Highlights
- •
CaAl and MgAl LDH dental composites were developed with varying LDH loading.
- •
Fluoride absorption/release cycles were studied (five recharges) in DW and AS.
- •
Water uptake, solubility, cation release, and mechanical properties were studied.
- •
LDH-composites repeatedly absorbed/released fluoride maintaining a sustained release.
- •
Physico-mechanical properties of composites were maintained with LDH-composites.
Abstract
Objective
This study aims to incorporate 2:1 MgAl and 2:1 CaAl layered double hydroxides (LDHs) in experimental dental-composites to render them fluoride rechargeable. The effect of LDH on fluoride absorption and release, and their physico-mechanical properties are investigated.
Methods
2:1 CaAl and 2:1 MgAl LDH-composite discs prepared with 0, 10 and 30 wt% LDH were charged with fluoride (48 h) and transferred to deionized water (DW)/artificial saliva (AS). Fluoride release/re-release was measured every 24 h (ion-selective electrodes) with DW/AS replaced daily, and samples re-charged (5 min) with fluoride every 2 days. Five absorption-release cycles were conducted over 10 days. CaAl and MgAl LDH rod-shaped specimens (dry and hydrated; 0, 10 and 30 wt%) were studied for flexural strength and modulus. CaAl and MgAl LDH-composite discs (0, 10, 30 and 45 wt% LDH) were prepared to study water uptake (over 7 weeks), water desorption (3 weeks), diffusion coefficients, solubility and cation release (ICP-OES).
Results
CaAl LDH and MgAl LDH-composites significantly increased the amount of fluoride released in both media ( P < 0.05). In AS, the mean release after every recharge was greater for MgAl LDH-composites compared to CaAl LDH-composites ( P < 0.05). After every recharge, the fluoride release was greater than the previous release cycle ( P < 0.05) for all LDH-composites. Physico-mechanical properties of the LDH-composites demonstrated similar values to those reported in literature. The solubility and cation release showed a linear increase with LDH loading.
Significance
LDH-composites repeatedly absorbed/released fluoride and maintained desired physico-mechanical properties. A sustained low-level fluoride release with LDH-composites could lead to a potential breakthrough in preventing early stage carious-lesions.
1
Introduction
The benefit of fluoride for controlling caries particularly in children and adolescents is widely known . The potential application of fluoride released at low levels (∼0.025–2 ppm), from dental materials in the oral environment, is the most effective method in preventing post-eruptive dental caries . The preventative action is due to the inhibition of demineralization, promotion of remineralization and, inhibition of bacterial growth and metabolism . Due to the similar size of fluoride ions (1.36 Å) and hydroxide ions (OH − = 1.40 Å), fluoride ions are able to exchange with hydroxide ions in hydroxyapatite to form fluorapatite, which is more resistant to dissolution by an acid challenge .
Many studies have demonstrated the benefits of fluoride at a low concentration. As an example, Lynch, Navada reported this effect in vitro with human teeth, by exposing them to a pH-cycling system while varying the fluoride concentration (0.009, 0.014, 0.025, 0.2 and 2.0 ppm). Significant changes in the reduction of demineralization, determined through calcium released in acetic acid and image analysis of the exposed tooth section were observed. A small increase in fluoride concentration, from 0.009 to 0.2 ppm subsequently approached a plateau after increasing to 2 ppm. Furthermore, a 2-year clinical trial involving 174 children (mean age 8 years old) was reported by Toumba and Curzon . A slow releasing fluoride dental device, in the form of a glass pellet, was attached to the tooth. The results showed an increase in the salivary fluoride concentration from 0.03 ppm (control, physiological concentration) to 0.11 ppm and a 67% decrease in carious teeth after 2 years. In addition, Fan et al. showed that acid-etched enamel surfaces immersed in 1 ppm fluoride for 16 h, produced a needle-like structure, indicating the formation of fluorapatite, which was further confirmed using pXRD.
