div epub:type=”chapter” role=”doc-chapter”>
S. Nares (ed.)Advances in Periodontal Surgeryhttps://doi.org/10.1007/978-3-030-12310-9_7
7. Regeneration of Intrabony Defects Utilizing Stem Cells Allograft
Stem cellsIntrabony defectsPeriodontal regenerationAllograft
7.1 Introduction
Periodontitis is commonly characterized by the formation of intrabony defects. Pioneering research on the use of autogenous bone grafts of both extra- and intraoral sources has been used successfully for periodontal regeneration [1]. This has been accepted as the gold standard for grafting materials. However, critical limitations for autogenous bone use include limited supply and possible need for multiple donor sites, and donor site morbidity can make this therapeutic approach less than desirable. Several surgical approaches shown to be effective in improving clinical and radiographic parameters such as clinical attachment level (CAL) and defect depth utilizing bone replacement grafts and/or guided tissue regeneration (GTR) are well documented in the literature [2–5]. However, these approaches have limitations in the predictability and effectiveness of regenerative therapy, inability to achieve vertical bone growth beyond the existing bony walls and therapeutic inconsistencies. This has resulted in the search for alternative regenerative strategies.
More recently, tissue engineering approaches for periodontal regeneration involving grafting with scaffolding material (i.e., demineralized or mineralized freeze-dried bone allograft materials (DFDBA, FDBA) or tricalcium phosphate (TCP)), grafting and/or recruitment of mesenchymal stem cells (MSCs, also known as multipotential stromal cells), and the use of signaling molecules (i.e., growth factors such as recombinant human platelet-derived growth factor (rhPDGF), recombinant human fibroblast growth factor (rhFGF), and enamel matrix derivative (EMD)) have been proposed to potentially overcome some of these limitations [6]. This has resulted in the extensive study of the use of biologics, like enamel matrix derivative (EMD) and recombinant human platelet-derived growth factor-BB plus β-tricalcium phosphate (rhPDGF-TCP) [7–12]. The past 15 years of studies have concluded that these biologic agents generally enhanced periodontal regeneration with comparable results similar to DFDBA and GTR. Additionally, it has been shown that these methods are superior to open flap debridement procedures in improving clinical parameters [13]. Long-term studies indicate that the improvements are maintainable up to 10 years, even in severely compromised teeth, consistent with a favorable, long-term prognosis.
In search of improved healing response in advanced compromised periodontal intrabony defects, we have explored if augmentation of all three components of tissue engineering variables, namely, the addition of stem cells, scaffolding graft material, and signaling molecules, will singularly or in combination enhance periodontal regeneration. In this chapter, we describe the use of stem cell allograft for the correction of intrabony defects and address whether the results can be improved if used in combination with guided tissue regeneration and/or recombinant human platelet-derived growth factor. Specifically, we examined if a commercially available MSCs preparation seeded on DFDBA when used singularly or in combination with rhPDGF +/− with GTR membrane will enhance periodontal regeneration.
7.2 Use of MSCs for Periodontal and Dental Implant Applications

Periodontal regeneration by applying tissue engineering principles
Stem cell protocols have the potential to improve current bone regeneration methods through increased bioactivity of grafting scaffolding, targeted growth factor delivery, and cell recruitment [6]. The concentration of MSCs in a cellular bone allograft compared with fresh age-matched iliac crest bone and bone marrow (BM) aspirate has been investigated [19, 20]. In these studies, the authors reported that without cultivation or expansion, the allograft displayed an osteoinductive molecular signature and the presence of CD45 − CD271 + CD73 + CD90 + CD105 + MSCs with a purity over 100-fold that of iliac crest bone. In comparison with BM, MSCs numbers enzymatically released from 1 g of cellular allograft can contain MSCs equivalent to approximately 45 mL of BM aspirate. They concluded that MSCs cellular allograft bone represents a unique “living” nonimmune matrix rich in MSCs and osteocytes. This osteoinductive cellular graft represents an attractive alternative to autograft bone, which reduces supply and morbidity issues. When used in the manner as we have described in the case series design, the cost of the material can be reduced to $100–150 per defect site depending on the size of the defect. Our experience is that a 1 g unit can be used to correct at least four large intraosseous defects. If the defects are narrow or have furcation involvement, a single unit can be used to manage additional cases.
