Fig. 9.1
Diagrammatic depiction of a cross-sectional view of the peripheral trigeminal nerve internal anatomy (From Steed [34]; with permission)
The outer connective tissue layer of the nerve is the external epineurium, which is a supporting and protective connective tissue made up primarily of collagen and elastic fibers (Fig. 9.2). The internal epineurium is the structure that invests the fascicles, which contain the nerve fibers themselves. Usually several fascicles are grouped together in bundles, constituting well-defined subunits of the nerve trunk. Fascicles vary in their size and quantity depending primarily on whether the region in question is at the proximal or distal site of the nerve. Both the lingual and inferior alveolar nerves are polyfascicular. The lingual nerve contains 15–18 fascicles at the region adjacent the mandibular third molar, while the inferior alveolar nerve contains 18–21 fascicles within the angle of the mandible. There may be fewer fascicles contained in the lingual nerve in the third molar region.
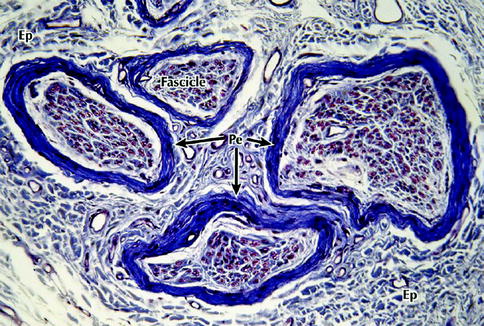
Fig. 9.2
Light micrograph of a peripheral nerve in transverse section. Several fascicles that make up this nerve are enveloped by the connective tissue of the epineurium (Ep) that merges imperceptibly with the surrounding loose connective tissue – the mesoneurium. A more deeply stained perineurium (Pe) encloses the fascicles. Each fascicle consists of a large number of nerve fibers that are embedded in a more delicate endoneurium (not well defined at this level of magnification), 200×, Masson trichrome (From Steed [34]; with permission)
Each fascicle is surrounded by a perineurium, a lamellated sheath with considerable tensile mechanical strength and elasticity (Fig. 9.3). The perineurium is made up of collagen fibers dispersed among perineural cells and acts as a diffusion barrier as a result of its selective permeability, maintaining the endoneurial space within it from the surrounding tissues. This preserves the ionic environment within the fascicle. The nerve fibers themselves are closely packed together within endoneurial connective tissue (endoneurium) inside of each fascicle (Figs. 9.4 and 9.5). The endoneurium is composed of a loose gelatinous collagen matrix.
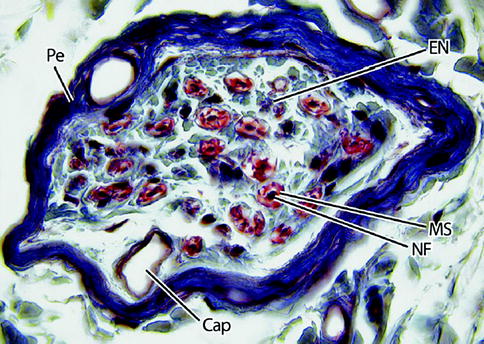
Fig. 9.3
Light micrograph of a nerve fascicle at higher magnification. Here, the perineurium (Pe) is dark blue and the endoneurium (EN) light blue. Nerve fibers (NF) are densely stained structures surrounded by a myelin sheath (MS), which is red. A capillary (Cap) is shown. 465×. Masson trichrome (From Steed [34]; with permission)
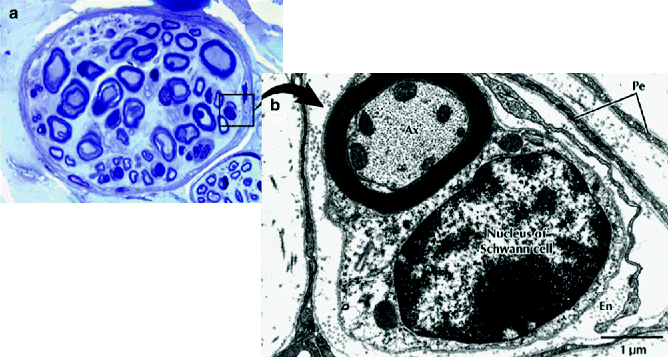
Fig. 9.4
Light micrograph of a peripheral nerve fascicle in transverse section. (a) Osmium fixation shows well-preserved myelin sheathes of nerve fibers. Nerve fibers vary in diameter and perineurium (Pe) surrounds the fascicle (toluidine blue, magnification ×600; semithin plastic section). (b) Electron micrograph of a myelinated nerve fiber and its associated Schwann cell in transverse section. The myelinated nerve fiber axoplasm (Ax) contains cytoskeletal elements and mitochondria that parallel its long axis. The Schwann cell, sectioned at the level of its nucleus, is enveloped by a basal lamina. Flattened perineurial cells (Pe) and collagen fibers of the endoneurium (En) are also seen (magnification ×16,800) (From Steed [34]; with permission)
