Abstract
Objectives
The main challenges facing composite restorations are secondary caries and bulk fracture. The objectives of this study were to synthesize novel nanoparticles of amorphous calcium phosphate (NACP), develop NACP nanocomposite with calcium (Ca) and phosphate (PO 4 ) ion release to combat caries, and investigate the effects of NACP filler level and glass co-filler reinforcement on composite properties.
Methods
NACP (diameter = 116 nm) were synthesized via a spray-drying technique for the first time. Since the local plaque pH in the oral cavity can decrease to 5 or 4, photo-activated composites were tested with immersion in solutions of pH 7, 5.5, and 4. Composite mechanical properties as well as Ca and PO 4 ion release were measured vs. pH and filler level.
Results
Increasing the NACP filler level increased the ion release. At 28 d and pH 4, the Ca release was (4.66 ± 0.05) mmol/L at 20% NACP, much higher than (0.33 ± 0.08) at 10% NACP ( p < 0.05). Decreasing the pH increased the ion release. At 20% NACP, the PO 4 release at 28 d was (1.84 ± 0.12) mmol/L at pH 4, higher than (0.59 ± 0.08) at pH 5.5, and (0.12 ± 0.01) at pH 7 ( p < 0.05). However, pH had little effect on composite mechanical properties. Flexural strength at 15% NACP was (96 ± 13) MPa at pH 4, similar to (89 ± 13) MPa at pH 5.5, and (89 ± 19) MPa at pH 7 ( p > 0.1). The new NACP nanocomposites had strengths that were 2-fold those of previous calcium phosphate composites and resin-modified glass ionomer control.
Significance
NACP composites were developed for the first time. Their strengths matched or exceeded a commercial composite with little ion release, and were 2-fold those of previous Ca–PO 4 composites. The nanocomposite was “smart” as it greatly increased the ion release at a cariogenic pH 4, when these ions would be most needed to inhibit caries. Hence, the new NACP composite may be promising for stress-bearing and caries-inhibiting restorations.
1
Introduction
Approximately 200 million dental restorations are placed annually in the United States . Resin composites, consisting of fillers in a polymer matrix, are increasingly popular due to their esthetics, direct-filling ability, and enhanced performance . Previous efforts have significantly improved the resin compositions, fillers types, and cure conditions . However, recent reports showed that “The two main challenges are secondary caries and bulk fracture” . Caries at the restoration margins is a frequent reason for replacement of existing restorations , which accounts for 50–70% of all restorations . Replacement dentistry costs $5 billion/year in the U.S. . Therefore, there is a need for mechanically strong composites with caries-inhibition capabilities.
Calcium phosphate (CaP) particles have been filled into resins to develop composites with remineralizing capabilities . One group of composites contained amorphous calcium phosphate (ACP) fillers, with ACP particles having a median diameter of 7.4 μm . Another study reported an ACP median diameter of 55 μm . These composites are promising because ACP is a precursor that forms initially and then transforms to apatite . Hydroxyapatite [HA: Ca 10 (PO 4 ) 6 (OH) 2 ], the structural prototype of the major mineral component of teeth and bones, is the final stable product in the precipitation of calcium and phosphate ions in neutral or basic solutions . Indeed, the ACP composites released supersaturating levels of calcium (Ca) and phosphate (PO 4 ) ions in aqueous solutions, and effectively remineralized enamel lesions in vitro . One drawback of the ACP composites is that they are mechanically weak, with flexural strength about half that of unfilled resin . Such a low strength was “inadequate to make these composites acceptable as bulk restoratives” . Therefore, there is a need to develop load-bearing ACP composites for a wide range of tooth cavity restorations.
Recently, CaP particles were combined with reinforcing whiskers or glass particles to yield composites with Ca and PO 4 ion release and good mechanical properties . These composites achieved Ca and PO 4 release similar to previous CaP composites, while their mechanical properties nearly matched commercial load-bearing composites without ion release. Another study combined CaF 2 nanoparticles with reinforcing fillers to develop composites with fluoride release and stress-bearing capability . A literature search revealed no report on the synthesis of nanoparticles of ACP (NACP) or dental composites containing NACP.