Early application of MSCs preparations in dentistry has focused on its application for implant site preparation. Pioneering work by McAllister first demonstrated the usefulness of MSCs preparations for sinus augmentation procedures [15]. In a histomorphometric study, a comparison of bone formation following sinus augmentation procedures using either a MSCs allograft cellular bone matrix containing native MSCs or a conventional allograft was assessed. Results over a 3.7 months. Follow-up healing period of the test group revealed a mean of 32.5 and 4.9% for vital bone and remaining graft content, respectively. In the control group, only 18.3% of the bone content was vital, and 25.8% of residual conventional graft remained [15]. Rickert et al. [21] reported similar results when they compared bovine-derived mineral bone seeded with mononuclear stem cells with bovine-derived mineral bone mixed with autogenous bone. Significantly greater bone formation was observed in the test group when compared with the control group at 14 weeks (17.7% vs. 12%, respectively). Another application of MSCs for implant site preparation were case reports whereby MSCs preparations were used to increase vertical and horizontal bone volume. In these case reports, the MSCs allograft combined with a titanium mesh created space maintenance for ridge augmentation in both width and vertical dimensions [22, 23].
To date, there have been limited case reports on the use of MSCs for periodontal regeneration. Pioneering animal studies have demonstrated that MSCs can enhance the regeneration of periodontal defects in dogs [24]. The clinical use of MSCs cellular allograft for treatment of human periodontal defects was first reported in both a single-rooted and a multi-rooted tooth [22]. In the single-rooted case, a significant reduction in probing was obtained with radiologic evidence of approximately 4 mm of vertical bone fill at 6 months following grafting. In the multi-rooted case, clinical evidence showed decreased probing depths and radiographic bone improvement at 6 months. A CBCT scan taken at 14 months demonstrated three-dimensional bone fill. A similar result was presented in a case report by Koo et al. [25], which describes the use of allograft cellular bone matrix containing MSCs in the treatment of a severe periodontal defect. These case reports indicate a potential resolution of periodontal defects using cellular allograft material. There is limited information regarding the use of MSCs for the correction of furcation defects other than a case report by Rosen [26], where a Class III furcation defect on a mandibular molar was corrected utilizing MSCs in conjunction with a resorbable GTR membrane. On reentry for implant placement, the regeneration of the furcation defect was confirmed radiographically, as well as clinically.
Though these case reports and series are encouraging, there has been no thorough examination of whether MSCs can enhance the potential of regenerative strategies (rhPDGF and/or GTR membrane) either singularly or in combination.
7.3 Clinical Assessment of MSCs for Periodontal Regeneration
7.3.1 Case Series Design
In this first comprehensive study of the use of MSCs seeded on DFDBA, we ask the question if this alone, or in combination with other regenerative strategies (rhPDGF and/or GTR membrane), can enhanced the regenerative results.
-
Group I: MSCs seeded on DFDBA.
-
Group II: MSCs + GTR.
-
Group III: MSCs + rhPDGF-BB (0.3 mg/mL rhPDGF-BB).
-
Group IV: MSCs + rhPDGF-BB (0.3 mg/mL rhPDGF-BB) + GTR.
Patient enrollment, adverse event, and periodontal defect demographics
Group 1: MSCs |
Group 2: MSCs + GTR |
Group 3: MSCs + rhPDGF |
Group 4: MSCs + rhPDGF + GTR |
|
---|---|---|---|---|
Patients enrolled Male/female |
20 10/10 |
17 9/8 |
20 11/9 |
20 11/9 |
Adverse event* |
3 |
0 |
1 |
3 |
Patients’ completed study |
17 8/9 |
17 9/8 |
19 11/8 |
17 9/8 |
Intrabony defect Depth × width (mean) |
6.6 m × 3.9 mm |
6.8 mm × 3.6 mm |
6.7 mm × 3.5 mm |
6.6 mm × 3.9 mm |
Defect characteristics (mean) |
3—Circumferential 11—3 walls 3—2 walls |
2—Circumferential 10—3 walls 5—2 walls |
6—Circumferential 10—3 walls 3—walls |
4—Circumferential 11—3 walls 2—2 walls |
The duration of this study was 12 months following implantation of the study device. Subjects had a maximum of 13 visits, which included a screening visit, 2 pre-surgery visits (if necessary), baseline visit, surgical visit, and 8 post-op visits. The design was similar to those previously used to evaluate regenerative properties of rhPDGF [27–29]. Before study treatment, each subject completed full-mouth scaling and root planing to control the disease process and minimize lesion variability.