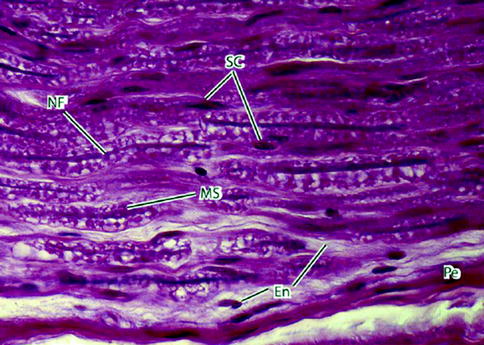
Fig. 9.5
Light micrograph of peripheral nerve in longitudinal section. Nerve fibers (NFs) – the slender deeply stained threads – pursue a wavy course. Myelin sheaths (MSs) appear vacuolated because of high lipid content and the effects of paraffin embedding on the tissue sample. Schwann cells (SCs) have elongated nuclei. They are indistinguishable from nuclei of fibroblasts of the delicate endoneurium (En) that invests the individual nerve fibers. A deeply stained perineurium (Pe) surrounds the nerve fascicle externally. 700×. H&E (From Steed [34]; with permission)
9.2.2 Blood Supply
Each neuron’s axon requires a continuous energy supply for impulse transmission and axonal transport. This is provided by an extrinsic vascular system and an intrinsic vascular system, which are interconnected. The extrinsic vessels enter the mesoneurium and communicate with the epineurial space via the vasa nervorum. A plexus develops at this level and runs longitudinally (Fig. 9.6). If the fascicles are examined closely, a large number of epineurial vascular branching is seen, supplying each fascicle in a segmental manner, so that each fascicle is vascularly analogous to a complete axon in a miniature model.
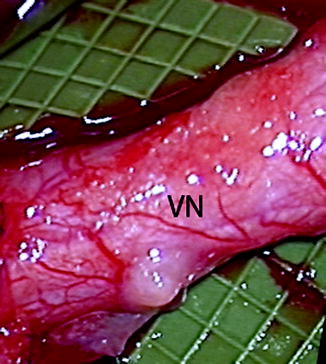
Fig. 9.6
Clinical photo of a lingual nerve under microscopic magnification demonstrating the vasa nervorum (VN). Large longitudinally oriented intrinsic epineurial arteriolar and venular vessels can be seen deep to this plexus (From Steed [34]; with permission)
The vascular plexus enters the endoneurium through the perineurium at an oblique angle to anastomose with the intrinsic circulation that surrounds each fascicle. The oblique passage of vessels through the inner perineurial membrane is a site of potential circulatory compromise within the intrafascicular tissue.
9.2.3 Nerve Fibers
A neuron consists of a nerve cell body and its processes. There are a number of dendrites associated with the cell body and one long extension – an axon, which travels to an end organ with branches terminating in peripheral synaptic terminals. Nerve fibers can be either myelinated or unmyelinated. Sensory and motor nerves contain both types of fibers in a ratio of 4 unmyelinated axons to 1 myelinated axon.
Unmyelinated fibers are made up of several axons enclosed by a single Schwann cell (Fig. 9.7). Unmyelinated axons are small in diameter, usually averaging 0.15–2.0 μm. The axons of a myelinated fiber are individually wrapped by a single Schwann cell that has laid down a laminar myelin sheath (Fig. 9.8). The center of a myelinated fiber is made up of cytoplasm (axoplasm) with associated cytoskeletal elements surrounded by a membrane (axolemma). A concentric sheath of myelin and a Schwann cell surround this membrane (Fig. 9.9). A thin basal lamina invests the interdigitating processes of Schwann cells. At the junction between two Schwann cells, the axolemma becomes exposed at a gap called a node of Ranvier (Fig. 9.10). The propagation of an action potential along the axon “jumps” from node to node over the insulated areas covered with myelin in the process of saltatory conduction. This provides for more rapid propagation along the axon. As a result, myelinated fibers are able to conduct an impulse at a speed up to 150 m/s, while unmyelinated fibers propagate impulses at speeds of 2–2.5 m/s.