Therefore, the objective of the present study was to develop the first NACP dental composite with load-bearing and caries-inhibiting capabilities. It was hypothesized that combining NACP with esthetic glass fillers would yield photo-cured composite with high levels of Ca and PO 4 ion release and improved mechanical properties, and the composite would be “smart” to greatly increase the ion release at acidic, cariogenic pH, when these ions are most needed to inhibit caries.
2
Materials and methods
2.1
Synthesis of ACP nanoparticles
A spray-drying technique was used to make NACP. While nanoparticles of HA , dicalcium phosphate anhydrous (CaHPO 4 , or DCPA) and CaF 2 were recently reported, ACP (Ca 3 [PO 4 ] 2 ) nanoparticles are reported here for the first time. ACP is important because it is a precursor that can convert to apatite, similar to the minerals in tooth enamel and dentin. A spraying solution was prepared by adding 1.5125 g of acetic acid glacial (J.T. Baker, Phillipsburg, NJ) into 500 mL of distilled water. Then, 0.8 g of calcium carbonate (CaCO 3 , Fisher, Fair Lawn, NJ) and 5.094 g of DCPA (Baker) were dissolved into the acetic acid solution. This solution was then added with distilled water to a total of 1 L. The final Ca and PO 4 ionic concentrations were 8 mmol/L and 5.333 mmol/L, respectively. This yielded a Ca/P molar ratio of 1.5, the same as that for ACP. The acetic acid concentration was 25 mmol/L. This solution was sprayed at a feed rate of 10 mL/min, through a nozzle (PNR, Poughkeepsie, NY) that was situated on a spray chamber with heated air flow. An electrostatic precipitator (AirQuality, Minneapolis, MN) was connected to the lower end of the column and drew air from the column to create a steady flow of air/mist. The water/volatile acid were evaporated into the dry, heated column and expelled from the precipitator into an exhaust-hood. The dried particles were collected by the electrostatic precipitator.
The collected powder was examined with X-ray diffractometry (XRD, DMAX2200, Rigaku, Woodlands, TX). The specific surface area of the powder was analyzed using a multipoint-BET (Brunauer, Emmet, and Teller) method (AUTOSORB-1, Quantachrome, Boynton Beach, FL) and transmission electron microscopy (TEM, 3010-HREM, JEOL, Peabody, MA).
2.2
Nanocomposite fabrication
Barium boroaluminosilicate glass particles of a median diameter of 1.4 μm (Caulk/Dentsply, Milford, DE) were selected as a co-filler because it is a typical dental glass filler similar to those in a hybrid composite (TPH, Cault/Dentsply). The glass particles were silanized with 4% 3-methacryloxypropyltrimethoxysilane and 2% n-propylamine (mass %) . A resin of Bis-GMA (bisphenol glycidyl dimethacrylate) and TEGDMA (triethylene glycol dimethacrylate) at 1:1 mass ratio was rendered light-curable with 0.2% camphorquinone and 0.8% ethyl 4-N,N-dimethylaminobenzoate.
Four composites were made with the following fillers: (1) 0% NACP + 75% glass (all mass fractions); (2) 10% NACP + 65% glass; (3) 15% NACP + 60% glass; and (4) 20% NACP + 50% glass. The total filler mass fraction was 75% except for (4) which had 70%. This was because with 20% NACP, the paste was relatively dry at 75% total filler level. NACP filler levels higher than 20% were not used in order for the NACP nanocomposite to have mechanical properties matching/exceeding a commercial control composite. The fillers and resin were mixed and the paste was placed into a stainless steel mold of 2 mm × 2 mm × 25 mm. The specimen was photo-cured (Triad 2000, Dentsply, York, PA) for 1 min on each side. All the specimens were cured in air. The specimens were then incubated at 37 °C for 24 h prior to testing.
Two commercial materials were used as comparative controls. A composite with nano-fillers (40–200 nm) and a low level of fluoride release served as a control (Heliomolar, Ivoclar, Ontario, Canada). The fillers consisted of silica and ytterbium–trifluoride (total filler mass fraction = 66.7%). Heliomolar is indicated for Class I and Class II restorations in the posterior region, Class III and Class IV anterior restorations, Class V restorations, and pit and fissure sealing in molar and premolar teeth. A resin-modified glass ionomer (Vitremer, 3M, St. Paul, MN), another control, consisted of fluoroaluminosilicate glass, and a light-sensitive, aqueous polyalkenoic acid. Indications include Classes III, V and root-caries restoration, Classes I and II in primary teeth, and core-buildup. A powder/liquid ratio of 2.5/1 was used (filler mass fraction = 71.4%) according to the manufacturer. Both materials were photo-cured.
