Abstract
Objectives
Collagen-based nanocomposite incorporating nanobioactive glass (Col/nBG) was developed as a scaffolding matrix for dentin–pulp regeneration. The effects of the novel matrix on the proliferation of human dental pulp cells (hDPCs) and their differentiation into odontoblastic lineage were investigated.
Methods
Nanocomposite scaffold was prepared by incorporating nBG within the Col solution and then reconstituting them into a membrane form. Cell growth by MTS assay, adhesion by scanning electron microscopy (SEM), and odontoblastic differentiation by alkaline phosphatase (ALP) activity, mineralization, and the mRNA expression of differentiation-related genes of DPCs on each scaffold were evaluated.
Results
The introduction of nBG significantly improved the bone mineral-like apatite formation in the simulated body fluid, suggesting excellent acellular bone-bioactivity. The hDPCs cultured on the Col/nBG nanocomposite have shown active growth behavior during culture for 14 days. The mRNA levels of major organic extracellular matrix of dentin, collagen type I and III were highly expressed in the Col/nBG matrix. Moreover, the alkaline phosphatase (ALP) activity and the mineralized nodule formation were increased in the Col/nBG nanocomposite compared to those in Col. Odontoblatic differentiation genes, including dentin sialophosphoprotein, dentin matrix protein I, ALP, osteopontin and osteocalcin were significantly stimulated in the Col containing nBG. Moreover, the key adhesion receptor integrin components α2 and β1, specifically binding to collagen molecule sequence, were upregulated in Col/nBG compared to Col, suggesting that odontogenic stimulation was closely related to the integrin-mediated process.
Significance
In our study, the nanocomposite Col/nBG matrix induced the growth and odontogenic differentiation more effectively than Col alone, providing a promising scaffold condition for regeneration of dentin–pulp complex tissue.
1
Introduction
Regenerative dentin and pulp could be potential tools for endodontic therapy, although technical problems need to be solved before they can be implemented in clinical applications . After tooth damage, dental pulp cells (DPCs) would be activated to migrate to the injury sites and differentiate into odontoblast-like cells, which synthesize and excrete an extracellular matrix (ECM) to form the reparative dentin . DPCs secrete type I collagen and other noncollagenous proteins, including osteocalcin (OCN), osteopontin (OPN), alkaline phosphatase (ALP), bone sialoprotein (BSP), dentin sialophosphoprotein (DSPP) and dentin matrix protein (DMP-1), which have been used as differentiation markers for odontoblast/osteoblast-like human DPCs .
Cells, bioactive molecules and supporting matrices are considered necessary for the regeneration of tissues including dentin through tissue engineering approach . These components must be carefully selected for successful regeneration of functional dentin, which protects pulp from external irritants. Dental pulp cells are frequently selected for the cellular component owing to their ability to produce dentin matrix . However, the specific growth factors or bioactive molecules that trigger mesenchymal stem cells to differentiate into odontoblasts have not been yet identified. Various scaffolding materials have been applied for regeneration of hard dental tissues, including degradable polymers (in synthetic or natural form), bioceramics (in crystalline or glass form) and their composites . Degradable synthetic polymers, mainly poly(alpha-hydroxyl acids), are easy to process and have adaptable mechanical flexibility; they largely lack cellular and biological activity, requiring cell triggering moieties on the surface and even mineralization-stimulating factors within the structure. In contrast, natural polymers, such as collagen, alginate and chitosan are considered better suited for use as cell matrices and scaffolds in terms of biological activity involving cell adhesion and population . In other biomaterial regime, bioactive ceramics have shown excellent biological activity in relation with tooth-derived cells, such as odontoblasts and dental pulp cells . Inorganic sources, such as calcium and phosphates, can also be a potent ionic stimulator for cellular differentiation and mineralization. Among others, the class of bioactive glasses (BGs) is considered osteoconductive and even osteoinductive, regulating genetic profiles of osteoprogenitor/stem cells down to the lineage of bone forming cells . However, bioactive ceramics, including BGs, have extremely poor mechanical properties, such as high brittleness, low tensile strength and are difficult to process, which primarily restricted their practical applications as scaffolds for dental tissue engineering. Therefore, the composite approach of using biopolymers in concert with bioactive ceramics is considered promising strategy to attain cell matrices with physic-chemical and biological properties appropriate for hard tissue engineering.