Surgical treatment consisted of the administration of local anesthesia followed by reflection of full thickness buccal and lingual flaps to allow visualization of the treatment site. Following debridement of the interproximal defect, conditioning of the root surfaces with a tetracycline paste, and measurements of the intrabony defects, the patients were randomized into the treatment groups. MSCs are commercially available as Osteocel® (Ace Surgical Supply, Brockton, MA, USA) and delivered to our clinical practice frozen on dry ice and stored in a − 80° refrigerator. If a − 80° refrigerator is not available, it can be stored for 2–3 days on dry ice. To utilize the MSCs, the cell preparation is thawed in a room temperature water bath. This takes approximately 15–20 min. The MSCs are delivered in 1 cc aliquots in a plastic container with a Millipore filter screen. The MSCs are washed twice with room temperature sterile saline. After washing, the cells may be used for a period of 5 h. In our office, we will “stack” the surgeries for the 1 cc of MSCs for use in multiple cases. It is important to maintain good sterile tissue management. It should be noted that the particles may be larger than what is normally use for periodontal surgery and we have found that the larger particles may be cut into smaller pieces with an orthodontic scissor. Application of rhPDGF and GTR membrane (RCM membrane, Osteogenic, Texas) for treatment group was applied as previously described [27–29]. The duration of the study was 12 months following surgery. Supragingival cleansing of the test sites was provided as needed. Supportive periodontal therapy was provided every 3 months post-surgically.
The primary goal of this clinical trial was to compare the safety and effectiveness of MSCs ± rhPDGF with or without GTR into intraosseous periodontal defects. Primary endpoints for the study included CAL (clinical attachment level) between baseline and 3, 6, 9, and 12 months; PD (probing depth) reduction between baseline and 6, 9, and 12 months; and GR (change in gingival recession) between baseline and 6, 9, and 12 months. Secondary endpoints consisted of a comparison of increased radiopacity (radiographic fill) of the intrabony defects based on monitoring radiographic films taken at 3, 6, 9, and 12 months as compared to baseline.