2.3
Flexural testing
Flexural strength and elastic modulus were measured using a three-point flexural test with a 20-mm span at a crosshead-speed of 1 mm/min on a computer-controlled Universal Testing Machine (5500R, MTS, Cary, NC). Flexural strength was calculated by: S = 3 P max L /(2 bh 2 ), where P max is the fracture load, L is span, b is specimen width and h is thickness. Elastic modulus was calculated by: E = ( P / d )( L 3 /[4 bh 3 ]), where load P divided by displacement d is the slope of the load-displacement curve in the linear elastic region.
2.4
Ca and PO 4 ion release
A sodium chloride (NaCl) solution (133 mmol/L) was buffered to three different pHs: pH 4 with 50 mmol/L lactic acid, pH 5.5 with 50 mmol/L acetic acid, and pH 7 with 50 mmol/L HEPES. Following previous studies , three specimens of approximately 2 mm × 2 mm × 12 mm were immersed in 50 mL of solution at each pH, yielding a specimen volume/solution of 2.9 mm 3 /mL. This compared to a specimen volume per solution of approximately 3.0 mm 3 /mL in a previous study . For each solution, the concentrations of Ca and PO 4 released from the specimens were measured at 1, 3, 7, 14, 21, and 28 days (d). At each time, aliquots of 0.5 mL were removed and replaced by fresh solution. The aliquots were analyzed for Ca and PO 4 via a spectrophotometric method (DMS-80 UV-visible, Varian, Palo Alto, CA) using known standards and calibration curves . The released ions were reported in cumulative concentrations.
One-way and two-way ANOVA were performed to detect the significant effects of the variables. Tukey’s multiple comparison test was used to compare the data at a p -value of 0.05.
2
Materials and methods
2.1
Synthesis of ACP nanoparticles
A spray-drying technique was used to make NACP. While nanoparticles of HA , dicalcium phosphate anhydrous (CaHPO 4 , or DCPA) and CaF 2 were recently reported, ACP (Ca 3 [PO 4 ] 2 ) nanoparticles are reported here for the first time. ACP is important because it is a precursor that can convert to apatite, similar to the minerals in tooth enamel and dentin. A spraying solution was prepared by adding 1.5125 g of acetic acid glacial (J.T. Baker, Phillipsburg, NJ) into 500 mL of distilled water. Then, 0.8 g of calcium carbonate (CaCO 3 , Fisher, Fair Lawn, NJ) and 5.094 g of DCPA (Baker) were dissolved into the acetic acid solution. This solution was then added with distilled water to a total of 1 L. The final Ca and PO 4 ionic concentrations were 8 mmol/L and 5.333 mmol/L, respectively. This yielded a Ca/P molar ratio of 1.5, the same as that for ACP. The acetic acid concentration was 25 mmol/L. This solution was sprayed at a feed rate of 10 mL/min, through a nozzle (PNR, Poughkeepsie, NY) that was situated on a spray chamber with heated air flow. An electrostatic precipitator (AirQuality, Minneapolis, MN) was connected to the lower end of the column and drew air from the column to create a steady flow of air/mist. The water/volatile acid were evaporated into the dry, heated column and expelled from the precipitator into an exhaust-hood. The dried particles were collected by the electrostatic precipitator.
The collected powder was examined with X-ray diffractometry (XRD, DMAX2200, Rigaku, Woodlands, TX). The specific surface area of the powder was analyzed using a multipoint-BET (Brunauer, Emmet, and Teller) method (AUTOSORB-1, Quantachrome, Boynton Beach, FL) and transmission electron microscopy (TEM, 3010-HREM, JEOL, Peabody, MA).
