Abstract
Objectives
Fluoride (F) releasing dental restoratives are promising to promote remineralization and combat caries. The objectives of this study were to develop nanocomposite containing calcium fluoride nanoparticles (nCaF 2 ), and to investigate the long-term mechanical durability including wear, thermal-cycling and long-term water-aging behavior.
Methods
Two types of fillers were used: nCaF 2 with a diameter of 53 nm, and glass particles of 1.4 μm. Four composites were fabricated with fillers of: (1) 0% nCaF 2 + 65% glass; (2) 10% nCaF 2 + 55% glass; (3) 20% nCaF 2 + 45% glass; (4) 30% nCaF 2 + 35% glass. Three commercial materials were also tested. Specimens were subjected to thermal-cycling between 5 °C and 60 °C for 10 5 cycles, three-body wear for 4 × 10 5 cycles, and water-aging for 2 years.
Results
After thermal-cycling, the nCaF 2 nanocomposites had flexural strengths in the range of 100–150 MPa, five times higher than the 20–30 MPa for resin-modified glass ionomer (RMGI). The wear scar depth showed an increasing trend with increasing nCaF 2 filler level. Wear of nCaF 2 nanocomposites was within the range of wear for commercial controls. Water-aging decreased the strength of all materials. At 2 years, flexural strength was 94 MPa for nanocomposite with 10% nCaF 2 , 60 MPa with 20% nCaF 2 , and 48 MPa with 30% nCaF 2 . They are 3–6 fold higher than the 15 MPa for RMGI ( p < 0.05). SEM revealed air bubbles and cracks in a RMGI, while composite control and nCaF 2 nanocomposites appeared dense and solid.
Significance
Combining nCaF 2 with glass particles yielded nanocomposites with long-term mechanical properties that were comparable to those of a commercial composite with little F release, and much better than those of RMGI controls. These strong long-term properties, together with their F release being comparable to RMGI as previously reported, indicate that the nCaF 2 nanocomposites are promising for load-bearing and caries-inhibiting restorations.
1
Introduction
Fluoride (F)-releasing restoratives are frequently studied because the F ions could increase the dissolution resistance of the tooth structure, enhance remineralization and hinder demineralization . Efforts have been made to develop and improve glass ionomers, resin-modified glass ionomers, and compomers . One advantage of these materials is that F ions could be incorporated into the tooth to form fluoroapatite or F-enriched hydroxyapatite, both with lower solubility than hydroxyapatite. Another advantage is that while F ions had little effect on Streptococcus mutans viability, high F ion concentrations significantly reduced the acid production of biofilms . These properties are important in view of the fact that recurrent caries is a major reason for restoration failure . The replacement of failed restorations accounts for 50–70% of all restorations that are placed . Replacement dentistry costs $5 billion annually in the U.S. . F-releasing restoratives are promising to addressing this problem by promoting remineralization and inhibiting microbial growth and metabolism .
While useful for low-load-bearing restorations, glass ionomer cements do not have sufficient mechanical properties to be used in large-load-bearing restorations. It was predicted that “the most intractable problem (for glass ionomers) is likely to be lack of strength and toughness” . Resin-modified glass ionomers were developed with improved mechanical strength, less moisture sensitivity, and better clinical handling properties . However, the mechanical enhancement was rather limited. For example, when traditional and resin-modified glass ionomers were immersed in water for 12 months, it was shown that the microhardness was not enhanced with the addition of resins . Efforts are being made to improve the F-releasing materials . Resin composites possess good mechanical properties and wear resistance . Therefore, one approach to improving the load-bearing capability of F-releasing restorations is to incorporate F-releasing fillers in resin composites.
Calcium fluoride nanoparticles (nCaF 2 ) were recently synthesized via a spray-drying technique and incorporated into resin composite . The composite containing 20% nCaF 2 had a cumulative F release of 2.34 mmol/L at 10 weeks . The initial F release rate was 2 μg/(h cm 2 ), and the sustained release-rate at 10 weeks was 0.29 μg/(h cm 2 ). These values matched or exceeded the reported releases of traditional and resin-modified glass ionomers . Immersion in solutions of pH 4–7 yielded similar strengths for the nCaF 2 nanocomposites, which were significantly higher than the strengths of commercial F-releasing controls . In addition, the nCaF 2 nanocomposite was smart and greatly increased the F release at cariogenic low pH when these ions are most needed to inhibit caries . However, the long-term mechanical durability of the nCaF 2 nanocomposites has not been reported.