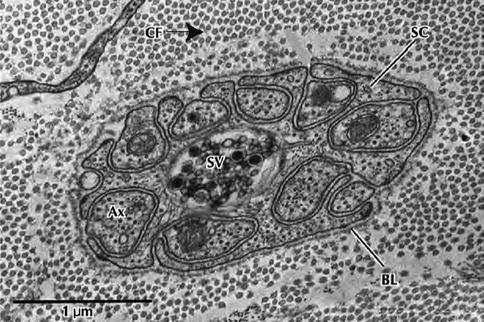
Fig. 9.7
Electron micrograph of a Schwann cell associated with several unmyelinated nerve fibers in transverse section. Nerve fibers (Ax) occupy channel-like invaginations of Schwann cell cytoplasm (SC). Most nerve fibers contain neurofibrils and microtubules; synaptic vesicles (SV); collagen fibrils (CF); basal lamina (BL) (From Steed [34]; with permission)
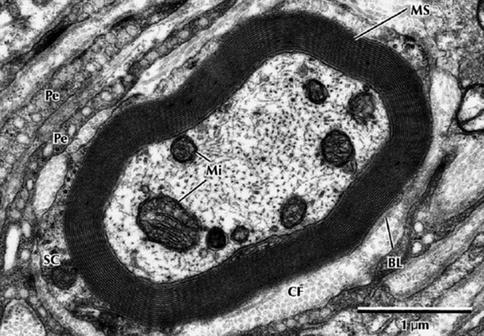
Fig. 9.8
Electron micrograph of a myelinated peripheral nerve fiber in transverse section. The axon is surrounded by a myelin sheath (MS) composed of multiple lamellae formed by the plasma membrane of a Schwann cell. A thin rim of Schwann cell cytoplasm (SC) envelops the myelin and is invested externally by a thin basal lamina (BL). Collagen fibers (CFs) of the endoneurium and flattened perineurial cells (Pe) are in the surrounding area. The nerve axoplasm contains mitochondria (Mi), neurofilaments, and a few microtubules. 30,000× (From Steed [34]; with permission)
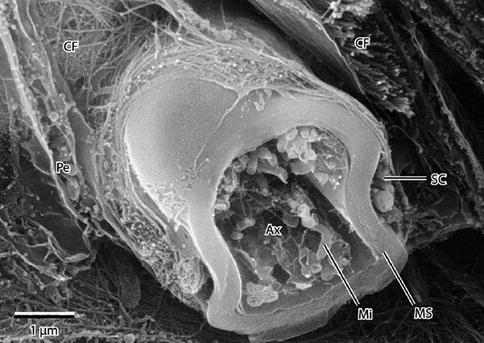
Fig. 9.9
High-resolution scanning electron micrograph of a myelinated peripheral nerve fiber fractured in the transverse plane. The axon, fractured open, reveals mitochondria (Mi) and cytoskeletal elements in the axoplasm (Ax). A peripheral rim of Schwann cell cytoplasm (SC) is outside the myelin sheath (MS). Collagen fibrils (CFs) of the surrounding endoneurium are shown well. A flattened perineurial cell (Pe) is also fractured open. 15,000× (From Steed [34]; with permission)
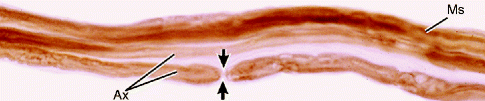
Fig. 9.10
Light micrograph of teased myelinated nerve fibers demonstrating a node of Ranvier. The axon (Ax) is the central pale region in each fiber. Myelin sheaths (MSs), visible when fixed and stained with osmium, appear as dark linear densities. A node of Ranvier (arrows) is indicated. 500×. Osmium (From Steed [34]; with permission)
The cell bodies of the peripheral trigeminal nerve, including the lingual and inferior alveolar nerve, are contained within the trigeminal ganglion (also called the semilunar ganglion). The trigeminal ganglion is analogous to the dorsal root ganglia of the spinal cord, which contain the cell bodies of incoming sensory fibers from the rest of the body. These nerve cells may have axons that extend over a distance corresponding to thousands of cell body diameters. This imposes special requirements on the communication systems between the proximal and distal regions of the cell. To meet these requirements the neuron has unique systems of anterograde as well as retrograde intracellular transport. These transport mechanisms are involved in the response to an injury.
