Abstract
Objectives
This electron microscopic study aimed at investigating effects of oral astringent stimuli on the enamel pellicle’s morphology.
Methods
Pellicles were formed in situ within 30 min on bovine enamel slabs, fixed to individuals’ upper jaw splints. The pellicle-coated specimens were immersed in vitro in seven diverse astringent solutions and subsequently analyzed by scanning electron microscopy (SEM), energy dispersive X-ray (EDX) spectroscopy, as well as transmission electron microscopy (TEM). Four biocompatible astringents, namely the polyphenol epigallocatechin gallate, the metal salt iron(III) sulfate, the basic protein lysozyme, and the aminopolysaccharide chitosan, were additionally applied in situ . After rinsing the oral cavity with these compounds, the pellicle’s ultrastructure was imaged by SEM and TEM, respectively. Untreated pellicle samples served as controls.
Results
Exposure to polyphenols and lysozyme induced particularly thicker and electron-denser pellicles in comparison to the control pellicle with similar characteristics in vitro and in situ . In contrast, acidic chitosan and metal salt solutions, respectively, revealed minor pellicle alterations. The incorporation of Fe and Al into the pellicles treated with the corresponding inorganic salts was verified by EDX analysis.
Conclusions
Astringent-induced pellicle modifications were for the first time visualized by TEM. The ultrastructural alterations of the dental pellicle may partly explain the tooth-roughening effect caused by oral astringent stimuli.
Clinical significance
Astringents might modify the pellicle’s protective properties against dental erosion, attrition, as well as bacterial adhesion, and by this means may influence tooth health. The findings may thus be particularly relevant for preventive dentistry.
1
Introduction
The dental pellicle is a 0.1–1 μm thick proteinaceous layer which forms instantaneously on the enamel’s surface when in contact with saliva . A two-step mechanism has been proposed for pellicle development: Small proteins rapidly adsorb to the tooth surface, followed by a slower, continuous adsorption of proteins to the already protein-coated enamel. As observed by transmission electron microscopy the initial pellicle layer is apparent as an electron-dense basal layer, whereas the outer layer consists of a less electron-dense film with globular and granular structures .
The protein film acts as a lubricant, protecting the teeth from attrition and abrasion, displays a barrier against erosive noxae, and has antibacterial properties. Besides these beneficial effects on tooth health, the pellicle also provides a basis for biofilm formation as several proteinaceous constituents facilitate bacterial adhesion .
Plant polyphenols such as epigallocatechin gallate found, e.g., in green tea extract , have been described to enhance the tenacity and thickness of the proteinaceous pellicle layer and improve the pellicle’s protective properties against erosive noxae . As the ingestion of polyphenols is typically accompanied by a mouth-puckering sensation and a loss of the pellicle’s inherent lubricating properties associated with blunt teeth , a phenomenon also referred to as oral astringency , we asked whether astringent molecules generally modified the pellicle’s morphology. Besides polyphenols, commonly-used haemostatic agents for oral interventions are perceived as oral astringents, such as the metal salts iron(III) sulfate and aluminum(III) chloride , alongside the aminopolysaccharide chitosan . A fourth class of astringent substances comprises proteins with a high isoelectric point , for example, the antibacterial enzyme lysozyme, which was found to be highly abundant in the acquired pellicle .
The present study tested the hypothesis whether oral astringency is generally linked to an alteration of the pellicle’s morphology. Therefore, pellicles were formed in situ on bovine enamel slabs, exposed in vitro and in situ to the latter set of chemically diverse model astringents and analyzed on the ultrastructural level by electron microscopy (TEM and SEM).
Astringent-induced ultrastructural modifications of the dental pellicle may influence its protective properties in respect to dental erosion or attrition. Furthermore, results of this study may provide molecular insights into the mechanism of blunt feeling teeth as evoked by the administration of haemostatic agents.
2
Methods
2.1
Subjects
Two healthy volunteers (age: 29 and 30 years), both members of the clinical staff, entered the study. The participants fulfilled the inclusion criteria showing no evidence of active caries or periodontal disease. Their mean stimulated saliva flow rate exceeded 1 ml/min. Subjects refrained from eating and drinking anything besides water 3 h before taking part in the experiments. Ethical approval (238/03; 2012) was granted by the medical ethics committee of the Medical Association of Saarland, Germany. The volunteers received verbal and written information concerning the study and signed a consent form prior to their participation.