Numerous consumer healthcare companies provide dentists with fluoride containing dental materials such as dental composites, fluoride varnishes, fissure sealants, glass ionomer cements (GICs) and resin modified glass ionomer cements (RMGICs) that are capable of releasing fluoride over time, in the oral cavity. However, fluoride release from these materials is not always well controlled and diminishes over time. Moreover, GICs have been reported to show an initial burst of fluoride, in a range from 5 to 155 ppm, within the first 24 h, depending on the different brands available . Although GICs have shown fluoride-recharging capabilities, the amount of fluoride re-released does not reach the initial concentration and substantially less is released with each successive recharge . It is worth acknowledging that high concentrations of fluoride in fluoridated water may lead to fluorosis, which causes mottling of enamel and may be esthetically unpleasing . A 1-year study conducted on 18,755 children in the United States, with varying fluoride concentrations in drinking water, showed that an ideal fluoride concentration between caries prevention and fluorosis was around 0.7 ppm .
Fluoride is incorporated into composite resins in several forms such as water soluble salts (e.g. sodium fluoride, NaF), leachable glasses and/or matrix bound fluoride . However, a study investigating the release of fluoride over 16 weeks (in 7 ml water, artificial saliva and lactic acid) from composites (containing a sparingly soluble ytterbium trifluoride [YbF 3 ] salt), GICs and RMGICs, reported that fluoride release was significantly lower from composites. Also, the release of fluoride from composites containing fluoride salts resulted in voids in the structure (as the salts dissolved), which affected the mechanical properties such as wear resistance . Composite resins also showed a burst of fluoride release, however it was less pronounced than from RMGICs and GICs. Yap et al. , who investigated a range of dental fluoride releasing materials, demonstrated that composites (containing fluoride leachable glass) released less fluoride compared to GICs and RMGICs. Composites released 1.44 ppm within the first 24 h, which reduced to 0.22 ppm by day 7 (replacing 1 ml of DW every 24 h), GIC released 8.78 ppm and RMGIC released 7.19 ppm fluoride in the first 24 h, which reduced to 1.51 and 3.18 ppm fluoride, respectively. Attar and Önen studied fluoride release from commercial composite materials (Dyract, Dentsply, Germany containing strontium-aluminum-fluoro-silicate-glass and Tetric, Vivadent, Liechtenstein containing YbF 3 ) over 60 days, and demonstrated a release of 0.04–0.55 ppm in the first 24 h (in 4 ml of de-ionized water); by the 60th day 0.02–0.03 ppm of fluoride was released. Even at this low fluoride concentration, fluoride containing composites have been shown to prevent the formation of secondary caries in vitro, in a 1 month study examining enamel demineralization . The in vitro study also demonstrated that the composite materials were unable to recharge with fluoride. A satisfactory method for maintaining a sustained low concentration of fluoride in the oral environment is as yet an unsolved problem. This issue will therefore be the focus of this present paper. To achieve this, a material incorporating layered double hydroxides (LDH) will be investigated, which will also render it as fluoride rechargeable.
Layered double hydroxides, also known as hydrotalcites, consist of positively charged inorganic sheets, counterbalanced by negatively charged anions e.g. fluoride, chloride, carbonates etc. . These structures have been successfully proven to remove excess fluoride from drinking water . They are also biocompatible since they have been studied in biological applications for controlled drug delivery. They are currently incorporated in commercially available antacids and antipeptics, known as Talcid™ and Altacite™, respectively, to neutralize the acidic environment . The general formula for LDH is [M 1− X 2+ ·M x 3+ ·(OH) 2 ][ A · X /n n −· m H 2 O], consisting of divalent, M 2+ (Mg, Zn, Ni, Co, Mn, etc.) and trivalent cations, M 3+ (Al, Cr, Fe, Co, etc.) in the positive sheets .
Only a limited number of studies have reported the use of LDH (2:1 MgAl LDH) for dental applications. Perioli et al. performed a clinical study where LDH containing fluoride (at 1–4% wt./wt) was incorporated into a hydrophilic buccal mucoadhesive (2 cm 2 ) patch. This patch was attached to the gum of five healthy volunteers. Residence time, swelling capacity, salivary modification, fragment loss, acceptability and organoleptic properties were evaluated in vivo. These LDH patches released fluoride in vitro at a controlled rate, over 4 h, in 100 ml of water containing 1.2 mM NaHCO 3 at 37 ± 0.1 °C. These results showed that fluoride release increased with an increase in LDH loading from 1 to 4 wt%. A further study by Yokogawa et al. , investigated the release of fluoride from 0.1 g of 2.7:1 MgFe LDH powder alone (without incorporating into a matrix), immersed into hydrogen sulfide (H 2 S), for absorption of volatile sulfur compounds (VSC) to reduce halitosis (malodour). This LDH released 8 ppm fluoride and absorbed VSC completely over 8 h.