9.3 Basic Injury Types
Clinically useful injury grading systems have been developed that allow reflection of patient symptomatology after nerve injury and with the histological changes occurring to the nerve. Histological parameters are the far most used predictors of peripheral nerve damage and regeneration. In 1941, Cohen introduced a classification scheme to describe the injury to peripheral nerves: neuropraxia, axonotmesis, and neurotmesis [30]. In the early 1940s, Seddon [33] examined 650 patients with peripheral injuries and popularized Cohen’s time and degree of recovery-based classification system. In 1951, Sunderland [36] expanded upon this classification system by defining five distinct degrees of nerve injury based on histological changes within the nerve [30] (Figs. 9.11 and 9.12). Within this classification scheme, first-degree injuries are representative of a neuropraxia, while axonotmesis is divided into second-, third-, and fourth-degree injuries. Fifth-degree injuries represent a neurotmesis. In 1989, Mackinnon coined the term “Sixth-degree injury” which was defined as a mixed injury involving different combinations of the above [29].
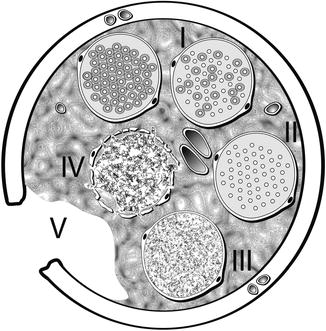
Fig. 9.11
Diagrammatic representation of Sunderland classification of distal segment nerve injury in transverse plane. A normal, uninjured fascicle with myelinated axons is represented at the top left. Proceeding clockwise, fascicle number I represents a first-degree injury with demyelination of some axons. Fascicle number II depicts a second-degree injury with more extensive demyelination without injury to the endoneurium. Fascicle number III is involved in a third-degree injury, now with disruption of the endoneurium around each axon. Fascicle number IV shows a fourth-degree injury with damage extending through the perineurium. Transection injury to all of the nerve components and supporting elements is reflected by the number V representing a fifth degree injury (Courtesy of Don Johnson, Atlanta VA Medical Center)
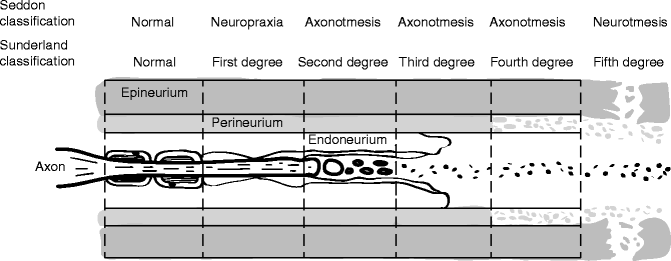
Fig. 9.12
Schematic representation of Sunderland classification of nerve injury in longitudinal plane. Sunderland classification scheme is shown in longitudinal section demonstrating the “inside–out” histological progression of injury with a fifth-degree injury representing a neurotmesis defined as complete nerve transection
9.3.1 First-Degree Injury, Neuropraxia
A first-degree peripheral nerve injury (neuropraxia) clinically shows recovery within the first 3 months. It represents a conduction block with good chances for complete recovery from within days to 3 months. Pathologic changes are mild (demyelination) or absent in first-degree injuries [4]. An example would be a mild nerve stretch injury to the lingual nerve from retraction of a lingual flap during a surgical extraction of a third molar tooth.
9.3.2 Second-Degree Injury, Axonotmesis
In second-degree injury the endoneurium and perineurium remain intact. In second-degree injuries there is little histological change at the injury site or proximal to it; however, distal to the site of injury, a calcium-mediated process known as Wallerian degeneration occurs [2]. The general arrangement of the axonal sheaths and the remaining structures comprising the nerve are preserved [30]. The prospect of recovery is possible in such injuries because of the remaining uninjured mesenchymal latticework that provides a path for subsequent sprouting axons to reinnervate their target organ. Nerve recovery should be complete but progresses slowly at approximately 1 cm/day or 1 in./month.