In this context, here we propose a novel nanocomposite scaffold made of collagen with incorporation of BG. In particular, the BG component was prepared in the form of nanofibers by utilizing the electrospinning process, as described previously . The nanofibrous form of the BG composition derived from a sol–gel process was shown to enable population of tissue cells and their stimulation into osteogenic differentiation of mesenchymal stem cells . The nanocomposite or hybrid approach can overcome the brittleness and poor shape availability of the inorganic nanocomponent, while it provides excellent bone-bioactivity and osteogenic potential to the polymeric phase . Our previous study on the nanocomposite of nBG with collagen demonstrated good organization between the glass nanofibrous network and collageneous fibrils . Moreover, shape forming, such as processing into porous foam and thin membrane, was facilitated using scaffolding/molding techniques. Furthermore, recent studies have demonstrated that sol–gel derived BG component enhanced osteoblastic differentiation of mesenchymal stem cells and osteoblasts . However, there is little information on the ability of the nBG or its composites with biopolymers including collagen in the responses related to dental pulp cells (DPCs), such as proliferation and odontogenic differentiation. It is considered that the nBG component should play some roles in the regulation of odontogenesis of DPCs while retaining a level of proliferative potential.
The aim of this study was thus to examine the in vitro response of human DPCs to collagen/nBG nanocomposite, which is useful for further applications in dentin–pulp tissue engineering. In vitro cell attachment, proliferation, odontogenic differentiation and extracellular matrix (ECM) molecule production on the collagen/nBG nanocomposite were evaluated in comparison with those on the pure collagen equivalent.
2
Materials and methods
2.1
Preparation of Col/nBG nanocomposites
For preparation of the bioactive inorganic nanocomponent, electrospinning process was applied, as described previously . Bioactive glass sol–gel solution (composition of 70% SiO 2 –25% CaO–5% P 2 O 5 in mol%) was contained in a syringe and then injected through the nozzle under constant electric field. A spinner jet started to form a non-woven nanofibrous mesh onto the metal collector. The nanofibrous mesh was further heat-treated at 700 °C to eliminate polymeric phase and stabilize the glass structure. The produced nanofibrous bioactive glass (nBG) had diameters of tens to hundreds of nanometers.
The nBG was dispersed in phosphate-buffered saline (PBS) solution and mixed with type I collagen (Col) solution at 3:1 by volume. After vortexing slightly, the mixture solution was incubated at 37 °C. During incubation, the collagen molecules were shown to reconstitute into fibrils, as evidenced by the turbidity change measured at 313 nm using UV–Vis spectrophotometer . The ratio of nBG and collagen was determined at 30:70 by weight. The reconstituted hybridized collagen/nBG solution was centrifuged at 5000 rpm for 6 min and the supernatant was discarded. The remnant was homogenized again by vortex, placed in a dish and then dried under laminar flow overnight, under sterile conditions, to form a thin nanocomposite membrane. Pure Col membrane was similarly prepared, without the incorporation of nBG. The dried membranes were further cross-linked with EDC/NHS (200 mM EDC and 150 mM NHS) in ethanol for 6 h, washed with ethanol/distilled water and dried under vacuum. The samples were sterilized in 70% ethanol and UV overnight and then kept in a refrigerator for further uses. The morphology of the hybridized membrane was observed by scanning electron microscopy (SEM; Hitachi).
2.2
Cell culture
Human dental pulp cells (hDPCs) immortalized by transfection with the telomerase catalytic subunit hTERT gene were used . Cells were cultured in Dulbecco’s Modified Eagle’s Medium (DMEM) supplemented with 10% fetal bovine serum, 100 U/ml penicillin and 100 mg/ml streptomycin in a humidified atmosphere of 5% CO 2 at 37 °C. For experiments, cells were cultured in odontogenic medium (OM), including 50 μg/ml ascorbic acid, 10 −7 M dexamethasone and 10 mM β-glycerophosphate, as described previously . The medium was changed every 2 days with freshly prepared medium. All experiments were done in triplicate.