Calarco et al. incorporated 2:1 MgAl LDH into a commercial resin composite and demonstrated fluoride release over 3 weeks, which was compared to a fluoride glass-filled commercial dental resin. A lower, but controlled release rate of fluoride was achieved by the LDH-composite in comparison to the fluoride glass-filled composite (burst release). The former system increased the migratory response of human dental pulp stem cell subpopulation (STRO-1+) and, indicated a complete odontoblast-like cell differentiation, an effect that was not observed with the fluoride glass-filled composite. Tammaro et al. pre-charged MgAl LDH powder with fluoride and incorporated this into a commercial resin composite as a filler, which improved the resin’s mechanical properties. However, the latter were only investigated prior to fluoride release (dry samples), and not after immersing in de-ionized water following fluoride release; this may have had an adverse effect on the materials properties, as it has been demonstrated that LDH also absorbs water within its interlayers . In vitro studies have also shown differentiation and proliferation of human dental pulp stem cells (hDPSC). The authors claimed this was due to the release of fluoride at low concentrations (0.25–5 ppm) over the study period . The authors did not recharge the LDH-composite with fluoride, and hence, it appears they did not incorporate LDH for recharging purposes, but merely as a filler that releases fluoride.
Recently, a study by Su et al. incorporated fluoride charged LiAl LDH at 3 and 5 wt% into dental composites (RX; Esthet-X Flow and CC; Dyract flow, Dentsply, USA) and investigated fluoride release in 3 ml of DW over 90 days, with a recharge (1000 ppm NaF solution 4 min) at day 30. After fluoride recharge, the fluoride release was increased by 0.07–0.2 ppm with the 5 wt% LDH containing composite. The incorporation of LDH in commercial materials masks the effect of the LDH alone, since these materials also contain other sources of fluoride. Therefore, it is essential to incorporate LDH in an experimental dental composite of known composition. In addition, DW alone does not mimic the oral environment and therefore fluoride absorption and release experiments should be carried out in AS, which mimics the oral environment. There is also a requirement to assess whether the amount of fluoride released after every recharge is maintained by the LDH-composite and does not diminish as reported for GICs. These requirements fall within the scope of the research reported in this paper.
This present study aims to investigate the potential of MgAl and CaAl LDH incorporated into experimental composites, thus rendering them fluoride rechargeable, in DW and AS. These materials would act as dental LDH-composite fluoride reservoirs, with the potential of preventing early-stage carious lesions and secondary caries. The effect of the LDH on the composites physico-mechanical properties will also be assessed to understand whether the properties are maintained or enhanced for use as dental composites e.g. restorative materials.
2
Materials and methods
2.1
Materials
Calcium chloride dihydrate (CaCl 2 ·2H 2 O; Sigma-Aldrich, UK), magnesium chloride (MgCl 2 ; Sigma-Aldrich, UK) and aluminum chloride (AlCl 3 ; Fluka Analytical) reagents, with a >99% purity, were used to produce LDH (see below). Fluoride absorption and release (over six cycles) was also compared from two commercial resin composites (Tetric and Tetric EvoCeram from Ivoclar Vivadent, Lichtenstein). These commercial products were selected as they closely matched the experimental composite matrix (see below). However, both commercial products additionally contained fillers (81 wt%). The full composition can be observed in Supplementary material).
2.2
Immersion media
Deionized water (DW) and artificial saliva (20 mM N -2-hydroxyethylpiperazine- N -2-ethanesulfonic acid [HEPES], 1.50 mM CaCl 2 ·2H 2 O, 0.90 mM potassium dihydrogen orthophosphate, 130 mM potassium chloride, pH 7.0) adopted from Lynch & ten Cate was used as immersion media.