7.3.2 Case Series Results
Subjects and Demographics
Seventy-seven subjects were screened and randomized in this study. Approximately 9.01% of enrolled patients had perio-endo complications, and the prognoses of these teeth were deemed hopeless. These patients proceeded with extraction and plans for an implant support crown replacement [30]. No other adverse events or patient withdrawal occurred. The majority of the subjects had defects classified as 3-wall or 2–3-wall combination defects in the apical and coronal portion and located at DB aspects of mandibular second molars (Table 7.1).
Primary and Secondary Endpoints
Clinical endpoint for treatment population
Group 1: MSCs |
Group 2: MSCs + GTR |
Group 3: MSCs + rhPDGF |
Group 4: MSCs + rhPDGF + GTR |
|
---|---|---|---|---|
Initial PD |
8.5 ± 1.3 mm |
9.1 ± 1.5 mm |
8.8 ± 1.3 mm |
8.8 ± 1.3 mm |
PD at 3 months |
3.6 ± 0.7 mm |
4.0 ± 4.1 mm |
3.8 ± 0.5 mm |
3.8 ± 0.5 mm |
PD at 6 months |
3.2 ± 0.4 mm |
3.6 ± 0.9 mm |
3.4 ± 0.7 mm |
3.4 ± 0.7 mm |
PD at 9 months |
3.1 ± 0.2 mm |
3.5 + 0.9 mm |
3.1 ± 0.3 mm |
3.1 ± 0.3 mm |
PD at 12 months |
3.0 ± 0.2 mm |
3.5 ± 0.9 mm |
3.2 ± 0.4 mm |
3.2 ± 0.4 mm |
CAL gain at 12 months |
5.5 ± 1.2 mm |
5.4 ± 1.1 mm |
5.5 ± 1.4 mm |
4.6 ± 1.7 mm |
Gingival recession at 12 months |
−0.2 ± 0.7 mm |
−0.5 ± 0.9 mm |
−0.1 ± 0.3 mm |
−0.3 ± 0.7 mm |

This is a representative case of a deep intrabony defect on the distal aspect of the mandibular left second molar (a, b). The defect was degranulated and grafted with MSCs or MSCs + rhPDGF (c). Clinical improvement was observed 12 months after surgical treatment (d). Pre-surgical (e) and 12 months posttreatment (f) radiographs are presented
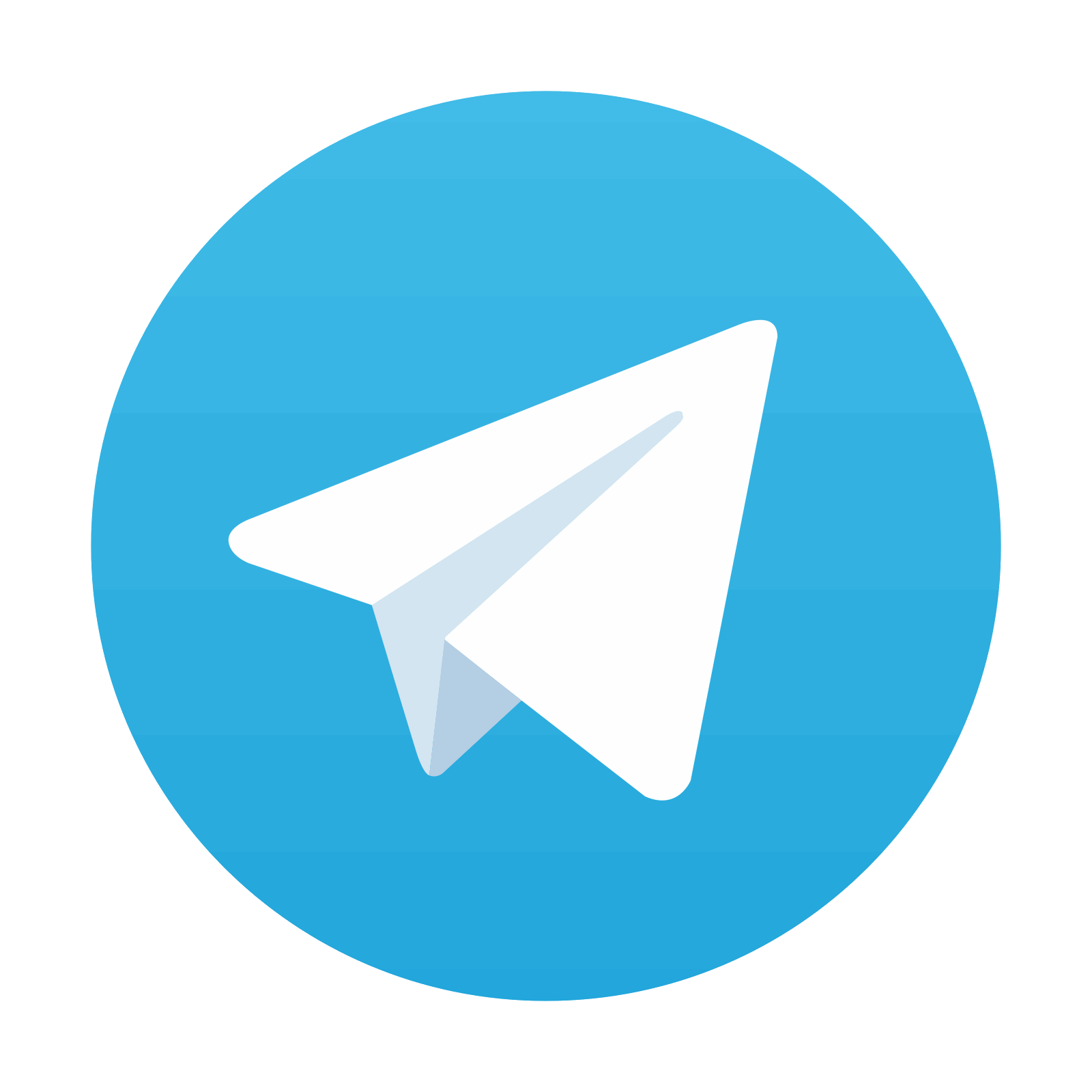
Stay updated, free dental videos. Join our Telegram channel

VIDEdental - Online dental courses
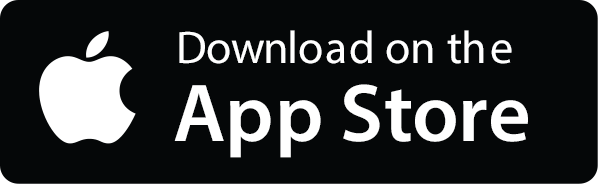
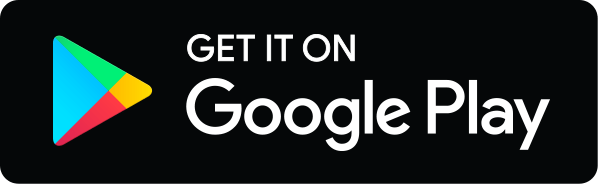