2.3
Cell adhesion and proliferation
hDPCs were seeded on Col and nBG/Col hybrid at a density of 2 × 10 4 cells per well, and cultured in OM. After each culturing period (3, 7 and 14 days), the cells were harvested and the cell growth was measured by cell proliferation assay kit (CellTiter 96 Aqueous One Solution; Promega, Madison, WI). A colorimetric measurement was performed using a microplate reader at an absorbance of 490 nm.
After 3 days of incubation, the cell growth morphology was observed by SEM at accelerating voltage of 15 kV, after fixing with glutaraldehyde (2.5%), dehydrating with a graded series of ethanol (75%, 90%, 95% and 100%), treated with hexamethyldisilazane and Pt-coating.
2.4
Measurement of alkaline phosphatase (ALP) activity
Incubated hDPCs were washed with PBS and then sonicated with cell disruptor. ALP activity was measured using p -nitrophenyl phosphate (3 mM final concentration) as substrate, in 0.7 M 2-amino-methyl-1-propanol, pH 10.3, and 6.7 mM MgCl 2 . Absorbance was measured at 410 nm using an enzyme-linked immunosorbent assay reader (Beckman Coulter, Fullerton, CA).
2.5
RNA isolation and reverse transcriptase-polymerase chain reaction (RT-PCR)
The total RNA of the hDPCs was extracted using the Trizol reagent (Life Technologies, Gaithersburg, MD), according to the manufacturer’s instruction. RNA reverse transcription was performed at 42 °C for 1 h in a total volume of 20 μl containing 5 μg of total RNA; 0.5 mg of oligo (dT) 12–18 ; 10 mM DTT; 0.5 mM each of dATP, dGTP, dCTP, and dTTP; and 10 U of Superscript II reverse transcriptase. The specific primers are detailed in Table 1 . RT-PCR was performed with AccuPower RT PreMix kit (Bioneer, Daejon, Korea). Each reaction contained 0.5 μg of total RNA as template, 4 μl of 5× RT-PCR buffer, 0.8 μl of dNTP mix (400 μM), 1.2 μl of each primer (0.6 μM), 0.8 μl of enzyme mix, and RNase-free water in a final volume of 20 μl. After RT-PCR, aliquots (6 μl) of the RT-PCR products were electrophoresed through 1.5% agarose gels (Bio-Rad, Hercules, CA) containing 0.5 g/ml of ethidium bromide (Bio-Rad) and visualized under ultraviolet light and photographed.
Molecules | Primer sequences | Size (bp) | Annealing (°C) | Cycle | Extension time (s) |
---|---|---|---|---|---|
ALP (ALPP) | F:5′-ACGTGGCTAAGAATGTCATC-3′ | 476 | 55 | 30 | 30 |
[ NM_000478 ] | R:5′-CTGGTAGGCGATGTCCTTA-3′ | ||||
OPN (SPP1) | F:5′-CCAAGTAAGTCCAACGAAAG-3′ | 348 | 55 | 35 | 30 |
[ NM_001251830 ] | R:5′-GGTGATGTCCTCGTCTGTA-3′ | ||||
OCN (BGLAP) | F:5′-CATGAGAGCCCTCACA-3′ | 315 | 55 | 35 | 30 |
[ NM_199173 ] | R:5′-AGAGCGACACCCTAGAC-3′ | ||||
DSPP (DSPP) | F:5′-GCAGTGATGAATCTAATGGC-3′ | 488 | 54 | 35 | 60 |
[ NM_014208 ] | R:5′-CTGATTTGCTGCTGTCTGAC-3′ | ||||
DMP-1 (DMP1) | F:5′-ACACCACCCTTGGCAAATGA-3′ | 382 | 60 | 35 | 60 |
[ NM_001079911 ] | R:5′-GAGTGGAGTCACCTCCCTCA-3′ | ||||
COLIA1 (COL1A1) | F:5′-AATCCCTTGTGCCGCAGGGC-3′ | 527 | 60 | 30 | 60 |
[ NM_000088 ] | R:5′-CGGGGACAGAGGACGCAGGA-3′ | ||||
COLIA2 (COL1A2) | F:5′-GGATGCGGAGGGCGGAGGTA-3′ | 346 | 65 | 30 | 60 |
[NM_000089] | R:5′-GGCATGCAGTCGTGGCCAGT-3′ | ||||
