Current periodontal treatments aim to control bacterial infection and decrease inflammation. To optimize contemporary conventional treatments that present limitations owing to an inability to reach the lesion site, new methods are based on nanomedicine. Nanomedecine allows delivery of host-modulatory drugs or antibacterial molecules at the lesion site in an optimal concentration with decreased toxicity and risk of systemic side effects. Chitosan and polylactic-co-glycolic acid-loaded nanoparticles, carbon quantum dots, and mesoporous silicates open new perspectives in periodontitis management. The potential therapeutic impact of the main nanocarriers is discussed.
Key points
- •
Nanomedicine is a promising tool to improve periodontal treatment outcomes with a decreased risk of side effects.
- •
Nanocarriers are able to deliver antibacterial, anti-inflammatory and proregenerative drugs or molecules.
- •
Nanoparticles or nanoreservoirs can be included in scaffolds, such as gels, membranes, and bone scaffolds.
Introduction
The regeneration of periodontal tissues is the ultimate goal of periodontal therapy. Despite impressive progress over the last 30 years, especially in terms of nonsurgical and surgical therapies (antibiotics, photodynamic therapy, probiotics, minimally invasive surgical approaches, biomaterials development, etc), tissue regeneration remains difficult to achieve in some specific clinical scenario, such as complex lesions with a lack of bone walls or those that are difficult to reach, such as a furcation area. Pharmacologic approaches have been proposed to optimize treatment outcomes, targeting either infection, inflammation, or tissue growth. However, systemic administration is complex because it induces an unnecessary systemic loading of the drug as well as a decreased bioavailability at the periodontal pocket site. , To overcome such limitations and improve treatment outcomes, innovative nanotechnologies have been developed and tested over the last years with promising results. Nanomedicine has been defined as “the monitoring, repair, construction, and control of human biological systems at the molecular level, using engineered nanodevices and nano-structures.” It uses the properties of materials at a nanometric scale to improve diagnosis and imaging, as well as to promote tissue regeneration through the delivery of bioactive compounds or drugs.
The application of nanomedicine and nanotechnologies has been suggested to be a part of the therapeutic arsenal for several diseases such as cancers and inflammatory diseases such as rheumatoid arthritis, aiming to deliver most efficiently the drug or active compounds to a specific site. Therefore, the use of such nanotechnologies for the treatment of periodontal diseases, mainly periodontitis, has been proposed with the goal to deliver a sufficient concentration of active molecules at the targeted site and to avoid its distribution in nonspecific tissues, consequently decreasing the risk of side effects. The onset and development of the periodontal lesion involve several cell types, including epithelial cells from the junctional epithelium, fibroblasts from the connective tissue, bone cells, and all immune cells, especially polymorphonuclear neutrophils and macrophages. The targeting of such cells to arrest the proinflammatory host response and/or to promote the proresolution of the inflammation is then required and several nanocarriers have been developed. Nanocarriers are polymers of biological or synthetic origin that are used to deliver drugs such as antibiotics, antibodies, or other macromolecules adsorbed on their surfaces or within their core and are for most of them biodegradable. Indeed, chitosan, polylactic-co-glycolic acid (PLGA), carbon quantum dots, and mesoporous silica/bioactive glass have been developed and tested in several applications including periodontitis , ( Fig. 1 ). The aim of this review is to describe the advantages of such nanoparticles and the feasibility of their use in the specific context of periodontitis treatment.

Chitosan-based nanomaterials
Chitosan is a natural polysaccharide that can be easily produced via the alkaline N -deacetylation process of a natural biopolymer commonly found in the shells of marine crustaceans and in fungi cells walls, the chitin. It is widely used to synthesize biomedical scaffolds or implants owing to its biocompatibility, antibacterial properties, positive host response, and sufficient mechanical strength. It is already used as a wound dressing due to its interesting hemostatic and antimicrobial properties contributing to the formation of the blood clot and to a decrease in the risk of infection at the treated site. , The inherent antimicrobial properties of chitosan, mainly associated with its cationic nature, have been demonstrated against different microorganisms in several cellular and animal models. In the context of periodontitis, the antibacterial properties of chitosan nanoparticles have been tested against major periodontal pathogens such as Porphyromonas gingivalis and Aggregatibacter actinomycetemcomitans . Indeed, it was shown that exposure of these bacteria to chitosan nanoparticles inhibits bacterial growth in vitro. Chitosan-based nanoparticles could also be loaded with antimicrobial peptide to interact with pathogens in a planktonic state, as well as when organized in biofilms as observed for P.gingivalis , Fusobacterium nucleatum , and Streptococcus gordonii . It can also be loaded with drugs such as antibiotics, that is, minocycline and antiseptics such as chlorhexidine to enhance their properties. , Chitosan nanoparticles or nanoreservoirs have also been used to deliver on site immune-modulatory molecules and proregenerative factors. Several studies have been conducted in this regard with significant effects , ( Table 1 ). For instance, the delivery of statins using chitosan carriers was evaluated and showed promising results in terms of periodontal regeneration. In a model of experimental periodontitis in dogs or in a mouse calvarial defect. Chitosan has also been used to synthesize nanoreservoirs that could be constructed on the fibers of scaffolds such as membrane. Such technology demonstrated efficiency toward the delivery of growth factors such as BMP-2 enhancing bone regeneration with improved biocalcification of the scaffold and especially in the context of maxillary bone regeneration ( Fig. 2 ).