9.3.3 Third-Degree Injury, Axonotmesis
The third-degree injury pattern involves endoneurium scarring and disorganization with the fascicles. Damage extends to the perineurium. The endoneurial tube (or sheath) is disrupted, resulting in misalignment of any regenerating axonal fibers. Regeneration from the proximal nerve takes place through scar tissue in the endoneurium, thereby limiting the regenerating axons ability to make contact with distal sites [30]. The rate of recovery progresses as expected for an axonotmesis (1 in./month), but the degree of return will not be complete.
9.3.4 Fourth-Degree Injury, Axonotmesis
A fourth-degree injury is in which the nerve is physically in continuity, but only the epineurium remains intact. Damage extends through the perineurium and regeneration attempts are blocked by scar tissue. After Wallerian degeneration takes place, the axonal continuity may eventually be interrupted, resulting in degeneration of the distal axonal segment and complete denervation. If recovery is to occur, then surgical intervention is necessary. These injuries are typically the result of severe stretch, traction, crush, or cautery injuries, or nerve injection injuries [30].
9.3.5 Fifth-Degree Injury, Neurotmesis
A fifth-degree injury results from the complete transection of a nerve trunk and loss of all supporting elements. Although these injuries can result from severe stretch that leads to avulsion, they are more often associated with sharp or penetrating trauma. Recovery is not possible without a surgical repair, although inferior alveolar nerve transection during third molar removal with replacement of the proximal and distal nerve stumps into the inferior alveolar canal may have a reasonable prognosis for spontaneous recovery without subsequent nerve repair. However, in most fifth-degree injuries, functional loss is complete and spontaneous recovery is unlikely.
9.3.6 Sixth-Degree Injury, Neuroma in Continuity
Mackinnon used the term “Sixth-degree injury, neuroma in continuity” in 1989, to describe a mixed nerve injury [29]. She illustrated how the pattern of recovery of the whole nerve is mixed to a varying degree by fascicle (I, II, III, IV, and V) [30]. Complete recovery takes place in fascicles that have sustained a first- or second-degree injury. Partial recovery is seen in fascicles that have a third-degree injury, while no recovery is seen in fourth- or fifth-degree injury patterns.
9.4 Overview of Response to Injury
The healing of peripheral nerve injuries is quite unique within the body as it is a process of cellular repair rather than tissue repair. In other words, the nerve cells themselves do not undergo mitoses. The number of nerve cells (neurons) does not increase, but the amputated nerve cell regains its original axoplasmic volume by sending out new processes to the end organ target. Peripheral nerves do have this capacity to regrow, but functional recovery in humans is often incomplete [15]. This is because the neuron and surrounding cells cannot maintain an effective growth promoting response for long periods of time. Peripheral nerves are some of the longest and most spatially complex cells in the body. A normal, uninjured nerve is represented in Fig. 9.13. Their length makes them unable to function without the structural and metabolic support provided by approximately ten times as many glial support cells [20]. A nerve injury that takes place centimeters from a neuronal cell body induces a response that involves the entire cell and its associated glial support cells. Although the number of neurons does not increase in number, the repair of each cell takes place in an environment of intense cellular proliferation. The cells that do show evidence of proliferation include Schwann cells, endothelial cells, and fibroblasts.
