Abstract
The aim of this study was to determine the quantitative assessment of bone volume defects using multislice computed tomography (CT), and to establish an image processing protocol that enables this analysis. Bone defects were created in nine dry skulls, to mimic oral clefts. All the defects were modelled with wax, following the contralateral shape of the alveolo-palatal region. The wax was used to obtain the real volume of the cleft (gold standard). The skulls (with and without wax) were submitted to multislice CT examination, and the images were processed in an independent workstation using volumetric software. All the images were analysed twice, by two examiners at different times, performing intra- and inter-examiner analyses. The results show that the protocol for image processing has high efficiency ( P = 0.995). Multislice CT showed excellent results, with high reliability in the study of bone defect volume ( P = 0.997). The intra- and inter-observer analysis showed no statistically significant differences. It was demonstrated that multislice CT is a reliable technique in the volumetric assessment of bone defects in the alveolar and palatal regions, and that this method was efficient for calculating the volume of the clefts.
The development of congenital facial, lip and palate abnormalities usually takes place between the fourth and twelfth weeks of intrauterine life, due to a deficiency, or absence, of fusion of the maxillary and medium nasal and/or palatal processes . Alveolar bone grafting is an essential procedure in the overall management of patients with a cleft bone defect providing bone support for dental implants and prosthetic rehabilitation .
Different imaging methods have been used to assess the extent of the oral cleft and follow up treatments based on bone grafts . When these examinations are performed before bone graft surgery, they allow the size, position and structures involved by the cleft to be estimated. After surgery, imaging examinations assist the outcome of the bone graft, monitoring the eruption of teeth adjacent to the graft and evaluating the amount of bone available for the insertion of the implants during the rehabilitation process .
Conventional radiography is often used for preoperative evaluation, but it does not show anatomic details in the cross sectional view, and the anatomical structures are shown overlapping . The increased use of volumetric imaging examinations in dentistry has enabled a better understanding of the morphological structures, aiding the diagnosis and treatment of the various processes that affect this region. Computed tomography (CT) allows precise assessment of the shape, quality (cortical and cancellous), height and thickness of the bone by using multi-planar reconstructions . The use of CT using three-dimensional (3D) CT protocols provides excellent visualisation of the bone architecture, and is considered a valuable tool in the evaluation of craniofacial deformities in patients with congenital malformations, such as cleft palate and alveolar ridge .
There are well-defined protocols for the treatment of oral clefts from the prenatal period into adulthood, but there is a lack of studies related to the development of a methodology capable of determining the volumetric size of the bone defect in volumetric imaging examinations. The aims of this study were to determine the applicability of multislice CT to determine the actual volume of bone defects in dry skulls, and to establish a protocol for image processing that enables this measurement.
Material and methods
Nine dry skulls were used to make bone defects in the region of the alveolar ridge and hard palate, mimicking unilaterally trans-foramen cleft. The bone defects were initially drawn on the skulls with a permanent marker pen, to guide cutting. Using a pneumatic saw (Micro 100 Reciprocating Pneumatic Handpiece; Zimmer Hall, Linvatec Corporation, Ohio, USA) pressurized by a cylinder of compressed air, bone defects were made differing in sizes, shapes and positions between the left and right sides ( Fig. 1 ). All bone defects produced were modelled with wax, following the contralateral shape of the alveolar ridge and hard palate ( Fig. 2 ). This wax was used as a model for obtaining the actual volume of the bone defects (described later using Archimedes’ principle in the gold standard analysis) and to serve as a contrast during the imaging assessment of the defects.


All the skulls were submitted to multislice CT scanning (Brilliance CT – 6-slice; Phillips Medical System, USA) following the parameters: slice thickness: 0.8 mm; table increment: 0.87 mm; reconstruction interval: 0435 mm; matrix: 1024 × 1024; 135 kVp; 250 mA; and field of view (FOV): 16 cm. The skulls were scanned twice, with and without wax, and dipped in a container with water to mimic the soft tissues of the face (skin, subcutaneous fat and muscles) ( Fig. 3 ).