COLIIIA1 (COL3A1) | F:5′-TGGCGTCCCTGGAGGTCCAG-3′ | 290 | 65 | 35 | 60 |
[ NM_000090 ] | R:5′-CAGTAGGCCCTGGGGGTCCC-3′ | ||||
Integrin α2 (ITGA2) | F:5′-TTTCCCTGCTCTCACCGGGC-3‘ | 132 | 64 | 33 | 60 |
[ NM_002203 ] | R:5′-ACCGGGGGACCGTAGTTGCG-3′ | ||||
Integrin β1 (ITGB1) | F:5′-GACGCCGCGCGGAAAAGATG-3‘ | 159 | 60 | 33 | 60 |
[ NM_002211 ] | R:5′-ACCACCCACAATTTGGCCCTGC-3′ | ||||
GAPDH (GAPDH) | F:5′-CGGAGTCAACGGATTTGGTCGTAT-3′ | 307 | 61 | 35 | 30 |
[ NM_002046 ] | R:5′-AGCCTTCTCCATGGTGGTGAAGAC-3′ | ||||
HPRT1 (HPRT) | F:5′-AGGACTGAACGTCTTGCTCG-3′ | 388 | 58 | 35 | 60 |
[NM_00194] | R:5′-ATCCAACACTTCGTGGGGTC-3′ |
2.6
Mineralization assay
Mineral nodule formation was detected by Dahl’s method for calcium. Cells were placed in a 24 well plate at a density of 1 × 10 5 cells per well and cultured in OM with either Col or nano-BG hybrid for 2 weeks. The cells were fixed in 3.7% formaldehyde neutral buffer solution and then stained with alizarin red S.
2.7
Statistical analysis
Data are presented as mean ± standard deviation (SD). Statistical analysis was performed by one-way analysis of variance (ANOVA) followed by multiple comparison Tukey’s test, using the SPSS program (SPSS version 12.0; SPSS GmbH, Munich, Germany). Statistical significance was set at p < 0.05.
2
Materials and methods
2.1
Preparation of Col/nBG nanocomposites
For preparation of the bioactive inorganic nanocomponent, electrospinning process was applied, as described previously . Bioactive glass sol–gel solution (composition of 70% SiO 2 –25% CaO–5% P 2 O 5 in mol%) was contained in a syringe and then injected through the nozzle under constant electric field. A spinner jet started to form a non-woven nanofibrous mesh onto the metal collector. The nanofibrous mesh was further heat-treated at 700 °C to eliminate polymeric phase and stabilize the glass structure. The produced nanofibrous bioactive glass (nBG) had diameters of tens to hundreds of nanometers.
The nBG was dispersed in phosphate-buffered saline (PBS) solution and mixed with type I collagen (Col) solution at 3:1 by volume. After vortexing slightly, the mixture solution was incubated at 37 °C. During incubation, the collagen molecules were shown to reconstitute into fibrils, as evidenced by the turbidity change measured at 313 nm using UV–Vis spectrophotometer . The ratio of nBG and collagen was determined at 30:70 by weight. The reconstituted hybridized collagen/nBG solution was centrifuged at 5000 rpm for 6 min and the supernatant was discarded. The remnant was homogenized again by vortex, placed in a dish and then dried under laminar flow overnight, under sterile conditions, to form a thin nanocomposite membrane. Pure Col membrane was similarly prepared, without the incorporation of nBG. The dried membranes were further cross-linked with EDC/NHS (200 mM EDC and 150 mM NHS) in ethanol for 6 h, washed with ethanol/distilled water and dried under vacuum. The samples were sterilized in 70% ethanol and UV overnight and then kept in a refrigerator for further uses. The morphology of the hybridized membrane was observed by scanning electron microscopy (SEM; Hitachi).