The objective of this study was to investigate the thermal-cycling, three-body wear, and 2-year water-aging behavior of the nCaF 2 nanocomposites vs. nCaF 2 filler level. It was hypothesized that: (1) increasing the nCaF 2 filler level will decrease the mechanical durability of the nanocomposite and increase the wear amount; (2) the nCaF 2 nanocomposites will have less wear than the commercial controls; (3) nCaF 2 nanocomposites will possess higher mechanical properties than commercial controls after thermal-cycling or water-aging for 2 years.
2
Materials and methods
2.1
Fabrication of nCaF 2 nanocomposites
A spray-drying apparatus described recently was used to synthesize the nCaF 2 . Briefly, a two-liquid nozzle (ViscoMist, Lechler, St. Charles, IL) was employed to allow two solutions to be mixed at the time of atomization. Calcium hydroxide, Ca(OH) 2 , was used to make the calcium solution . Ammonium fluoride, NH 4 F, was used to prepare the fluoride solution. The two-liquid nozzle sprayed the solution into the heated chamber of the spay-dryer. The reaction of Ca(OH) 2 and NH 4 F led to: Ca(OH) 2 + 2NH 4 F → CaF 2 + 2NH 3 ↑ + 2H 2 O↑. The CaF 2 nanoparticles were collected via an electrostatic precipitator (MistBuster, Air Quality Eng., Minneapolis, MN). The NH 3 and H 2 O vapors were removed with the air flow. The nanopowder was confirmed to be CaF 2 by X-ray diffraction in a previous study . The nanopowder was dried in air overnight at 110 °C, and multipoint BET particle surface area analyses were performed (AUTOSORB-1, Quantachrome, Boynton Beach, FL) with ultra-high-purity nitrogen as the adsorbate gas and liquid nitrogen as the cryogen. Transmission electron microscopy (TEM, 3010-HREM, JEOL, Peabody, MA) was used to examine the particles.
A monomer consisting of 48.975% Bis-GMA (bisphenol glycidyl dimethacrylate), 48.975% TEGDMA (triethylene glycol dimethacrylate), 0.05% 2,6-di- tert -butyl-4-methylphenol, and 2% benzoyl peroxide formed part I, the initiator, of a two-part chemically activated resin . Part II, the accelerator resin, consisted of 49.5% Bis-GMA, 49.5% TEGDMA, and 1.0% N , N -dihydroxyethyl- p -toluidine. The two-part chemically activated system was used because the nCaF 2 paste was relatively opaque. Besides nCaF 2 fillers, barium boroaluminosilicate glass particles of a median size of 1.4 μm (Caulk/Dentsply, Milford, DE) were used as a co-filler for mechanical reinforcement. Glass particles were silanized with 4% (all mass%, unless otherwise noted) 3-methacryloxypropyltrimethoxysilane and 2% n -propylamine . Four composites were fabricated with the following fillers: (1) 0% nCaF 2 + 65% glass; (2) 10% nCaF 2 + 55% glass; (3) 20% nCaF 2 + 45% glass; (4) 30% nCaF 2 + 35% glass. nCaF 2 filler levels of 40% or higher were not used because a previous study showed that the composite with 20% nCaF 2 had F release comparable to that of a resin-modified glass ionomer. In addition, it is desirable to have at least 35% of glass fillers for reinforcement. Each resin was mixed with the filler particles, and then equal masses of paste I and paste II were mixed to form the chemically cured composite. For thermal-cycling and water-aging, the mold dimensions were 2 mm × 2 mm × 25 mm. For wear, the mold cavity had a 4-mm diameter and 3-mm depth . Specimens were incubated at 37 °C in a humidor for 1 d prior to treatments as described in subsequent sections.
In addition, three commercial materials were tested as comparative controls. A composite with glass nanoparticles of 40–200 nm (Heliomolar, Ivoclar Vivadent, Amherst, NY) is referred to as “Composite control”. The fillers were silica and ytterbium-trifluoride with a filler level of 66.7%. Heliomolar is indicated for Class I and Class II restorations in the posterior region, and Class III–V restorations. A resin-modified glass ionomer (Vitremer, 3 M ESPE, St. Paul, MN) is referred to as “RMGI V”. It consisted of fluoroaluminosilicate glass, and a light-sensitive, aqueous polyalkenoic acid. Indications include Classes III, V and root-caries restoration, Classes I and II in primary teeth, and core-buildup. A powder/liquid ratio of 2.5/1 was used (filler mass fraction = 71.4%) according to the manufacturer. Another resin-modified glass ionomer (Ketac Nano, 3 M) is referred to as “RMGI K”. It consisted of polycarboxilic acid modified with methacrylate groups and fluoroaluminosilicate glass, with a filler level of 69%. It is a two-part, paste/paste system and dispensed using the Clicker Dispensing System. It is recommended for primary teeth restorations, small Class I restorations, and Class III and V restorations. The specimens were photo-cured (Triad 2000, Dentsply, York, PA) for 1 min on each open side. Specimens were incubated in a humidor at 37 °C for 1 d prior to the treatments described below.