Axial cuts were made extending from the calvaria to the maxillary teeth cusps, and the cutting plane was positioned parallel to the hard palate. All the original axial images were stored on CD-ROM in DICOM (Digital Imaging Communications in Medicine) format on the islands of the CT scan, and then carried to an independent workstation (Dell ® 420 – Windows XP) containing the program Vitrea, version 3.6 (Vital Images Inc., Plymouth, MN, USA). Before analysing the image, the criteria used to define the limits of the bone defects were determined. The criteria used in the skulls scanned with the wax model were defined through the limits promoted by the lower attenuation of the wax compared with the water and the bone tissues, using the soft tissue window tool on the workstation ( Fig. 4 ). In skulls scanned without the mould, the criteria used mirrored (following the bone contour) the morphological structures of the normal contralateral side ( Fig. 5 ). The images in which defects were identified (with and without the mould) were outlined with the mouse using a tool called ‘Free’ to remarket the region of interest. The computer automatically gives the area of each design slice, and the volume of the defect was obtained by multiplying the sum of the areas with the range of the image reconstruction (volume of bone defect = ∑sum of areas outlined × range of the image reconstruction), which is obtained automatically by applying the commands ‘Surface’ and ‘Measure’ to obtain the corresponding area and volume of the bone defect. After complete selection of the area of interest (in all the axial images), the 3D reconstruction was used for final visualisation of the anatomical structures ( Fig. 6 ), following the methodology used in previous studies .



The authors compared the volumetric data obtained through the outlining of the bone defects made with and without the mould. This analysis was conducted by two postgraduate students (examiners 1 and 2) with extensive experience in interpreting CT scans, independently and on separate occasions, each making their own decisions about the limits of the bone defects. Previous training was given, so that each examiner felt comfortable with the use of the electronic measuring tools. Examiner 1 performed the steps at two different times, and examiner 2 performed the steps only once, to test the accuracy of the measurements (intra- and inter-observer). The purpose of this comparison is to validate this methodology for determining the volume of bone defects and cleft palate edge. These data were compared with the gold standard of the analysis, which was defined by the real volume of the wax model, calculated using Archimedes’ principle of water displacement. Using a precision balance (Adventurer, Ohaus, Pine Brook, NJ, USA) several steps were performed to obtain the actual volume of the wax models. Initially, a suspended hook system was used, with a weight to prevent the wax from floating during its immersion in the container of water. Using the precision balance, the mass of the hook system, with the counterweight and without the wax in the air, was initially determined (system mass on the air) ( Fig. 7 A ). Next, the hook system with the counterweight was submerged in a tub of Becker (200 ml) containing 150 ml of water (this volume of water was kept constant during all the steps) to calculate the mass of the immersed system ( Fig. 7 B). The wax model was placed on the hook system and hung on the precision balance to calculate the mass of this system (hook system + wax model of each skull) measured in air (system mass + wax mass in the air) ( Fig. 7 C). This system was completely submerged in water and its mass was measured (system mass + wax mass immersed) ( Fig. 7 D).