2.2
Enamel specimen preparation
Enamel slabs (3 × 4 × 1.5 mm) were prepared from the labial surfaces of bovine incisor teeth (BSE-negative, 2-year old cattle). After cutting cylindrical specimens from the teeth with a saw (Conrad Apparatebau Clausthal GmbH, Clausthal-Zellerfeld, Germany), they were progressively polished by wet grinding with up to 4000 grit (Buehler, Düsseldorf, Germany). The enamel samples were disinfected, following a sequential protocol: After ultrasonication in sodium hypochlorite (3%) for 3 min to remove the smear layer, the slabs were thoroughly rinsed with distilled water. The specimens were then washed for 5 min in ice-cold water and ultrasonicated in ethanol (70%) for 15 min. Finally, the specimens were stored for 24 h in distilled water.
2.3
Astringent stimuli
Test compounds used for in situ and in vitro experiments were applied at concentrations explicitly above their orosensory threshold for astringency : (−)-Epigallocatechin gallate (EGCG) and Fe 2 (SO 4 ) 3 , respectively, were obtained from Sigma-Aldrich, St. Louis, MO, USA and dissolved in ultrapure H 2 O at a concentration of 5 mM and 1 mM, respectively. Lysozyme (Sigma-Aldrich, Steinheim, Germany) was used at a concentration of 0.5 mM. Poly-( β -(1 → 4)- d -glucosamine (chitosan, degree of deacetylation >92.6%, molecular weight ∼500 kDa, Heppe Medical Chitosan GmbH, Halle/Saale, Germany) was dissolved in 0.1 M HCl (aq) and was further diluted with H 2 O to gain a final concentration of 2 μM.
The following compounds were applied in vitro only: AlCl 3 ·6H 2 O (Carl Roth, Karlsruhe, Germany) was used as a 5 mM solution to approximate the Al content in the commercial astringent mouth rinse Mallebrin ® . Tannic acid (Caelo, Hilden, Germany) was used at a concentration in the order of 1 mM. To isolate the astringent, high molecular weight fraction from red currant seeds, 500 g fresh red currants ( Ribes rubrum ), which were bought on a local market, were squished and the seeds were mechanically separated from the fruit pulp, with the seed coats intact. The astringent seed coat liquid was extracted with 70% MeOH. The solvent was removed and the crude extract freeze-dried. The lyophilizate was taken up in H 2 O and further fractionated by a Vivacell 250 static gas pressure ultrafiltration unit equipped with a 5 kDa molecular weight cutoff polyethersulfone membrane (Sartorius, Göttingen, Germany). After freeze-drying, the high molecular weight astringent retentate of the seed coat extract was used at a concentration of 5 mg/mL for in vitro experiments. Table 1 summarizes the concentrations of the astringent solutions and experimental conditions used within this study.
Compound class | Test compound | Molecular weight [g/mol] | Concentration [mg/ml] | Experimental condition |
---|---|---|---|---|
Polyphenol | (−)-Epigallocatechin gallate (EGCG) | 458 | 2.3 | in vitro/in situ |
Tannic acid | ∼1700 # | 1.7 | in vitro | |
Red currant seed coat extract | >5000 | 5.0 | in vitro | |
Metal Salt | Fe 2 (SO 4 ) 3 | 399 | 0.4 | in vitro/in situ |
AlCl 3 ·6H 2 O | 241 | 1.2 | in vitro | |
Aminopolysaccharide | Chitosan | ∼500,000 | 1.0 | in vitro/in situ |
Basic Protein | Lysozyme | 14,300 | 7.1 | in vitro/in situ |
2.4
In situ pellicle formation
Individual splints were manufactured for the upper jaw from 1.5 mm thick methacrylate foils (Scheu-Dental, Iserlohn, Germany) using a thermoforming technique (Erkopress ES 2004, Erkodent GmbH, Pfalzgrafenweiler, Germany). The bovine enamel slabs were then mounted on the buccal side of the splints with a polyvinyl siloxane impression material (President Light Body, Coltène, Switzerland), exposing only the enamel surface to the oral environment.