2.2
Thermal-cycling test
A computer-controlled two-temperature thermal-cycler was used at the Paffenbarger Research Center in the National Institute of Standards and Technology. Two water baths were maintained at temperatures of 5 °C and 60 °C, respectively. The seven materials were treated with 10 5 thermal cycles. Each cycle consisted of 15 s (s) immersion in each water bath and a travel time of 8 s . The two temperatures were chosen to approximate the minimum and maximum temperatures found in the oral cavity. The water baths were constantly stirred with stirrers, and the variation in the temperature of each water bath was within 1 °C of the set temperature. All specimens for this test were first immersed in distilled water at 37 °C for 1 d. Then the specimens were divided into two groups. Group 1 had no thermal-cycling and was fractured in three-point flexure. Group 1 is designated as “before thermal-cycling”. Group 2 was subjected to thermal-cycling as described above, and then fractured in three-point flexure. Group 2 is referred to as “after thermal-cycling”. The specimens of both groups were tested in three-point flexure in the same manner.
2.3
Flexural testing
A three-point flexural test was used to measure the flexural strength and elastic modulus on a computer-controlled Universal Testing Machine at a crosshead-speed of 1 mm/min and a 20-mm span (5500 R, MTS, Cary, NC). Flexural strength was calculated as: S = 3 P max L /(2 bh 2 ), where P max is the fracture load, L is span, b is specimen width and h is thickness. Elastic modulus was calculated as: E = ( P / d )( L 3 /[4 bh 3 ]), where load P divided by displacement d is the slope of the load–displacement curve in the linear elastic region.
2.4
Three-body wear test
Wear was tested using a four-station wear apparatus (Caulk/Dentsply, Milford, DE) , similar to that previously described . Each composite disk (diameter = 4 mm, thickness = 3 mm) was surrounded by a brass ring filled with a water slurry containing 63% of polymethyl methacrylate (PMMA) beads (mean size = 44 μm). A carbide steel pin with a tip diameter of 3 mm was loaded onto the specimen, which was submerged in the slurry of PMMA beads in each of the four stations. The pin was pressed down against the PMMA beads on the specimen surface and rotated 30°. Upon reaching a maximum load of 76 N, the pin was counter-rotated during unloading and moved upward back to its original position. Each specimen was subjected to 4 × 10 5 wear cycles following previous studies . This type of wear produced a “dimple-like” wear scar into the specimen surface. The diameter and depth of each wear scar were measured via a computer-controlled profilometer (Mahr, Cincinnati, OH) equipped with a 5 μm diamond stylus. For each worn impression, profilometric tracings were made at intervals of 50 μm in two directions perpendicular to each other, with the unworn surface of the specimen as the baseline. The maximum values in the two perpendicular directions were then averaged to yield the maximum wear scar depth and the maximum diameter for each wear scar .
2.5
Water-aging
Specimens were immersed in distilled water at 37 °C for 0 d, 1 d, 1 month, 3 months, 6 months, 12 months, 18 months, and 24 months. The 0 d group refers to specimens that were incubated at 37 °C for 1 d in a humidor without immersion and then tested in flexure. For the immersion specimens, each group of six specimens of the same material was immersed in 200 mL of water in a sealed polyethylene container, following a previous study . The water was changed once every week. At the end of each time period, the specimens were fractured using the aforementioned three-point flexural test to measure the flexural strength and elastic modulus. The immersed bars were tested within a few minutes after being taken out of the water and fractured while being wet.
2.6
SEM and statistics
Scanning electron microscopy (SEM) was used to examine the specimens after water-aging treatment. Both the specimen external surfaces and the fractured cross-sections were sputter-coated with platinum and palladium, and examined in a SEM (Quanta 200, FEI Company, Hillsboro, OR).