2.3
Methods
2.3.1
LDH powder synthesis
Two LDH powders (2:1 calcium aluminum [CaAl LDH] and 2:1 magnesium aluminum [MgAl LDH]) were produced using a co-precipitation method which was adopted from Mandal & Mayadevi . The LDH powders were co-precipitated (at room temperature; 21 ± 0.1˚C) using a solution of metal chlorides with a 2:1 divalent (Mg 2+ or Ca 2+ ) to trivalent (Al 3+ ) cations ratio; 0.667 M concentration CaCl 2 (or MgCl 2 ) and 0.333 M AlCl 3 aqueous solution at pH 10 ± 1 for MgAl LDH and 11.5 ± 1 for CaAl LDH. The pH for each LDH was maintained with the dropwise addition of 2 M sodium hydroxide (NaOH). The precipitate was aged for 24 h at room temperature, washed and centrifuged several times with DW until a neutral solution was obtained (using litmus paper) and then dried at 80 °C for 36 h. The solid powder was ground with a mortar and pestle and sieved using a 63 μm analytical sieve (Endecotts, Ltd, London, UK), for 45 min on a Retsch VS1000 vibrating machine (Retch GmbH, Germany).
2.3.2
Composite sample preparation
Light-curable experimental composites were produced from a prepared mixture of bisphenol A-diglycidyl methacrylate (Bis-GMA), urethane dimethacrylate (UDMA) and triethylenglycol dimethacrylate (TEGDMA) (35/35/30 wt%), containing N , N -dimethyl- p -toluidine and camphorquinone (Sigma-Aldrich, UK). Seven mixtures were prepared; a control containing no LDH and three loadings (10, 30 and 45 wt%) of each LDH (2:1 CaAl and 2:1 MgAl). The refractive index of LDH (1.510–1.518) also closely matches the refractive index of the monomers used in the experimental composite (1.4703–1.5370), therefore a minimal effect on the curing depth of the LDH-composite can be considered .
For fluoride absorption and release, circular discs of two loadings (10 and 30 wt%) of each LDH (2:1 CaAl and 2:1 MgAl) and an experimental resin control were studied (tested in DW and AS; n = 6, 60 samples in total). A 45 wt% incorporation of LDH was not investigated since the water uptake studies showed unfavorable properties at this higher concentration (see section below). Two commercial composites (Tetric and Tetric Evoceram, Ivoclar Vivadent, Lichtenstein) were also studied in DW only ( n = 6), which were part of a previous pilot study and used for comparison purposes.
For water uptake studies, seven LDH mixtures (three loadings × two LDHs: 10, 30 and 45 wt% and 0% control; n = 6, 42 samples in total) were used to prepare circular discs (measuring 1 mm × 10 mm)
For mechanical properties (according to the ISO 4049:2009 specifications ), rod-shaped samples were produced measuring 25 mm × 2.5 mm × 2.5 mm using 10 and 30 wt% of each LDH (2:1 CaAl and 2:1 MgAl) and control (tested dry and hydrated, n = 10, 100 samples in total). Half of the samples were hydrated in 50 ml DW (37 °C in an incubator shaker) for 2 weeks prior to testing.
For all studies, one sample was made at a time using a polytetrafluoroethylene (PTFE) mold. The mold was slightly overfilled with each mixture and was supported on both sides by glass slides covered with acetate sheets, the latter to prevent the resin from sticking to the glass slides. Whilst applying pressure over the slides for the circular discs, the samples were cured for 20 s using a light curing unit (3 M ESPE EliparTM, USA; wavelength: 430–480 nm, 455 nm peak with 1200 mW/cm 2 light intensity) on one side to complete curing (to mimic the materials’ chairside application). For the rod-shaped samples, the samples were cured three times (slightly overlapping the light curing area each time) along the length of the specimen, in order to cure the whole length of the specimen. All samples were visually inspected and any sample with voids or defects was removed. All sample edges were smoothed using silicon carbide abrasive paper (P600) (Buehler, IL, USA) to remove any flash or irregularities. Fig. 1 shows a flow chart of the work conducted with the LDH-composites in this report.