Study | Type of Study | Active Drug | Size of Particles | Effects |
---|---|---|---|---|
Hu et al, 2021 | In vitro: biofilm ( F nucleatum, P gingivalis and S gordonii ) | Nal-P-113 (antimicrobial peptide) loaded poly (ethylene glycol) combined CS nanoparticles (Nal-P-113-PEG-CSNPs) | 216.2 ± 1.6 nm |
|
Soe et al, 2020 | In vitro: HPDLCs | AS loaded SBEβCD/CS NPs | 25–350 nm |
|
Xu et al, 2020 | In vitro: effect of P gingivalis on HGFs | Dox:CS/CMCS-NPs | 203.1 ± 10.51 nm |
|
Martin et al, 2019 | In vitro: P gingivalis LPS-stimulated culture of HGF | MH-NPs | 50 ± 17 nm |
|
Aminu et al, 2019 | In vivo: experimental periodontitis in rat | Triclosan and flurbiprofen loaded in a CS-based hydrogel | 100–400 nm |
|
Xue et al, 2019 | In vitro: Effect on proliferation and mineralization of periodontal membrane cells In vivo: Proliferation and mineralization of periodontal membrane cells were investigated and tested in animals (New Zealand White rabbits) |
CS, PLGA, and silver nanoparticles (PLGA nanoparticles, CS nanoparticles, silver nanoparticles) | 112–180 nm |
|
Hu et al, 2018 | In vitro: Cytocompatibility and biocompatibility in human periodontal ligament fibroblasts. Inhibition test on mixed bacteria ( P gingivalis and Prevotella intermedia ) In vivo: Test on biofilm formation and alveolar bone absorption (rats) |
Quaternary ammonium CS, that is, TMC-Lip-DOX NPs | 129.7 nm |
|
He et al, 2018 | In vitro: cell culture of rBMSCs | Gln and CS composite GBR membrane containing hydroxyapatite nanoparticles and antimicrobial peptide–loaded PLGA microspheres |
|
|
Guarino et al, 2017 | In vitro: Inhibitory activity evaluated against E coli , S aureus , and A actinomycetemcomitans |
Amoxicilline trihydrate loaded in CS nano-reservoirs | 0.1–0.4 μm |
|
Lin et al, 2017 | In vivo: Effect of nanosphere in induced periodontitis in rat |
PLGA and CS encapsulated metronidazole and N-phenacylthiazolium bromide | 499 ± 21.24 nm |
|
Li et al, 2017 | In vivo: In rat calvarial defect and periodontitis induced bony defect in beagle dog |
pDNA-BMP2-loaded in CS nanoparticles (pDNA-BMP2)-GP |
|
|
Li et al, 2016 | In vitro: Release and cytocompatibility to HPDLCs |
CSn loaded with pDNA-BMP2 into a CS-based hydrogel with α,β-glycerophosphate | 270.1 nm |
|
Lee et al, 2016 | In vitro: Cytotoxic effect and alkaline phosphatase activity of the nanoparticles in osteoblast cell culture and antibacterial activity against periodontal pathogens ( A actinomycetemcomitans and Prevotella nigrescens ) In vivo: Regeneration potential in 3 wall defect in beagle dog |
PLGA-lovastatin-CS-tetracycline nanoparticles | 111.5 nm. |
|
Barreras et al, 2016 | In vitro: Antibacterial effect against Enterococcus faecalis cultures and infected collagen membranes |
CS nanoparticles containing chlorhexidine | 70.67 ± 14.86 nm |
|
Arancibia et al, 2013 | In vitro: Antibacterial effect against periodontal pathogens ( P gingivalis, A actinomycetemcomitans ) and inflammatory response in gingival fibroblasts |
CS | Not specified |
|

Polylactic-co-glycolic acid
PLGA is a synthetic biodegradable copolymer widely used in medical applications owing to its minimal cytotoxicity. Its degradation by hydrolysis results in biocompatible byproducts, lactic acid and glycolic acid, that are physiologically metabolized. PLGA has been used as a nanocarrier to deliver active drugs to treat several diseases or to promote tissue regeneration. It has been used extensively to design scaffolds, including barrier membranes, bone scaffolds, sponges, and gels ( Fig. 3 ). PLGA displays interesting physical properties because its viscosity is modulable; however, it is also associated with an initial burst release of the active molecule, and therefore is often use in combination with chitosan in a core shell technique. The modulation of the thickness of the shell could be interesting to increase the time needed to deliver the active molecule. In the context of periodontal treatment, PLGA nanoparticles (or PLGA–chitosan nanoparticles) have already been tested in several model (in vitro and in vivo) and in clinical settings ( Table 2 ). Indeed, antibiotics, compounds of natural origin with anti-inflammatory properties such as curcumin, metals such as silver, and immunomodulatory drugs such as statins have been loaded. Most of the studies exhibited positive outcomes resulting in a decrease in a decrease of periodontal pathogens growth and inflammatory cytokine secretion. Interestingly, PLGA nanoparticles have also been tested as methylene blue carrier in the context of treatment of periodontal pockets with photodynamic therapy. , The use of nanocarriers, such as PLGA, allows to deliver locally a high concentration of photosensitizer at the site, decreasing the risk of multidrug resistance.