For initial in situ pellicle formation the splints were carried in the oral cavity for 30 min. After removing the splints, the slabs were thoroughly rinsed with distilled water to remove loosely-attached saliva components.
2.5
In vitro experiments
Pellicle-coated specimens of both individuals (two samples each) were immersed in vitro for 1 min in one of the aqueous solutions of the astringents EGCG, tannic acid, red currant seed extract, Fe 2 (SO 4 ) 3 , AlCl 3 , chitosan, and lysozyme, and then rinsed with distilled water. One of the slabs was prepared for scanning electron microscopy, the other slab for transmission electron microscopy.
2.6
In situ experiments
After initial in situ pellicle formation, two specimens were directly removed from the oral cavity to serve as control pellicles. Solutions (10 ml) of iron(III) sulfate, chitosan, EGCG, and lysozyme, respectively, were then used for intraoral rinsing (30 s). Two specimens were removed from the oral cavity immediately after rinsing with astringent, whereas a final set of two samples was left intraorally for 30 min. All slabs were rinsed with distilled water and prepared for either transmission electron microscopy or scanning electron microscopy.
2.7
Scanning electron microscopy (SEM)
The samples were fixed in a solution containing 2.5% glutaraldehyde and 0.1 M cacodylate buffer for 1 h at 4 °C and were then washed with 0.1 M cacodylate buffer. After dehydration with an ascending series of ethanol, specimens were chemically dried using hexamethyldisilazane (HMDS) and sputtered with carbon (sputter coater SCD 030, Balzers, Liechtenstein). The pellicle’s surface micromorphology was imaged using a scanning electron microscope (Fei XL30 ESEM FEG, FEI Company, Eindhoven, Netherlands) at a magnification up to 20,000-fold. Potential traces of Fe or Al were determined with an energy-dispersive X-ray (EDX) analyzer (SEM-EDX Philips XL30, Philips, Eindhoven, Netherlands) integrated in the environmental scanning electron microscope (Fei XL30 ESEM FEG, FEI Company, Eindhoven, Netherlands).
2.8
Transmission electron microscopy (TEM)
After fixing the specimens in 2.5% glutaraldehyde and 0.1 M cacodylate buffer for 1 h at 4 °C, they were washed in 0.1 M cacodylate buffer. Osmium tetroxide (1%) was then used for post-fixation (2 h) before the samples were dehydrated in an ascending series of ethanol and embedded in Araldite CY212 (Agarscientific, Stansted, United Kingdom). After removing the dentine with a diamond bur, the enamel part was decalcified in 1 M HCl (aq) . The specimens were then re-embedded in Araldite. Cutting of ultrathin sections in series was performed with an ultramicrotome (Ultracut E, Bensheim, Germany), using a diamond knife. The sections were then mounted on Pioloform-coated copper grids (Plano, Wetzlar, Germany) and contrasted with aqueous solutions of uranyl acetate and lead citrate. The samples were imaged at magnifications from 20,000- to 100,000-fold using a TEM TECNAI 12 Biotwin (FEI, Eindhoven, Netherlands) and images were processed digitally. Pellicle thickness was measured every 150 nm. Means of pellicle thickness were calculated using a minimum of 50 single point measurements.
2
Methods
2.1
Subjects
Two healthy volunteers (age: 29 and 30 years), both members of the clinical staff, entered the study. The participants fulfilled the inclusion criteria showing no evidence of active caries or periodontal disease. Their mean stimulated saliva flow rate exceeded 1 ml/min. Subjects refrained from eating and drinking anything besides water 3 h before taking part in the experiments. Ethical approval (238/03; 2012) was granted by the medical ethics committee of the Medical Association of Saarland, Germany. The volunteers received verbal and written information concerning the study and signed a consent form prior to their participation.