2.2
Nanocomposite fabrication
Barium boroaluminosilicate glass particles of a median diameter of 1.4 μm (Caulk/Dentsply, Milford, DE) were selected as a co-filler because it is a typical dental glass filler similar to those in a hybrid composite (TPH, Cault/Dentsply). The glass particles were silanized with 4% 3-methacryloxypropyltrimethoxysilane and 2% n-propylamine (mass %) . A resin of Bis-GMA (bisphenol glycidyl dimethacrylate) and TEGDMA (triethylene glycol dimethacrylate) at 1:1 mass ratio was rendered light-curable with 0.2% camphorquinone and 0.8% ethyl 4-N,N-dimethylaminobenzoate.
Four composites were made with the following fillers: (1) 0% NACP + 75% glass (all mass fractions); (2) 10% NACP + 65% glass; (3) 15% NACP + 60% glass; and (4) 20% NACP + 50% glass. The total filler mass fraction was 75% except for (4) which had 70%. This was because with 20% NACP, the paste was relatively dry at 75% total filler level. NACP filler levels higher than 20% were not used in order for the NACP nanocomposite to have mechanical properties matching/exceeding a commercial control composite. The fillers and resin were mixed and the paste was placed into a stainless steel mold of 2 mm × 2 mm × 25 mm. The specimen was photo-cured (Triad 2000, Dentsply, York, PA) for 1 min on each side. All the specimens were cured in air. The specimens were then incubated at 37 °C for 24 h prior to testing.
Two commercial materials were used as comparative controls. A composite with nano-fillers (40–200 nm) and a low level of fluoride release served as a control (Heliomolar, Ivoclar, Ontario, Canada). The fillers consisted of silica and ytterbium–trifluoride (total filler mass fraction = 66.7%). Heliomolar is indicated for Class I and Class II restorations in the posterior region, Class III and Class IV anterior restorations, Class V restorations, and pit and fissure sealing in molar and premolar teeth. A resin-modified glass ionomer (Vitremer, 3M, St. Paul, MN), another control, consisted of fluoroaluminosilicate glass, and a light-sensitive, aqueous polyalkenoic acid. Indications include Classes III, V and root-caries restoration, Classes I and II in primary teeth, and core-buildup. A powder/liquid ratio of 2.5/1 was used (filler mass fraction = 71.4%) according to the manufacturer. Both materials were photo-cured.
2.3
Flexural testing
Flexural strength and elastic modulus were measured using a three-point flexural test with a 20-mm span at a crosshead-speed of 1 mm/min on a computer-controlled Universal Testing Machine (5500R, MTS, Cary, NC). Flexural strength was calculated by: S = 3 P max L /(2 bh 2 ), where P max is the fracture load, L is span, b is specimen width and h is thickness. Elastic modulus was calculated by: E = ( P / d )( L 3 /[4 bh 3 ]), where load P divided by displacement d is the slope of the load-displacement curve in the linear elastic region.
2.4
Ca and PO 4 ion release
A sodium chloride (NaCl) solution (133 mmol/L) was buffered to three different pHs: pH 4 with 50 mmol/L lactic acid, pH 5.5 with 50 mmol/L acetic acid, and pH 7 with 50 mmol/L HEPES. Following previous studies , three specimens of approximately 2 mm × 2 mm × 12 mm were immersed in 50 mL of solution at each pH, yielding a specimen volume/solution of 2.9 mm 3 /mL. This compared to a specimen volume per solution of approximately 3.0 mm 3 /mL in a previous study . For each solution, the concentrations of Ca and PO 4 released from the specimens were measured at 1, 3, 7, 14, 21, and 28 days (d). At each time, aliquots of 0.5 mL were removed and replaced by fresh solution. The aliquots were analyzed for Ca and PO 4 via a spectrophotometric method (DMS-80 UV-visible, Varian, Palo Alto, CA) using known standards and calibration curves . The released ions were reported in cumulative concentrations.
One-way and two-way ANOVA were performed to detect the significant effects of the variables. Tukey’s multiple comparison test was used to compare the data at a p -value of 0.05.