Study | Type of Study | Active Drug | Size of Particles | Effects |
---|---|---|---|---|
Beg et al, 2020 | In vivo: experimental periodontitis in rats. Effect of in situ gel containing nanoparticle of moxifloxacin hydrochloride |
Moxifloxacin hydrochloride | 204.63–292.81 nm |
|
Ghavimi et al, 2020 | In vitro: Staphylococcus aureus, Escherichia coli , and Enterococcus faecalis cultures; Dental pulp stem cells In vivo: alveolar bone defect in mongrel dogs Antibacterial, cytocompatibility and proregenerative properties were evaluated |
Membrane functionalized with curcumin and aspirin-loaded PLGA nanoparticles | 50–85 nm |
|
Pérez-Pacheco et al, 2019 | Clinical (6 mo): periodontitis patients received SRP + PLGA/PLA nanoparticles loaded with 50 μg of curcumin or SRP + empty nanoparticles | Curcumin | Not specified |
|
Lecio et al, 2019 | Clinical (6 mo): Effect of PLGA nanospheres containing 20% doxycycline on patients with diabetes type II with chronic periodontitis vs PLGA + placebo |
Doxycycline | 1 μm |
|
Mahmoud et al, 2019 | In vitro: telomerase immortalized gingival keratinocytes In vivo: Measure of alveolar bone destruction in mouse experimental periodontitis Effect of peptide (BAR) derived from S gordonii – modified PLGA nanoparticles |
BAR (peptide) | 333 nm and 312 nm (hydrated) |
|
