Graphical abstract

Highlights
- •
Osteogenic differentiation of hDPSCs was enhanced after exposed to White MTA or Quick-Set2.
- •
White MTA displayed better mineralogenic stimulation capacity than Quick-Set2 on hDPSCs.
- •
Intracellular mineral-containing vesicles were found in differentiated hDPSCs.
Abstract
Objectives
An experimental discoloration-free calcium aluminosilicate cement has been developed with the intention of maximizing the beneficial attributes of tricalcium silicate cements and calcium aluminate cements. The present study examined the effects of this experimental cement (Quick-Set2) on the mineralogenic characteristics of osteogenic lineage-committed human dental pulp stem cells (hDPSCs), by comparing the cellular responses with a commercially available tricalcium silicate cement (white mineral trioxide aggregate (ProRoot ® MTA); WMTA).
Methods
The osteogenic potential of hDPSCs exposed to the cements was examined using qRT-PCR for osteogenic gene expressions, Western blot for osteogenic-related protein expressions, alkaline phosphatase enzyme activity, Alizarin red S staining, Fourier transform infrared spectroscopy and transmission electron microscopy of extracellular calcium deposits.
Results
Results of the six assays indicated that osteogenic differentiation of hDPSCs was significantly enhanced after exposure to the tricalcium silicate cement or the experimental calcium aluminosilicate cement, with the former demonstrating better mineralogenic stimulation capacity.
Significance
The better osteogenic stimulating effect of the tricalcium silicate cement on hDPSCs may be due to its relatively higher silicate content, or higher OH − and Ca 2+ release. Further investigations with the use of in vivo animal models are required to validate the potential augmenting osteogenic effects of the experimental discoloration-free calcium aluminosilicate cement.
1
Introduction
Tricalcium silicate-based hydraulic cements such as mineral trioxide aggregate (MTA)-based cements are currently the material of choice for both surgical and complex non-surgical root canal treatment . These hydraulic cements are used as root-end filling materials following apicoectomy, perforation repair below the alveolar crest, in vital pulp therapy for direct pulp capping or apexogenesis of immature teeth, as an apical barrier in apexification, and as a coronal barrier in regenerative endodontic procedures. In terms of biocompatibility, tricalcium silicate cements have outperformed other materials used for the aforementioned endodontic procedures . MTA is hard tissue-inductive and conductive, possessing the ability to stimulate type I collagen synthesis and dentinogenesis/osteogenesis from mesenchymal stem cells for healing of pulpal and periapical tissues . Despite these benefits, the first commercial MTA products were far from optimal in terms of their dark color, poor handling characteristics , long setting time and washout susceptibility . Furthermore, even the light-colored (white) versions of some tricalcium silicate products have been documented to cause discoloration of root and crown dentin when the material comes into contact with sodium hypochlorite, chlorhexidine or blood , and also in pediatric pulpotomies over time . Such discoloration compromises the esthetics in anterior teeth and has been attributed to the inclusion of bismuth oxide as the radiopacifier in the MTA products . Another problem with tricalcium silicate cements is their susceptibility to acid attack. Acidic environments such as those present in the vicinity of periradicular infections prevent the cement from setting, and use supragingivally such as treatment for cervical resorption. The acidic environment also causes dissolution of the calcium hydroxide phase of the cement, resulting in porosity .
Other ceramic cements have been tested in attempts to overcome some of the shortcomings of tricalcium silicate cements. Calcium aluminate cements, based on calcium mono- and dialuminate, are inherently more acid-resistant than tricalcium silicates certain tricalcium aluminate cements exhibit better fluidity, shorter setting time, improved handling properties and reduced porosity compared with tricalcium silicate cements . Calcium aluminate compositions support better differentiation of osteogenic cells compared with similar cells exposed to MTA . A biomembrane composed of calcium aluminate cement embedded in chitosan and collagen induced more optimal differentiation of human pulp stem cells into odontoblast-like cells that exhibited a highly secretory phenotype . Both EndoBinder (Binderware, São Carlos, SP, Brazil), a calcium aluminate cement, and MTA, when employed as one defect fillers, enabled complete repair of intentionally-created bone defects in rat tibias . However, mono/di calcium aluminate cements lack silica, have a slightly lower pH (fewer hydroxide ions) than the tricalcium silicates and release fewer calcium ions .
