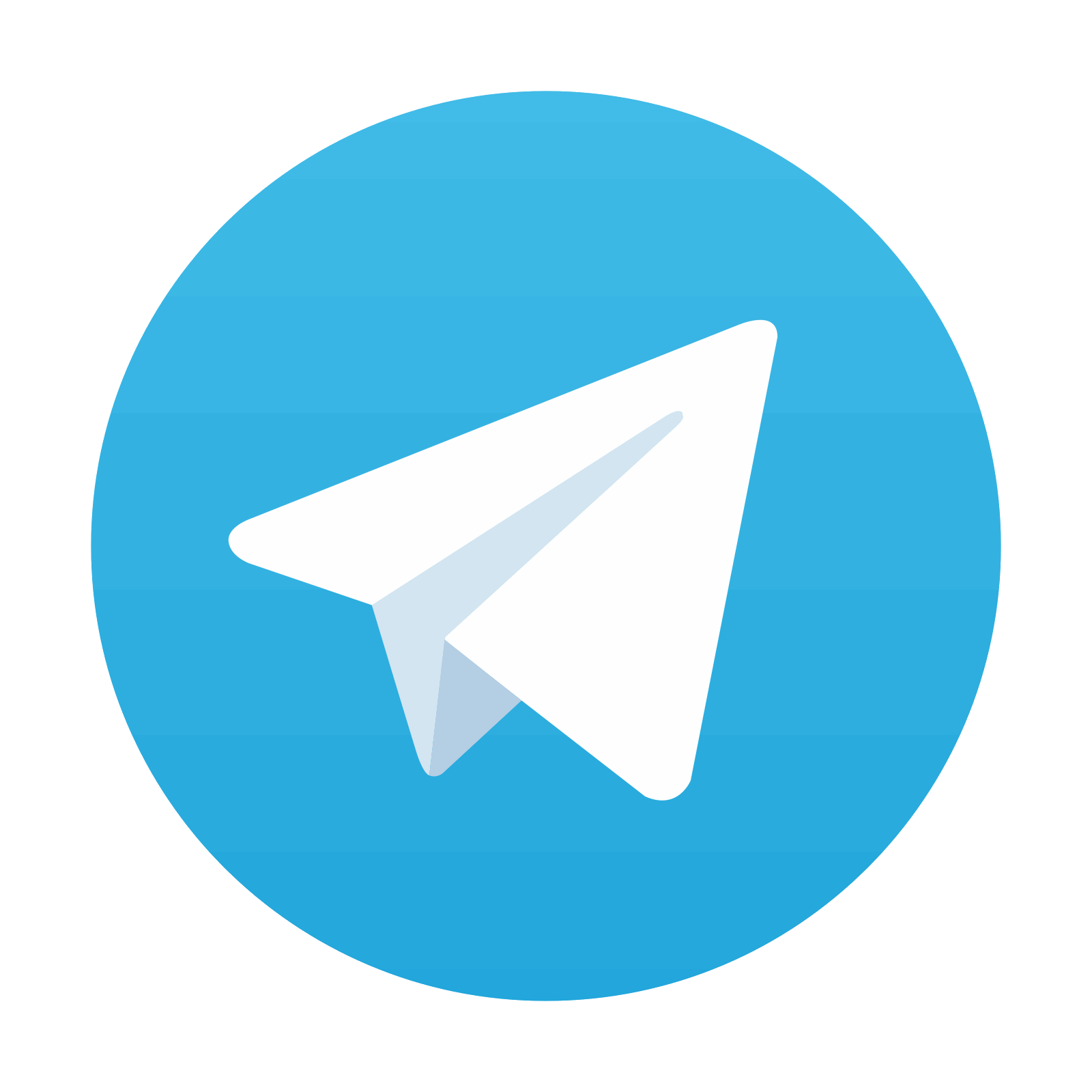
Stay updated, free dental videos. Join our Telegram channel

VIDEdental - Online dental courses
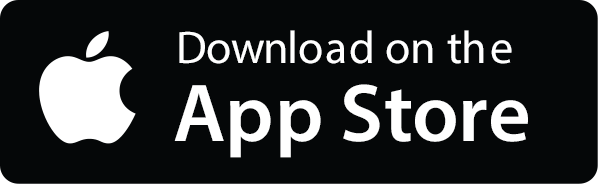
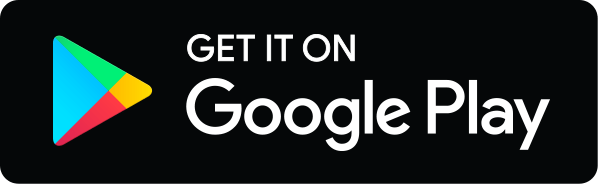