Xue et al, 2019 | In vitro: primary human periodontal ligament cells; E coli culture In vivo: Measure of the bone regeneration in the mandible of New Zealand white rabbits Effect of PLGA nanoparticles, CS nanoparticles, silver nanoparticles and a combination of the 3 were evaluated. |
112.4 ± 8.33 nm (PLGA nanoparticles) 180.3 ± 11.2 nm (CS nanoparticles) |
|
|
Pereira et al, 2018 | In vivo: Evaluation of the effect of metformin hydrochloride-loaded PLGA in a ligature-induced periodontitis model in diabetic rats | Metformin hydrochloride | 457.1 ± 48.9 nm |
|
Rizzi et al, 2016 | In vitro: Human keratinovytes Evaluation of proliferative effects of epiregulin- PLGA nanoparticles on human keratinocytes |
Epiregulin | 190–370 nm |
|
Lee et al, 2016 | In vitro: human bone marrow-derived osteoblasts; A actinomycetemcomitans and Prevotella nigrescens culture In vivo: maxillary intrabony defect in dog Evaluation of cytotoxic effect and alkaline phosphatase activity, as well as antibacterial activity and bone regenerative potential of PLGA–lovastatin–chitosan–tetracycline nanoparticles |
Lovastatin-chitosan-tetracycline | 105–111 nm |
|
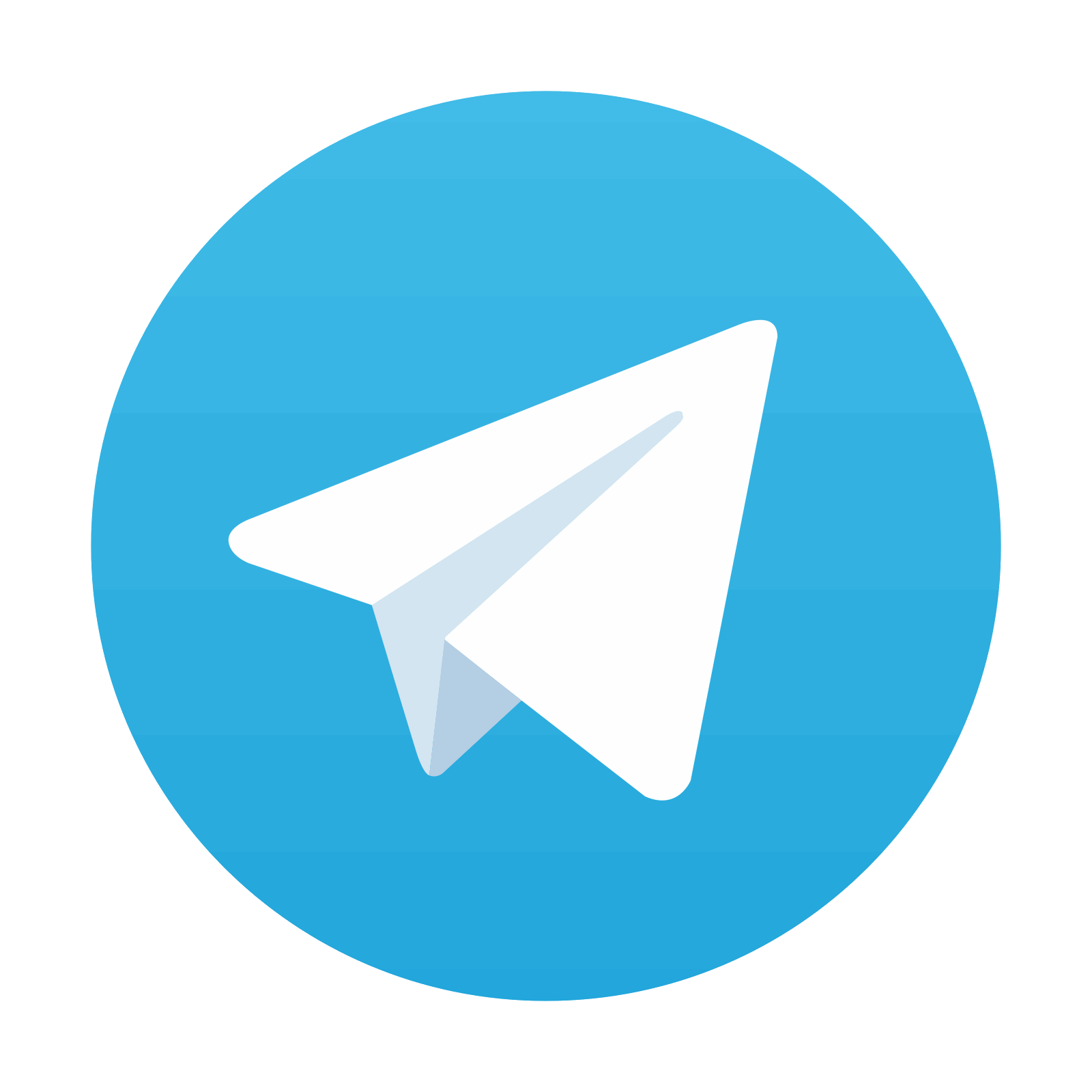
Stay updated, free dental videos. Join our Telegram channel

VIDEdental - Online dental courses
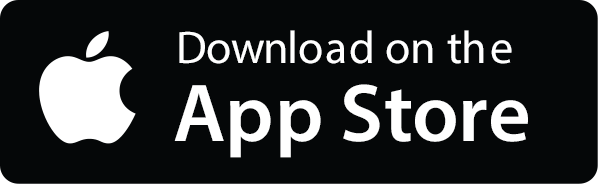
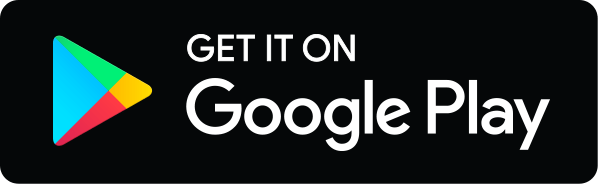
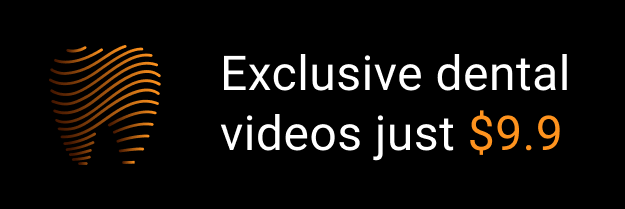