2.2
Cell culture
Human dental pulp cells (hDPCs) immortalized by transfection with the telomerase catalytic subunit hTERT gene were used . Cells were cultured in Dulbecco’s Modified Eagle’s Medium (DMEM) supplemented with 10% fetal bovine serum, 100 U/ml penicillin and 100 mg/ml streptomycin in a humidified atmosphere of 5% CO 2 at 37 °C. For experiments, cells were cultured in odontogenic medium (OM), including 50 μg/ml ascorbic acid, 10 −7 M dexamethasone and 10 mM β-glycerophosphate, as described previously . The medium was changed every 2 days with freshly prepared medium. All experiments were done in triplicate.
2.3
Cell adhesion and proliferation
hDPCs were seeded on Col and nBG/Col hybrid at a density of 2 × 10 4 cells per well, and cultured in OM. After each culturing period (3, 7 and 14 days), the cells were harvested and the cell growth was measured by cell proliferation assay kit (CellTiter 96 Aqueous One Solution; Promega, Madison, WI). A colorimetric measurement was performed using a microplate reader at an absorbance of 490 nm.
After 3 days of incubation, the cell growth morphology was observed by SEM at accelerating voltage of 15 kV, after fixing with glutaraldehyde (2.5%), dehydrating with a graded series of ethanol (75%, 90%, 95% and 100%), treated with hexamethyldisilazane and Pt-coating.
2.4
Measurement of alkaline phosphatase (ALP) activity
Incubated hDPCs were washed with PBS and then sonicated with cell disruptor. ALP activity was measured using p -nitrophenyl phosphate (3 mM final concentration) as substrate, in 0.7 M 2-amino-methyl-1-propanol, pH 10.3, and 6.7 mM MgCl 2 . Absorbance was measured at 410 nm using an enzyme-linked immunosorbent assay reader (Beckman Coulter, Fullerton, CA).
2.5
RNA isolation and reverse transcriptase-polymerase chain reaction (RT-PCR)
The total RNA of the hDPCs was extracted using the Trizol reagent (Life Technologies, Gaithersburg, MD), according to the manufacturer’s instruction. RNA reverse transcription was performed at 42 °C for 1 h in a total volume of 20 μl containing 5 μg of total RNA; 0.5 mg of oligo (dT) 12–18 ; 10 mM DTT; 0.5 mM each of dATP, dGTP, dCTP, and dTTP; and 10 U of Superscript II reverse transcriptase. The specific primers are detailed in Table 1 . RT-PCR was performed with AccuPower RT PreMix kit (Bioneer, Daejon, Korea). Each reaction contained 0.5 μg of total RNA as template, 4 μl of 5× RT-PCR buffer, 0.8 μl of dNTP mix (400 μM), 1.2 μl of each primer (0.6 μM), 0.8 μl of enzyme mix, and RNase-free water in a final volume of 20 μl. After RT-PCR, aliquots (6 μl) of the RT-PCR products were electrophoresed through 1.5% agarose gels (Bio-Rad, Hercules, CA) containing 0.5 g/ml of ethidium bromide (Bio-Rad) and visualized under ultraviolet light and photographed.
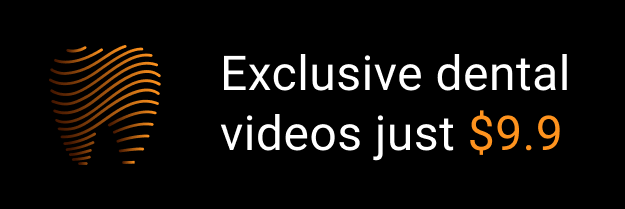