Aluminosilicate cements have been developed with the intention of maximizing the beneficial attributes of tricalcium silicate cements and calcium aluminate cements . The Quick-Set2 hybrid cement (Primus Consulting, Bradenton, FL, USA) contains a silicate phase and the calcium mono- and dialuminate phases. The aluminate phases in these hybrid cements confer acid resistance and quick setting time, while the silicate component is added to support mineralogic responses. In this experimental material, free alumina (Al 2 O 3 ) and bismuth oxide has been eliminated from the earlier Quick-Set material. The absence of free alumina increases the percentage of the hydraulic phase. The rationale for elimination of free alumina is based on the results of previous in vivo pulpotomy and root-end filling studies performed in dogs, showing that free alumina may be a source of irrigation to vital tissues . A radiopaque powder is included in Quick-Set2 to allow differentiation from dentin. Replacement of the bismuth oxide radiopacifier in the original Quick-Set with tentalum pentoxide (Ta 2 O 5 ) in Quick-Set2 eliminates the risk of discoloration .
Hydraulic cements intended to be placed in close proximity with pulpal and periradicular tissues must be biocompatible to expedite reparative dentinogenesis by pulpal stem cells during vital pulp therapy. Such cements should also stimulate osteogenesis in treatment of necrotic teeth with apical periodontitis. Because there has been significant changes in composition from the original version of the cement, including the elimination of free alumina, both the biocompatibility aspects and osteogenic potential of the modified calcium aluminosilicate cement have to be re-examined in order for the experimental cement to be approved by the U.S. Food and Drug Administration prior to commercialization. The biocompatibility of Quick-Set2 calcium aluminosilicate cement has been reported in a recent study . No differences in cytotoxicity were observed between this experimental material and the control (tricalcium silicate cement) after two cycles of aging in deionized water. Both cements were less cytotoxic than a zinc oxide eugenol-based cement when placed in close proximity with undifferentiated human dental pulp stem cells (hDPSCs). The favorable biocompatibility results warrants further evaluation of the effects of the modified calcium aluminosilicate on the osteogenic potential of hDPSCs. Accordingly, the objective of the present study was to examine the effects of water-aged endodontic hydraulic cements on the osteogenic potential of hDPSCs. The null hypothesis tested was that there are no differences in the mineralogenic characteristics of osteogenic lineage-committed hDPSCs after they are exposed to the experimental discoloration-free calcium aluminosilicate hydraulic cement or the control tricalcium silicate hydraulic cement.
2
Materials and methods
2.1
Human dental pulp stem cells
Previously characterized, undifferentiated hDPSCs with CD29 + /CD44 + /CD90 + /CD105 + /CD34 − /CD45 − immunophenotype were employed in the present study . The original cells were harvested from young healthy patients (18–25 years old) according to a protocol approved by the Ethics Committee of the Fourth Military Medical University, Xi’an, China. The majority of the hDPSCs retained their fibroblastoid morphology in colony-forming-unit assay, a feature that was consistent with other mesenchymal stem cell populations. The multipotency of those hDPSCs had been established previously using chrondrogenic, adipogenic and osteogenic culturing conditions.
2.2
Mineralogenic stimulants
Two potential mineralogenic stimulants were employed. They included tricalcium silicate cement (White ProRoot MTA (WMTA); Dentsply Tulsa Dental Specialties, Tulsa, OK, USA) that was used as the control, and the experimental discoloration-free calcium aluminosilicate cement (Quick-Set2). For each cement-based stimulant, the powder was mixed with the proprietary hydrogel or deionized water according to the instructions of the respective manufacturer, using a liquid/powder ratio of 0.3. The mixed materials were placed in Teflon molds (5-mm diameter and 3-mm thick), covered with polyethylene terephthalate sheets, and allowed to set in a 100% humidity chamber for 24 h. Because of the initial cytotoxicity of both cements , set cement disks were incubated in deionized water for two weeks to facilitate outward diffusion of water-soluble cytotoxic components. All set materials were subsequently sterilized with ultraviolet light for 4 h prior to testing.