2.2
Enamel specimen preparation
Enamel slabs (3 × 4 × 1.5 mm) were prepared from the labial surfaces of bovine incisor teeth (BSE-negative, 2-year old cattle). After cutting cylindrical specimens from the teeth with a saw (Conrad Apparatebau Clausthal GmbH, Clausthal-Zellerfeld, Germany), they were progressively polished by wet grinding with up to 4000 grit (Buehler, Düsseldorf, Germany). The enamel samples were disinfected, following a sequential protocol: After ultrasonication in sodium hypochlorite (3%) for 3 min to remove the smear layer, the slabs were thoroughly rinsed with distilled water. The specimens were then washed for 5 min in ice-cold water and ultrasonicated in ethanol (70%) for 15 min. Finally, the specimens were stored for 24 h in distilled water.
2.3
Astringent stimuli
Test compounds used for in situ and in vitro experiments were applied at concentrations explicitly above their orosensory threshold for astringency : (−)-Epigallocatechin gallate (EGCG) and Fe 2 (SO 4 ) 3 , respectively, were obtained from Sigma-Aldrich, St. Louis, MO, USA and dissolved in ultrapure H 2 O at a concentration of 5 mM and 1 mM, respectively. Lysozyme (Sigma-Aldrich, Steinheim, Germany) was used at a concentration of 0.5 mM. Poly-( β -(1 → 4)- d -glucosamine (chitosan, degree of deacetylation >92.6%, molecular weight ∼500 kDa, Heppe Medical Chitosan GmbH, Halle/Saale, Germany) was dissolved in 0.1 M HCl (aq) and was further diluted with H 2 O to gain a final concentration of 2 μM.
