The ultimate goal in periodontal therapy is the complete re-establishment of the lost tissues. Dental researchers and clinicians are continuously working to develop current therapeutic techniques and technologies that can regenerate damaged periodontal tissues. Predicting the outcome of the treatment is a challenging endeavor, because a variety of local and systemic variables can affect the success of the applied regenerative therapy. To real-time monitor the biological changes during periodontitis or after periodontal treatment, various biomarkers have been studied in periodontology. This article discusses the available evidence on the use of biomarkers in the detection of periodontal regeneration.
Key points
- •
Simple and reliable methods that can observe periodontal tissue regeneration will bring significant advantages to dental clinicians.
- •
In medicine, various serum proteins have been studied as potential biomarkers of osteoblast or osteoclast activities.
- •
Available data are not enough to propose the use of any specific biomarker in determination of periodontal regeneration.
- •
Bone alkaline phosphatase and osteocalcin are potential biomarker candidates to predict and follow the success of periodontal regeneration after treatment.
Introduction
Periodontal disease is of infectious origin and inflammatory character, where chronic and uncontrolled immune responses initiate the process that leads to the destruction of tooth-supporting tissues and, if untreated, eventually to tooth loss. During the disease process, the balance between removal and regeneration of periodontal tissue components is disrupted: new tissue formation activated by keratinocytes, fibroblasts, and osteoblasts is suppressed, whereas tissue degradation activated by neutrophils, macrophages, and osteoclasts is stimulated. The integrity of the junctional epithelium, periodontal ligament, and alveolar bone is broken in some degrees, depending on the stage of periodontitis.
Periodontal tissue damage around the affected teeth can be measured by using clinical (probing pocket depth [PPD], clinical attachment level [CAL]) or radiographic (alveolar bone loss) indices. In most cases, it is possible to prevent the disease progression using nonsurgical and surgical periodontal therapies; however, replacing the damaged periodontal tissues with the physically and functionally similar structures (ie, regeneration) remains challenging. Periodontal regeneration is the ultimate goal of periodontal therapy, and the anatomic form, localization, and extent of the periodontal defect are local determinants of periodontal treatment to be applied. In general, residual pockets with 2- to 3-wall intrabony defects are accepted as the most favorable cases for periodontal regeneration, because these defects can successfully regenerate after minimally invasive surgical and minimally invasive nonsurgical treatments, without the need of complicated surgical procedures. Nevertheless, various bone regenerative substitutes and biological agents with osteoinductive or osteoconductive properties are widely used in periodontal practice to get new bone around periodontitis-affected teeth.
Randomized controlled studies have documented the potential of various regenerative therapies to achieve periodontal regeneration in periodontal defects; however, the predictability of treatment success or failure is still a challenge. The outcome of the treatment can differ between 2 identical defects because the regeneration is influenced by multiple factors related to patient, surgical approach, and defect site. As the periodontal regenerative treatments are expensive and time-consuming, clinical use of biological markers that indicate the success or failure of the treatment at its early phases would be beneficial.
Technological advancements and breakthroughs during the last 3 decades have improved our quality of life significantly. Moreover, these advancements have influenced oral sciences, because nowadays it is possible to quantify previously undetectable host or bacterial proteins from patient samples as well as to relate their levels to disease diagnosis, prognosis, or treatment response.
This narrative review aims to discuss periodontal regeneration, that is, regeneration of alveolar bone and periodontal ligament, from biomarker research perspective and to present the available evidence on the use of regeneration-related biomarkers in periodontology. Biomarkers of periodontal wound healing and pocket depth reduction are not included in this review, because they do not necessarily represent regeneration.
Biological markers: biomarkers
A biomarker is “a characteristic that is objectively measured and evaluated as an indicator of normal biological processes, pathogenic processes, or pharmacologic responses to a therapeutic intervention” as defined by the working group of the National Institutes of Health Director’s Initiative on Biomarkers and Surrogate Endpoints. In general, biomarkers are applied to clinical study designs to get fast and reliable information on an objectively defined clinical outcome (clinical endpoint). In such study designs, a well-validated biomarker (ie, with high sensitivity, specificity, and reproducibility), which has either a causal or mechanistic association with the disease pathogenesis or therapeutic intervention, functions as a surrogate end point to predict the clinical end point. Overall, biomarkers are used to (1) diagnose a disease, (2) stage or classify the extension of the disease, (3) follow the disease prognosis, and (4) predict or monitor the treatment outcome.