One-way and two-way ANOVA were performed to detect the significant effects of the variables. Tukey’s multiple comparison test was used to compare the data at a p value of 0.05.
2
Materials and methods
2.1
Fabrication of nCaF 2 nanocomposites
A spray-drying apparatus described recently was used to synthesize the nCaF 2 . Briefly, a two-liquid nozzle (ViscoMist, Lechler, St. Charles, IL) was employed to allow two solutions to be mixed at the time of atomization. Calcium hydroxide, Ca(OH) 2 , was used to make the calcium solution . Ammonium fluoride, NH 4 F, was used to prepare the fluoride solution. The two-liquid nozzle sprayed the solution into the heated chamber of the spay-dryer. The reaction of Ca(OH) 2 and NH 4 F led to: Ca(OH) 2 + 2NH 4 F → CaF 2 + 2NH 3 ↑ + 2H 2 O↑. The CaF 2 nanoparticles were collected via an electrostatic precipitator (MistBuster, Air Quality Eng., Minneapolis, MN). The NH 3 and H 2 O vapors were removed with the air flow. The nanopowder was confirmed to be CaF 2 by X-ray diffraction in a previous study . The nanopowder was dried in air overnight at 110 °C, and multipoint BET particle surface area analyses were performed (AUTOSORB-1, Quantachrome, Boynton Beach, FL) with ultra-high-purity nitrogen as the adsorbate gas and liquid nitrogen as the cryogen. Transmission electron microscopy (TEM, 3010-HREM, JEOL, Peabody, MA) was used to examine the particles.
A monomer consisting of 48.975% Bis-GMA (bisphenol glycidyl dimethacrylate), 48.975% TEGDMA (triethylene glycol dimethacrylate), 0.05% 2,6-di- tert -butyl-4-methylphenol, and 2% benzoyl peroxide formed part I, the initiator, of a two-part chemically activated resin . Part II, the accelerator resin, consisted of 49.5% Bis-GMA, 49.5% TEGDMA, and 1.0% N , N -dihydroxyethyl- p -toluidine. The two-part chemically activated system was used because the nCaF 2 paste was relatively opaque. Besides nCaF 2 fillers, barium boroaluminosilicate glass particles of a median size of 1.4 μm (Caulk/Dentsply, Milford, DE) were used as a co-filler for mechanical reinforcement. Glass particles were silanized with 4% (all mass%, unless otherwise noted) 3-methacryloxypropyltrimethoxysilane and 2% n -propylamine . Four composites were fabricated with the following fillers: (1) 0% nCaF 2 + 65% glass; (2) 10% nCaF 2 + 55% glass; (3) 20% nCaF 2 + 45% glass; (4) 30% nCaF 2 + 35% glass. nCaF 2 filler levels of 40% or higher were not used because a previous study showed that the composite with 20% nCaF 2 had F release comparable to that of a resin-modified glass ionomer. In addition, it is desirable to have at least 35% of glass fillers for reinforcement. Each resin was mixed with the filler particles, and then equal masses of paste I and paste II were mixed to form the chemically cured composite. For thermal-cycling and water-aging, the mold dimensions were 2 mm × 2 mm × 25 mm. For wear, the mold cavity had a 4-mm diameter and 3-mm depth . Specimens were incubated at 37 °C in a humidor for 1 d prior to treatments as described in subsequent sections.
In addition, three commercial materials were tested as comparative controls. A composite with glass nanoparticles of 40–200 nm (Heliomolar, Ivoclar Vivadent, Amherst, NY) is referred to as “Composite control”. The fillers were silica and ytterbium-trifluoride with a filler level of 66.7%. Heliomolar is indicated for Class I and Class II restorations in the posterior region, and Class III–V restorations. A resin-modified glass ionomer (Vitremer, 3 M ESPE, St. Paul, MN) is referred to as “RMGI V”. It consisted of fluoroaluminosilicate glass, and a light-sensitive, aqueous polyalkenoic acid. Indications include Classes III, V and root-caries restoration, Classes I and II in primary teeth, and core-buildup. A powder/liquid ratio of 2.5/1 was used (filler mass fraction = 71.4%) according to the manufacturer. Another resin-modified glass ionomer (Ketac Nano, 3 M) is referred to as “RMGI K”. It consisted of polycarboxilic acid modified with methacrylate groups and fluoroaluminosilicate glass, with a filler level of 69%. It is a two-part, paste/paste system and dispensed using the Clicker Dispensing System. It is recommended for primary teeth restorations, small Class I restorations, and Class III and V restorations. The specimens were photo-cured (Triad 2000, Dentsply, York, PA) for 1 min on each open side. Specimens were incubated in a humidor at 37 °C for 1 d prior to the treatments described below.