The following compounds were applied in vitro only: AlCl 3 ·6H 2 O (Carl Roth, Karlsruhe, Germany) was used as a 5 mM solution to approximate the Al content in the commercial astringent mouth rinse Mallebrin ® . Tannic acid (Caelo, Hilden, Germany) was used at a concentration in the order of 1 mM. To isolate the astringent, high molecular weight fraction from red currant seeds, 500 g fresh red currants ( Ribes rubrum ), which were bought on a local market, were squished and the seeds were mechanically separated from the fruit pulp, with the seed coats intact. The astringent seed coat liquid was extracted with 70% MeOH. The solvent was removed and the crude extract freeze-dried. The lyophilizate was taken up in H 2 O and further fractionated by a Vivacell 250 static gas pressure ultrafiltration unit equipped with a 5 kDa molecular weight cutoff polyethersulfone membrane (Sartorius, Göttingen, Germany). After freeze-drying, the high molecular weight astringent retentate of the seed coat extract was used at a concentration of 5 mg/mL for in vitro experiments. Table 1 summarizes the concentrations of the astringent solutions and experimental conditions used within this study.
Compound class | Test compound | Molecular weight [g/mol] | Concentration [mg/ml] | Experimental condition |
---|---|---|---|---|
Polyphenol | (−)-Epigallocatechin gallate (EGCG) | 458 | 2.3 | in vitro/in situ |
Tannic acid | ∼1700 # | 1.7 | in vitro | |
Red currant seed coat extract | >5000 | 5.0 | in vitro | |
Metal Salt | Fe 2 (SO 4 ) 3 | 399 | 0.4 | in vitro/in situ |
AlCl 3 ·6H 2 O | 241 | 1.2 | in vitro | |
Aminopolysaccharide | Chitosan | ∼500,000 | 1.0 | in vitro/in situ |
Basic Protein | Lysozyme | 14,300 | 7.1 | in vitro/in situ |
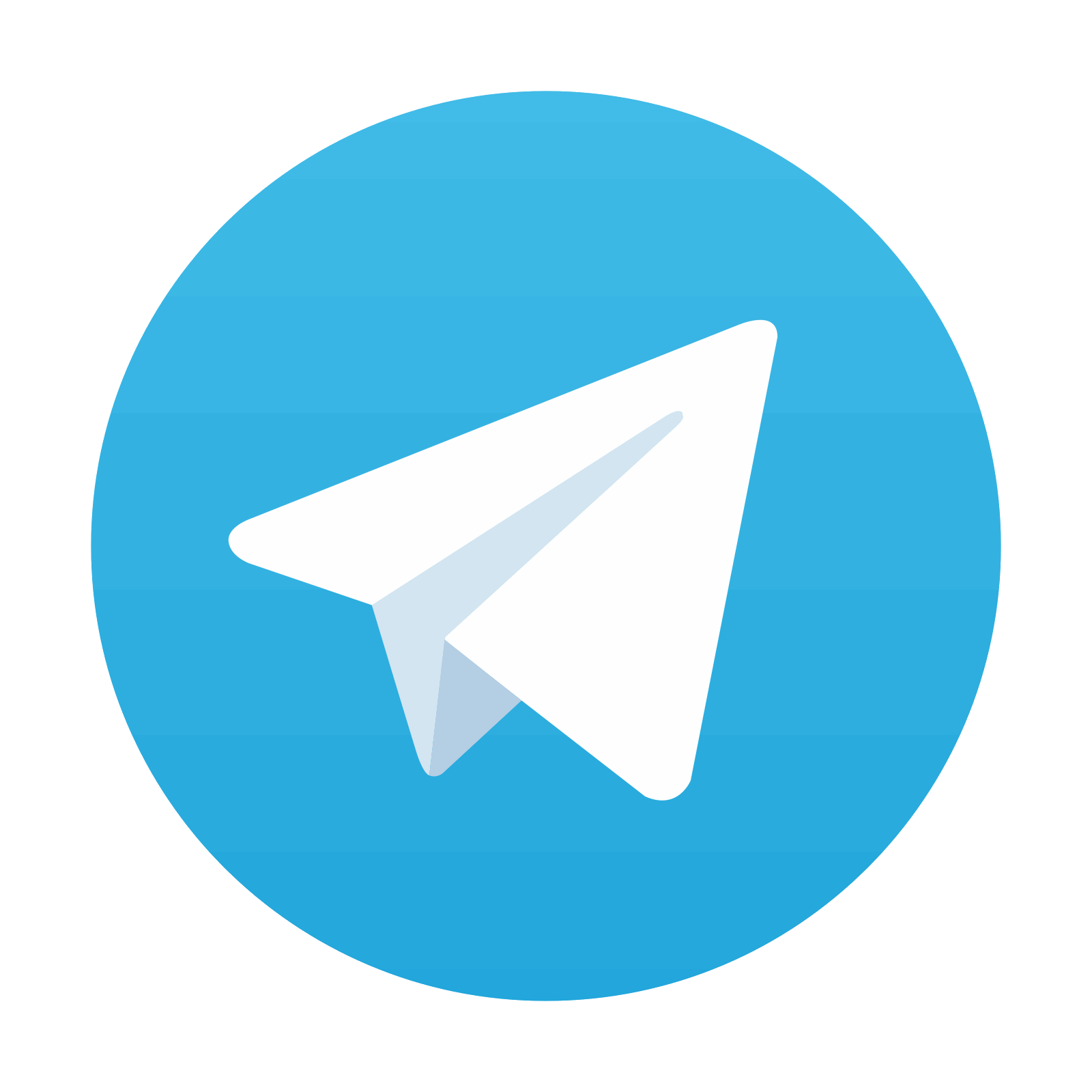
Stay updated, free dental videos. Join our Telegram channel

VIDEdental - Online dental courses
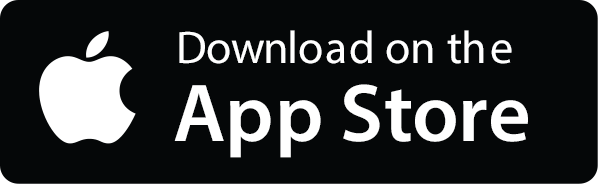
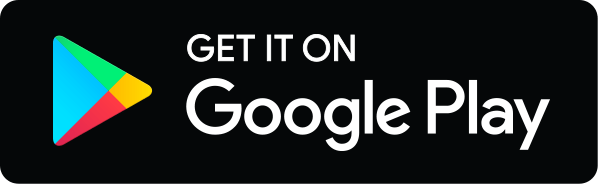
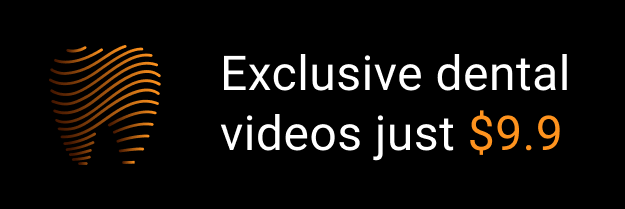