Biomarker research in periodontology
In periodontology, searches for quantifiable and easy-to-detect biomarkers from noninvasively collected biological samples (saliva, oral rinse, gingival crevicular fluid) are not new. Indeed, for decades, researchers have looked for biomarkers to detect disease, disease initiation, disease progression, successful treatment outcomes, and periodontal regeneration , ( Table 1 ). Most biomarker studies in periodontology have been performed either to discriminate patients with periodontitis from periodontally healthy individuals (at the population level) or to detect disease activity in periodontal pockets (at the site level). It is indeed understandable that the “susceptibility to develop periodontitis,” “initiation of periodontitis,” or “periodontal regeneration” has been rarely applied as periodontal end points, because these outcomes require longitudinal studies. To validate a biomarker’s sensitivity and specificity to detect “susceptibility to develop periodontitis” or “initiation of periodontitis,” a large number of individuals need to be followed over years and preferably must not be treated when study participants develop gingivitis. This type of study design will be neither ethically nor financially possible.
Marker types | Potential applications |
---|---|
Genetic markers (eg, interleukin-1, mannose-binding lectin genotypes) |
|
Infectious and inflammatory markers (eg, Porphyromonas gingivalis and its gingipain , interleukin-1β, interleukin-17, tumor necrosis factor-α) |
|
Wound healing markers (eg, keratinocyte growth factor, basic fibroblast growth factor) |
|
Regeneration markers (eg, alkaline phosphatase, osteocalcin) |
|
Until now various host and bacterial proteins, including matrix metalloproteinase (MMP)-8, interleukin (IL)-1β, and Porphyromonas gingivalis or its gingipains, have been demonstrated to be successful in diagnosing periodontitis and to predict the healing response after periodontal treatment. , ,
Biomarkers of periodontal regeneration: obstacles
Research targeted to define a periodontal regeneration biomarker has some obstacles. First, as a general rule in biomarker studies, the primary clinical end point needs to be precisely defined and measured objectively. To give an example, when “periodontitis” is taken as a clinical end point, it is defined precisely as “periodontal tissue destruction due to inflammation as a primary feature, with a threshold of interproximal, clinical attachment loss of ≥4 mm at ≥2 non-adjacent teeth.” Here, the levels of inflammation and interproximal clinical attachment loss can be measured objectively. On the contrary, periodontal regeneration, that is, formation of new bone and periodontal attachment, does not have a precise and globally accepted clinical definition. Moreover, gold-standard detection methods of periodontal regeneration are either (1) determination of new bone formation by surgical reopening of the defect area, which is highly invasive and costly, or (2) radiographic determination of bone gain, which can only detect already matured bone (ie, cannot reveal early bone formation).
Another limitation in determining periodontal regeneration biomarkers is related to the biology of new tissue formation. Periodontitis is a degenerative disease regulated by inflammation, where levels of proinflammatory cytokines (eg, IL-1β, IL-6, IL-8, IL-17, tumor necrosis factor [TNF]-α), bacterial and host proteases (eg, P gingivalis gingipains, MMPs), and tissue degradation end products (eg, cross-linked carboxy terminal telopeptide of type I collagen) are highly elevated in active phase of the disease. These biomarkers can be easily detected in gingival crevicular fluid or in saliva with current laboratory methods. Periodontal regeneration, however, is a slow and long-term process, which does not lead to a rapid synthesis of signaling molecules ( Fig. 1 ). Moreover, extracellular levels of new tissue formation-inducing signaling molecules during regeneration do not increase as significantly as those of proinflammatory cytokines or proteolytic enzymes during active periodontitis. Therefore, measuring alveolar bone regeneration-related marker levels in oral biological fluids, especially in saliva and oral rinse, with current methodological techniques is troublesome.

Biomarkers of periodontal regeneration: potential targets
The periosteum, periodontal ligament, and alveolar bone walls act as reservoirs for the cells that take part in periodontal regeneration. Growth and differentiation factors are the major regulators of cellular behaviors. Among them, bone morphogenic proteins, growth differentiation factors, platelet-derived growth factor, fibroblast growth factor (FGF), insulin-like growth factor (IGF), transforming growth factor (TGF)-β, and regeneration signaling pathways (Wnt, FGF, TGF-β signaling) have been extensively studied.
Bone is a dynamic tissue and undergoes continuous remodeling (resorption, reversal, formation) throughout the life. Both remodeling and regeneration of alveolar bone depend on systemic and local factors and may show significant variations between individuals, teeth, and periodontal sites. Understanding the mechanical and biological properties of bone tissue allows researchers to develop new surgical techniques and bioactive molecules that may support regeneration; yet, predicting the tissue response and the rate of regeneration is still a challenge.
During a physiologic remodeling process, the resorption of old bone by osteoclasts and formation of new bone by osteoblasts are in a delicate balance. Although osteoclasts are not included in bone formation, coupling of osteoclasts to osteoblast function is required for the maturation of new bone. The main events that regulate new bone formation include the mesenchymal stem cell recruitment, nonmineralized matrix deposition, osteoblast differentiation, and mineralization. Among signaling pathways, the Wnt pathway was related to osteogenic gene expression in alveolar bone in mice. mRNA expressions of alkaline phosphatase (ALP), osteoprotegerin, osteopontin, osteocalcin, and bone sialoprotein have been detected in newly formed alveolar bone. Although growth factors are responsible for the recruitment, migration, differentiation, and proliferation of regenerative cells and bone formation, studies investigating the associations between serum growth factor levels and bone turnover have yielded conflicting results. For example, IGF levels in serum were found to be associated or not associated with new bone formation.