2.2
Thermal-cycling test
A computer-controlled two-temperature thermal-cycler was used at the Paffenbarger Research Center in the National Institute of Standards and Technology. Two water baths were maintained at temperatures of 5 °C and 60 °C, respectively. The seven materials were treated with 10 5 thermal cycles. Each cycle consisted of 15 s (s) immersion in each water bath and a travel time of 8 s . The two temperatures were chosen to approximate the minimum and maximum temperatures found in the oral cavity. The water baths were constantly stirred with stirrers, and the variation in the temperature of each water bath was within 1 °C of the set temperature. All specimens for this test were first immersed in distilled water at 37 °C for 1 d. Then the specimens were divided into two groups. Group 1 had no thermal-cycling and was fractured in three-point flexure. Group 1 is designated as “before thermal-cycling”. Group 2 was subjected to thermal-cycling as described above, and then fractured in three-point flexure. Group 2 is referred to as “after thermal-cycling”. The specimens of both groups were tested in three-point flexure in the same manner.
2.3
Flexural testing
A three-point flexural test was used to measure the flexural strength and elastic modulus on a computer-controlled Universal Testing Machine at a crosshead-speed of 1 mm/min and a 20-mm span (5500 R, MTS, Cary, NC). Flexural strength was calculated as: S = 3 P max L /(2 bh 2 ), where P max is the fracture load, L is span, b is specimen width and h is thickness. Elastic modulus was calculated as: E = ( P / d )( L 3 /[4 bh 3 ]), where load P divided by displacement d is the slope of the load–displacement curve in the linear elastic region.
2.4
Three-body wear test
Wear was tested using a four-station wear apparatus (Caulk/Dentsply, Milford, DE) , similar to that previously described . Each composite disk (diameter = 4 mm, thickness = 3 mm) was surrounded by a brass ring filled with a water slurry containing 63% of polymethyl methacrylate (PMMA) beads (mean size = 44 μm). A carbide steel pin with a tip diameter of 3 mm was loaded onto the specimen, which was submerged in the slurry of PMMA beads in each of the four stations. The pin was pressed down against the PMMA beads on the specimen surface and rotated 30°. Upon reaching a maximum load of 76 N, the pin was counter-rotated during unloading and moved upward back to its original position. Each specimen was subjected to 4 × 10 5 wear cycles following previous studies . This type of wear produced a “dimple-like” wear scar into the specimen surface. The diameter and depth of each wear scar were measured via a computer-controlled profilometer (Mahr, Cincinnati, OH) equipped with a 5 μm diamond stylus. For each worn impression, profilometric tracings were made at intervals of 50 μm in two directions perpendicular to each other, with the unworn surface of the specimen as the baseline. The maximum values in the two perpendicular directions were then averaged to yield the maximum wear scar depth and the maximum diameter for each wear scar .
2.5
Water-aging
Specimens were immersed in distilled water at 37 °C for 0 d, 1 d, 1 month, 3 months, 6 months, 12 months, 18 months, and 24 months. The 0 d group refers to specimens that were incubated at 37 °C for 1 d in a humidor without immersion and then tested in flexure. For the immersion specimens, each group of six specimens of the same material was immersed in 200 mL of water in a sealed polyethylene container, following a previous study . The water was changed once every week. At the end of each time period, the specimens were fractured using the aforementioned three-point flexural test to measure the flexural strength and elastic modulus. The immersed bars were tested within a few minutes after being taken out of the water and fractured while being wet.
2.6
SEM and statistics
Scanning electron microscopy (SEM) was used to examine the specimens after water-aging treatment. Both the specimen external surfaces and the fractured cross-sections were sputter-coated with platinum and palladium, and examined in a SEM (Quanta 200, FEI Company, Hillsboro, OR).
One-way and two-way ANOVA were performed to detect the significant effects of the variables. Tukey’s multiple comparison test was used to compare the data at a p value of 0.05.