The volume of each wax model was determined using the following formula:
[ ( system mass + wax mass in the air ) − ( system mass + immersed wax mass ) ] − [ ( system mass in the air ) − ( system mass + immersed wax mass ) ] p H 2 O distilled at 25 ° C = volume of wax model ;
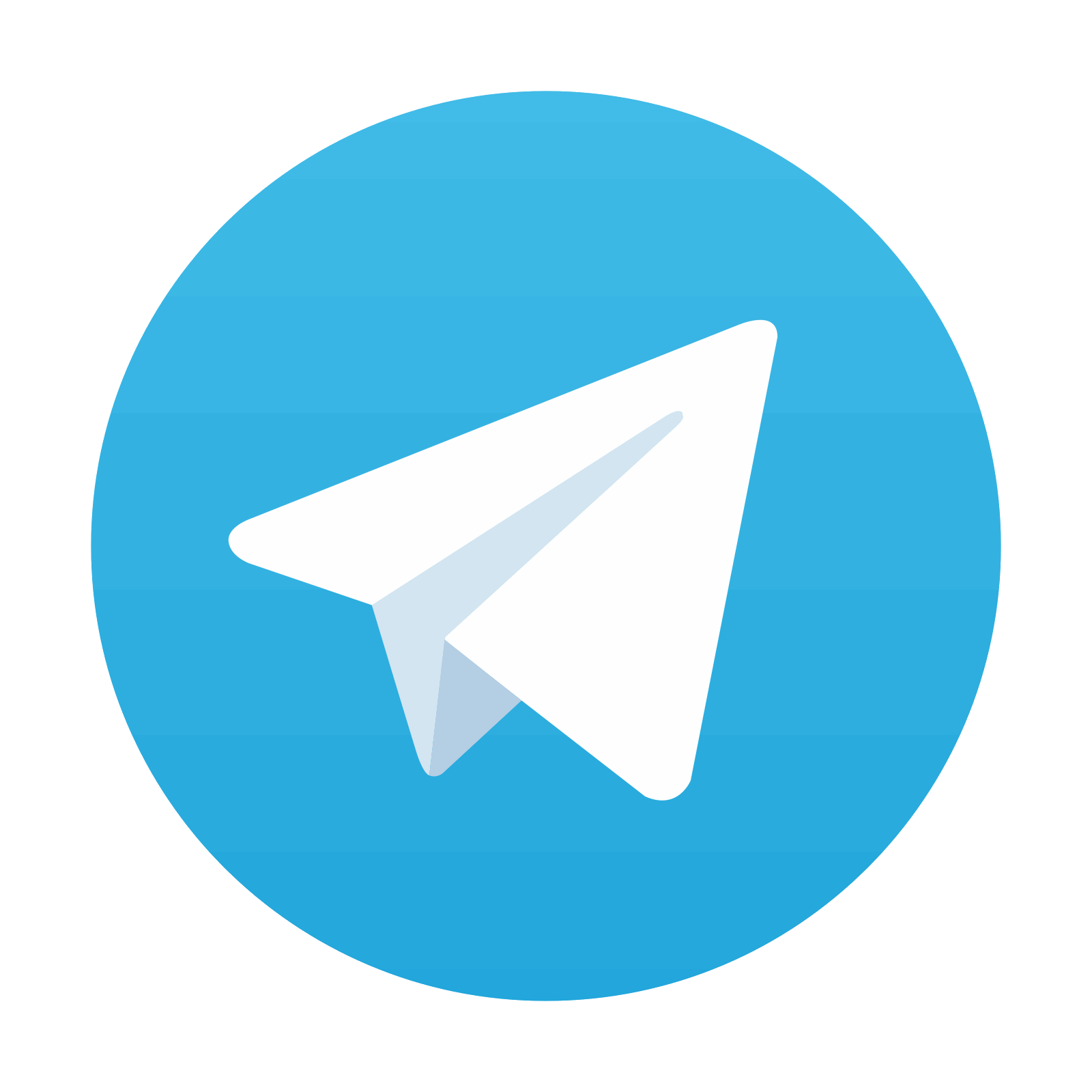
Stay updated, free dental videos. Join our Telegram channel

VIDEdental - Online dental courses
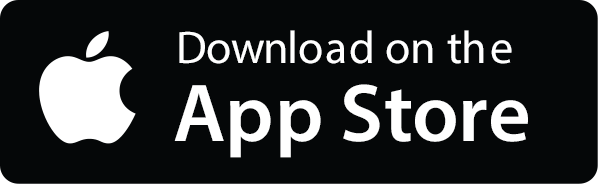
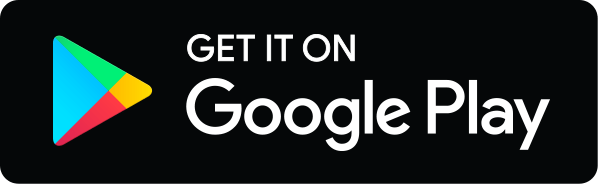