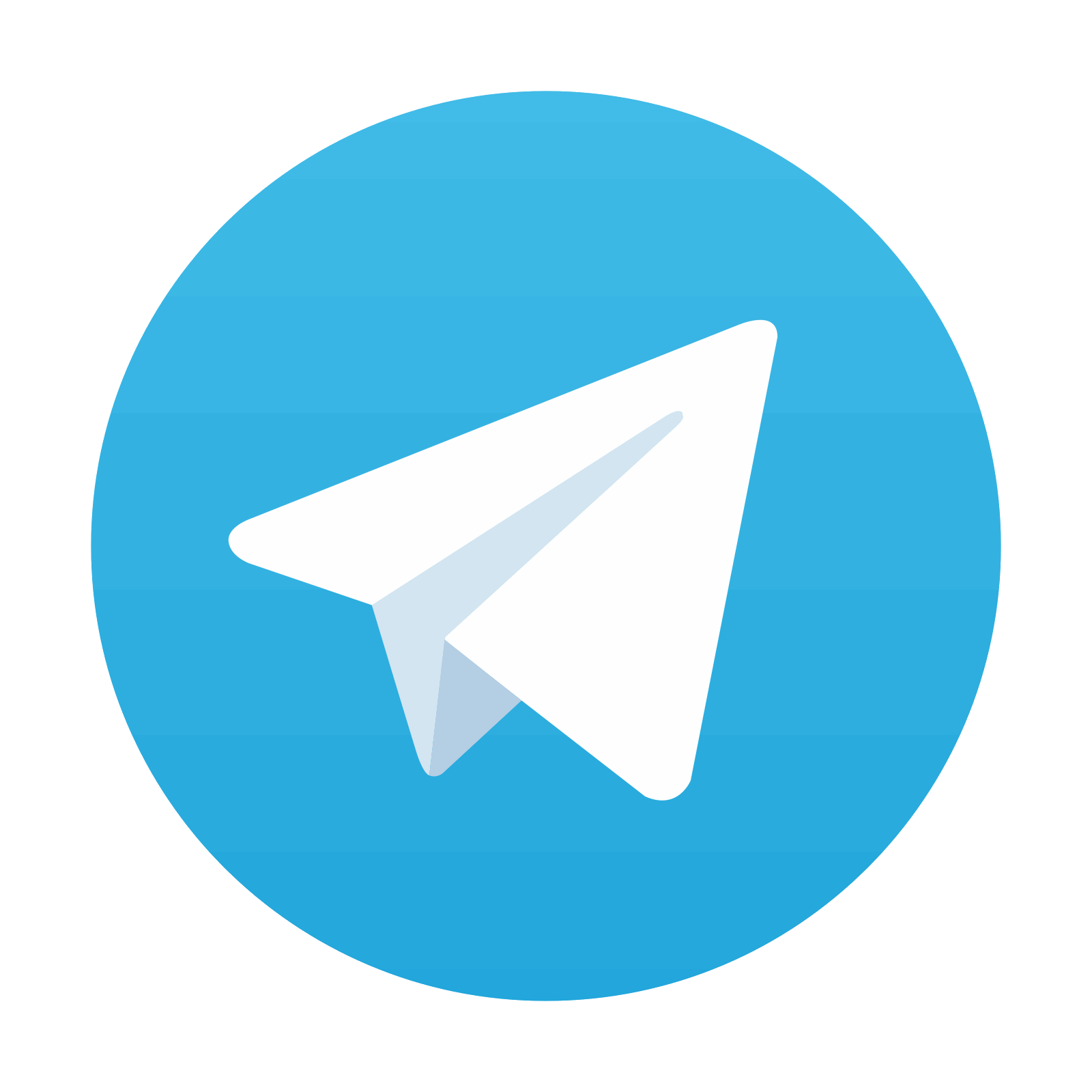
Stay updated, free dental videos. Join our Telegram channel

VIDEdental - Online dental courses
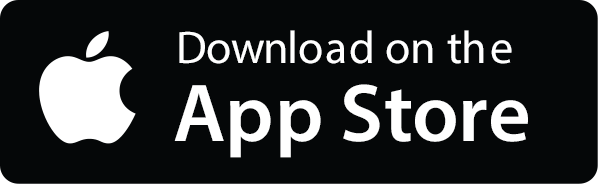
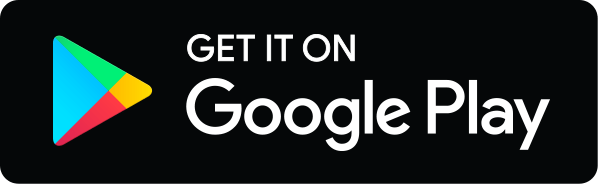