In medicine, bone alkaline protease (BALP), total osteocalcin, and procollagen type I N-terminal propeptide (P1NP) levels in serum are used as sensitive and reliable markers of new bone formation.
ALP is an enzyme that is produced mainly by the liver, bones, intestines, and kidneys. Serum levels of ALP vary by gender, age, pregnancy, and blood type, and its unusual levels refer to diseases of the liver (primary biliary cirrhosis) or bone (rickets, osteomalacia). BALP is an osteoblast enzyme and functions in new bone mineralization. In a systemically healthy adult, bones produce about 50% of the ALP present in serum. Various laboratory methods, including enzyme immunoassays and immunoradiometric assays, have been applied to specifically detect BALP levels and relate them to new bone formation levels. Immunohistochemical studies on rats and humans have demonstrated a strong ALP positivity in newly formed alveolar bone. , Elevated numbers of ALP-positive cells were also detected in relation to the inhibition of alveolar bone loss in periodontitis-induced rats. In orthodontic treatment studies , and in immunohistochemical studies using human and animal models, BALP activity levels correlate with new bone formation. BALP can be measured by electrophoresis, immunoassays, and high-performance liquid chromatography-based methods. Although immunoassays produce the most reproducible and precise results, the cross-reactivity of BALP antibodies with the liver isoform, ALP, is the major limitation of immunoassay use.
Osteocalcin is a small matrix protein, which is synthesized by mature osteoblasts, odontoblasts, and chondrocytes. The main functions of osteocalcin are connected to bone mineralization and calcium ion homeostasis. In patients with osteoporosis, osteocalcin has been effectively used for the determination of osteoblast function and drug-induced bone formation. Elevated numbers of osteocalcin-positive cells after taxifolin treatment were immunohistochemically demonstrated in an experimental periodontitis model in rats. Various methods, including enzyme-linked immunosorbent assay (ELISA), bead-based immunoassay, and chemiluminesence immunoassays, are used in detecting osteocalcin; however, the results may vary between different methods due to the heterogeneity of osteocalcin in the circulation. Furthermore, the reproducibility of osteocalcin level measurements is problematic, because osteocalcin is rapidly degraded into fragments in the circulation and has a short half-life (∼5 min). Moreover, increased osteocalcin levels in can be detected in the serum of individuals with renal failure, because osteocalcin is degraded by the kidneys.
P1NP and procollagen type 1 C-terminal propeptide (P1CP) are by-products of the collagen synthesis and thus biomarkers of collagen deposition. Type I collagen forms 90% of the organic bone matrix. During the collagen synthesis, P1NP and P1CP are released into the intracellular space in trimeric structures and can be detected in serum. The unstable trimeric form degrades into stable monomers and fragments that may affect its detectability. Radioimmunoassay and ELISA are methods developed to detect serum P1NP levels.
Biomarkers of periodontal regeneration: evidence
Evidence on the use of biomarkers to predict periodontal regeneration is highly limited. In the literature, there are numerous studies that relate biomarker levels to posttreatment clinical outcomes. However, the selected clinical end points among the studies vary significantly. The most commonly used clinical end points are (1) inhibited disease progression (elimination of clinical signs of inflammation), (2) reduced pocket depths (formation of long junctional epithelium), , or (3) periodontal regeneration (periodontal attachment and alveolar bone gain). Notably, neither decreased bleeding on probing nor reduced PPDs indicate periodontal regeneration, thus these studies were not included in this section. Also, studies using salivary biomarkers were not included, because they cannot be related to site-specific changes. Hereby, we present the available evidence collected from clinical studies where both periodontal regeneration (CAL gain or radiographic evidence of new bone formation) was taken as a clinical end point and the biomarker and periodontal regeneration levels were quantified from the same tooth site.
No reports on biomarkers in relation to new bone formation were found. In 4 studies, biomarker levels were quantified in gingival crevicular fluid and related to CAL gain , ( Table 2 ). Perinetti and coworkers showed a correlation between the ALP activity in gingival crevicular fluid and CAL gain, whereas Pellegrini and coworkers suggested that elevated endothelial growth factor levels in gingival crevicular fluid at baseline indicate posttreatment CAL gain.
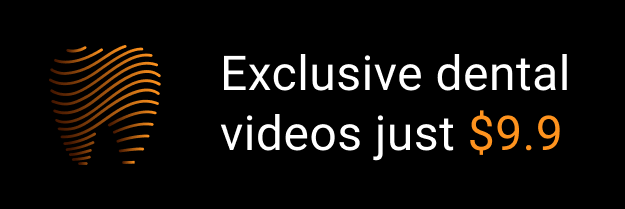