2.3.3
Fluoride absorption and release
Each LDH-composite disc and commercial composite was immersed separately in 15 ml of 0.1 M sodium fluoride (NaF) solution in an incubator shaker (37 °C and 60 rotations per minute, rpm) for two days (48 h) to absorb fluoride. The disc was then removed, blotted dry and transferred to 5 ml of DW or AS (37 °C and 60 rotations per minute, rpm) for 24 h. The amount of fluoride released in the immersion solution was measured every 24 h, using fluoride ion selective electrodes (NICO2000), following the addition of a total ionic strength adjustment buffer (TISAB, 5 ml) to break down any potential complexes formed in solution e.g. CaF 2 or AlF 3 . The immersion solution was then replaced with fresh DW or AS (every 24 h), to avoid saturation. After two days (48 h) of release the disc was immersed into a tube containing 15 ml NaF solution (0.05 M; 37 °C and 60 rotations per minute, rpm) to absorb fluoride (recharge), for 5 . The disc was then removed, blotted dry and placed back into fresh DW or AS (5 ml) to re-release fluoride. Fluoride recharge cycles were performed over 10 days (re-charging every 48 h), with two days of fluoride release between the recharges.
2.3.4
Water uptake
A similar method to that used by Agha et al. was used to conduct this study. All composite discs were firstly conditioned (dried) at 37 ± 1 °C in an incubator (Carbolite, Camlab, Cambridge, UK) over 72 h and then weighed separately (time 0, W 0 ) and immersed in 100 ml DW at 37 ± 1 °C. At regular pre-determined time intervals over 7 weeks the samples were removed from DW, blotted dry on filter paper (Fisherbrand, USA) and weighed ( W t ) to an accuracy of 0.0001 g (Mettler HK balance, USA), before returning to the bottle with DW in the oven. Several readings were recorded on the first day and then less frequently over 7 weeks. Each sample was weighed in less than 30 s to avoid any dehydration of the samples. The weight change (%) at each time point was calculated using Eq. (1) . W t was the weight at the time interval ( t ) and W 0 was the initial weight at time zero (time 0).