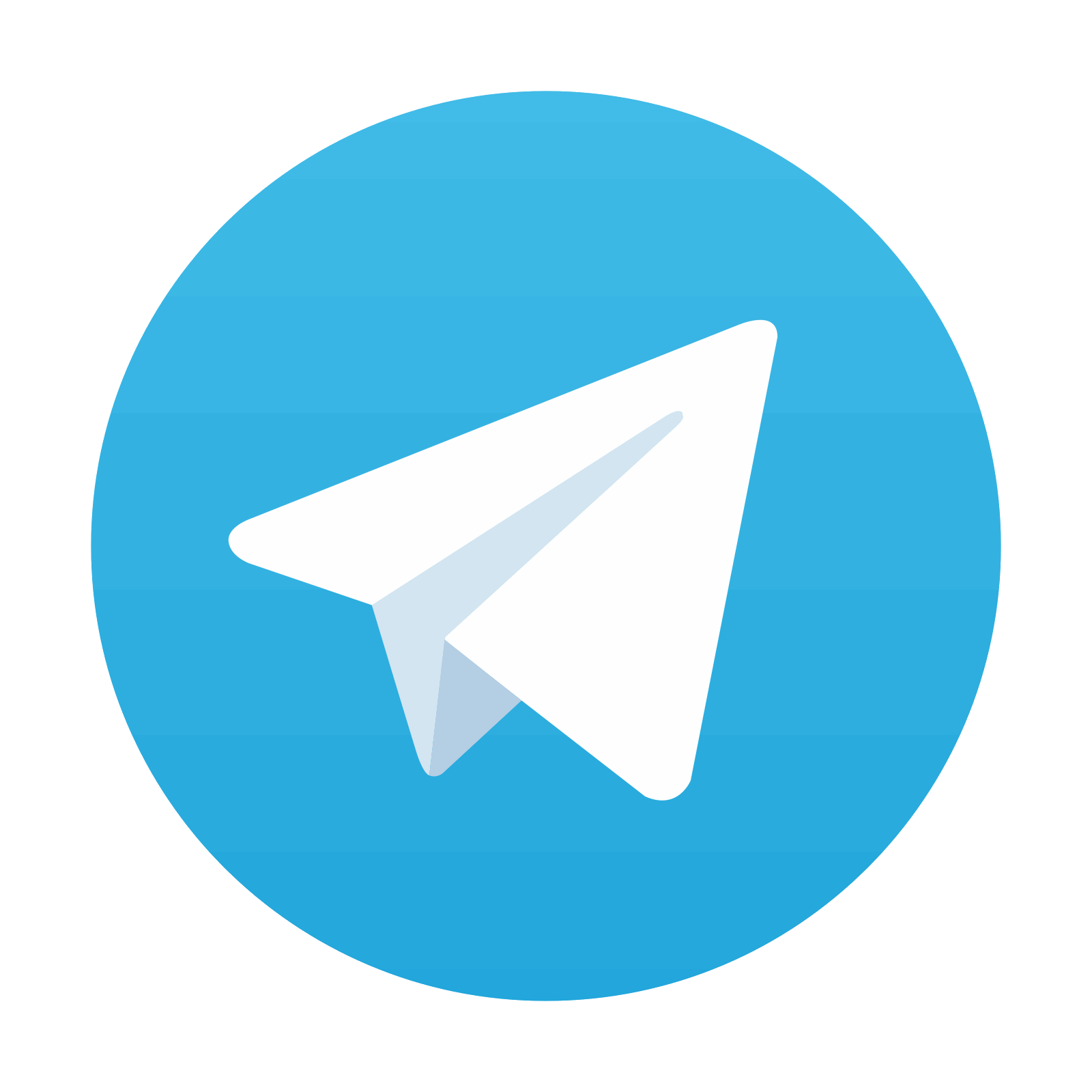
Stay updated, free dental videos. Join our Telegram channel

VIDEdental - Online dental courses
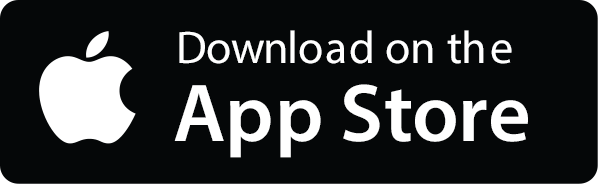
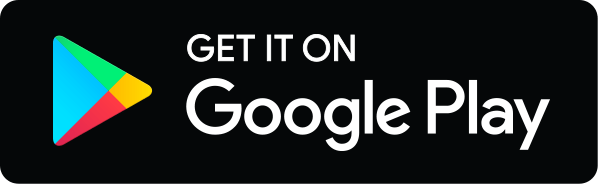