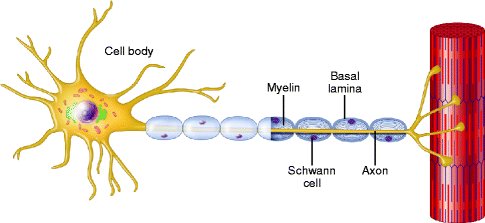
Fig. 9.13
A normal myelinated axon. A schematic representation of a normal myelinated axon associated with a longitudinal chain of Schwann cells and enclosed within a continuous basal lamina (From Steed [34]; with permission)
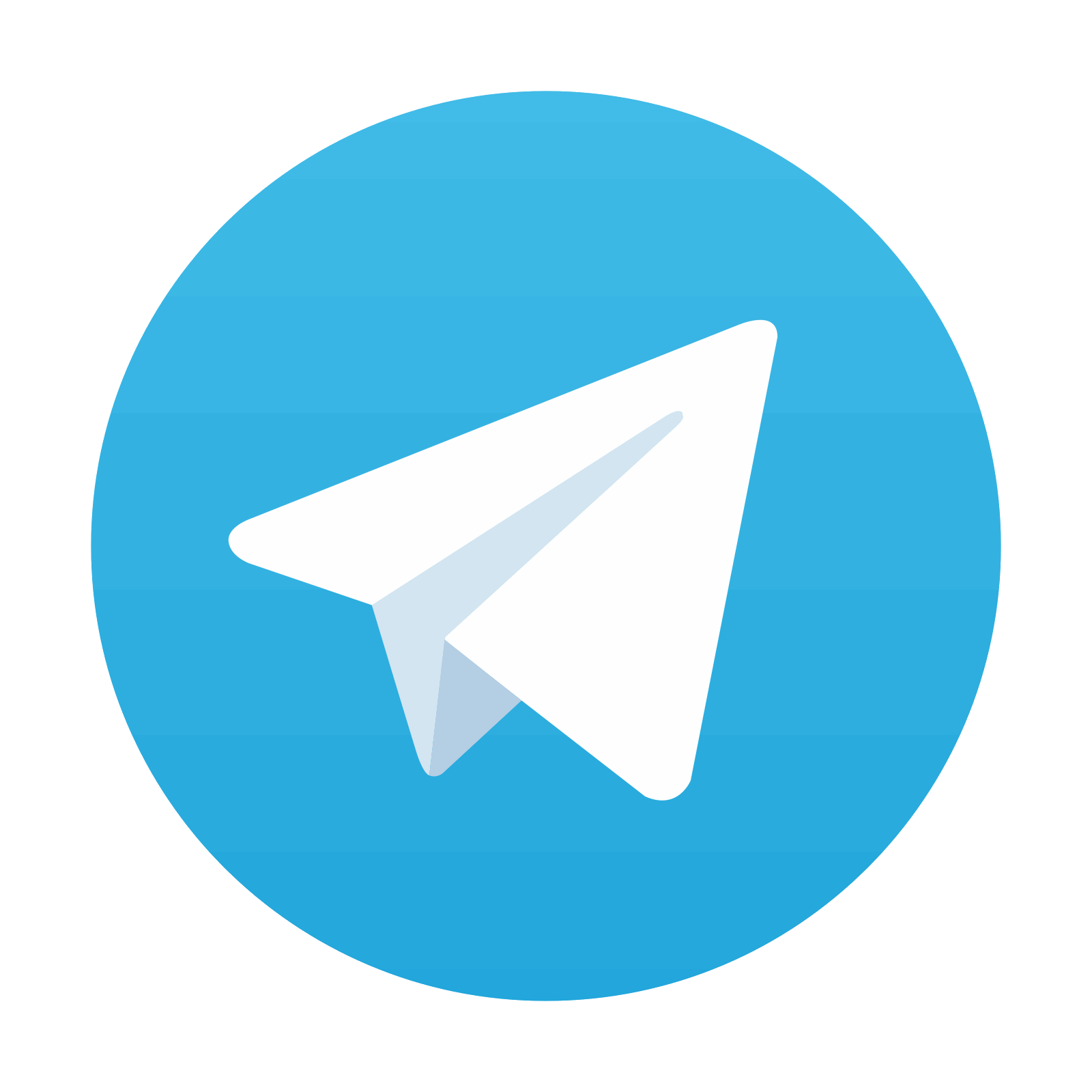
Stay updated, free dental videos. Join our Telegram channel

VIDEdental - Online dental courses
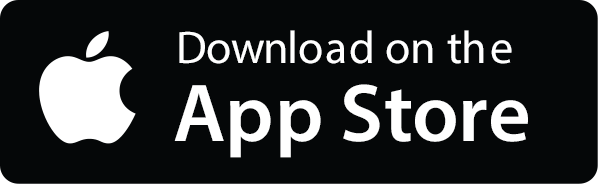
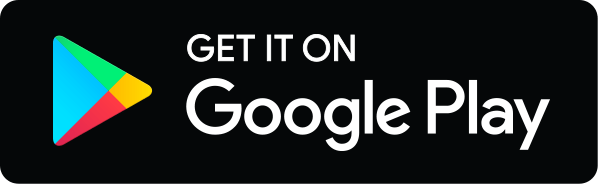