Weightchange(%uptake)=(Wt−W0/W0)×100.
The mean weight change was then plotted against time 1/2 ( t 1/2 in s 1/2 ), with standard deviations for each interval.
2.3.5
Desorption, diffusion coefficient, solubility and cation release
After 7 weeks of studying water uptake, the samples were removed from the solutions, blotted dry, weighed and transferred to a desorption oven (Carbolite, Camlab, Cambridge, UK) at 37 ± 1 °C. Similar to the water uptake method, the weight change (%) was calculated over 3 weeks and plotted against time 1/2 . W 0 was the weight at time 0 (at week 7 of absorbing water), and W d represents the constant minimum weight reached.
The solubility of the material was calculated by subtracting the weight after desorption ( W d ) from the initial weight ( W 0 ) and dividing by W 0 (Eq. (2) ).
Solubility(%)=(W0−Wd/W0)/100.
For calculating the diffusion coefficients for the absorption (speed at which water enters the sample before equilibrating) and desorption (speed at which water is lost from the sample before equilibrating) processes, the weight change data for both were plotted in the form of M t / M ∞ versus the square root of time (s). Where M t is the weight at each time point, t (in s) and M ∞ is the weight at equilibrium (i.e. where the linear part of % weight change versus t 1/2 plots begin to equilibrate. Eq. (3) was used to calculate the diffusion coefficients for the absorption and desorption processes:
D=(S2πL2)/4,
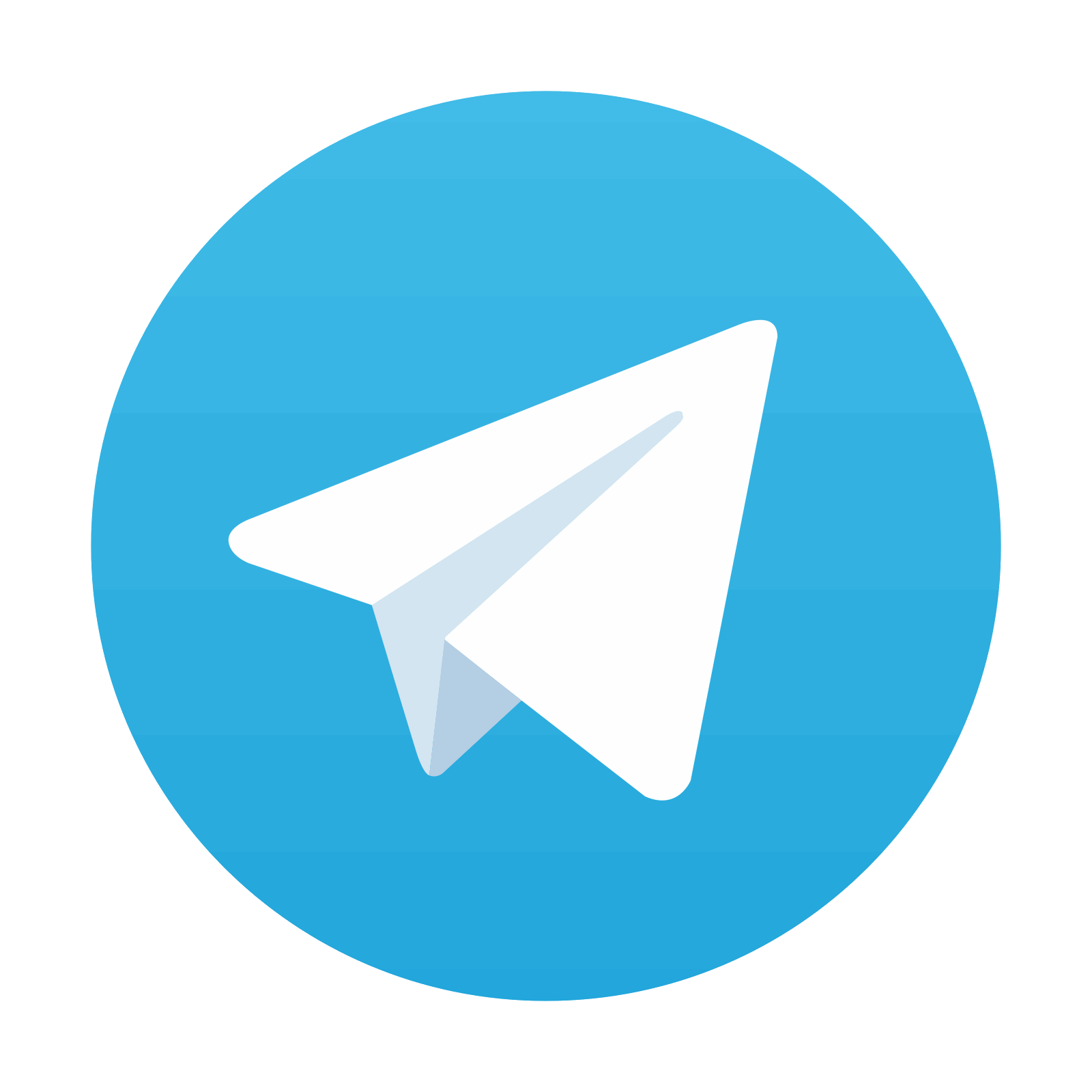
Stay updated, free dental videos. Join our Telegram channel

VIDEdental - Online dental courses
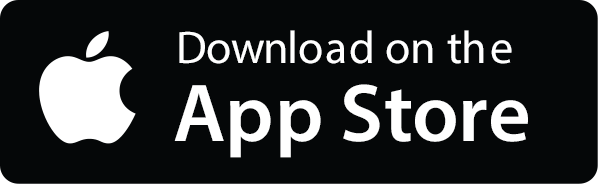
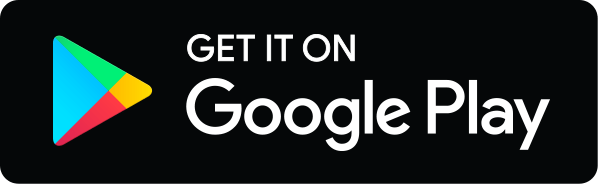
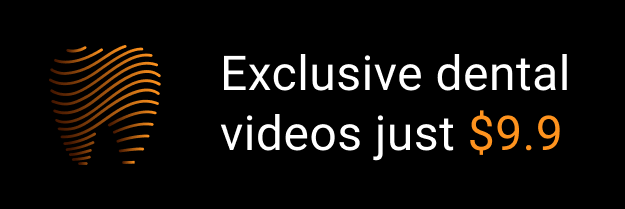