2.3
Cell culture
Sorted hDPSCs were cultured in complete growth medium consisting of α-modified Eagle medium (Gibco BRL, Gaitherburg, MD, USA) supplemented with 10% fetal bovine serum (Gibco), 100 units/mL penicillin and 100 mg/mL streptomycin (Sigma–Aldrich, St. Louis, MO, USA) in 5% CO 2 at 37 °C. Cultured cells from the third to sixth passages were used for subsequent experiments. The hDPSCs were seeded at 10 5 cells/cm 2 in 24-well plates and incubated at 37 °C in a humidified 5% CO 2 atmosphere. Seeded cells were cultured in complete growth medium to ∼60% confluency prior to the commencement of osteogenic differentiation in the presence of the water-aged cements. The osteogenic medium comprised the original growth medium supplemented with 50 mg/mL ascorbic acid, 10 mmol/L β-glycerophosphate and 100 nmol/L dexamethasone (Sigma–Aldrich). Each cement disk was placed individually inside a Transwell insert with 3-μm pore size (BD Falcon, Franklin Lakes, NJ, USA) to prevent direct cell contact. The cement disks were exposed to the plated cells for 1 week, 2 weeks or 3 weeks, with the osteogenic medium renewed every 3 days. The same procedures were adopted for the virgin hDPSC control, with the exception that no material was placed inside the Transwell insert during osteogenic differentiation.
2.4
Quantitative reverse-transcription polymerase chain reaction (qRT-PCR)
Expression of osteogenic/odontogenic differentiation markers by hDPSCs was examined in sextuplicates ( N = 6). The total RNA present in the hDPSCs derived from each experimental or control group was isolated and purified using RNeasy Mini Kit (QIAGEN, Valencia, CA, USA). Quality control of the extracted RNA was performed with 2 μL samples by measuring their UV absorbance with the NanoDrop ND-1000 UV–vis spectrophotometer (Thermo Fisher Scientific Inc., Waltham, MA, USA). Quantity of extracted RNA was determined from the RNA absorbance at 260 nm; RNA purity was determined from the ratio of the absorbance (A@260 nm/A@280 nm and A@260/A@230 >1.8 and <2.0). Equal amounts of total RNA (0.1 mg/mL) were reverse-transcribed into single-stranded complementary DNA (cDNA) using a High Capacity cDNA Reverse Transcription Kit (Applied Biosystems, Foster City, CA, USA) in a 7300 real-time PCR system (Applied Biosystems). Reverse transcription was performed using 25 °C for 10 min, 37 °C for 120 min, and 85 °C for 5 min. The cDNA was stored at −20 °C until use.
Osteogenic/odontogenic target markers employed for qRT-PCR were runt-related transcription factor 2 ( RUNX2 ), osterix ( OSX ), alkaline phosphatase ( ALP ), bone sialoprotein ( BSP ), osteocalcin ( OCN ), dentin matrix protein-1 ( DMP-1 ) and dentin sialophosphoprotein ( DSPP ). Glyceraldehyde 3-phosphate dehydrogenase ( GAPDH ) was used as the housekeeping gene. A FastStart Universal SYBR Green Master Kit (Roche Applied Science, Indianapolis, IN, USA) was employed for qRT-PCR, using forward and reverse primers for the aforementioned genes (Supplementary material) in the 7300 RT-PCR system. Plates were loaded in the thermocycler and run in regular cycles. Each cycle consisted of 50 °C for 2 min, 95 °C for 10 min, 40 cycles of denaturing/annealing at 95 °C for 15 s and 60 °C for 1 min. The comparative threshold cycle method (ΔΔ C t ) was employed for relative quantification of gene expression. The expression level of each target cDNA marker was normalized to the GAPDH endogenous control. Data output was expressed as fold regulations of mRNA expression levels, given by 2 −ΔΔ C t . Gene expressions of hDPSCs exposed to the two mineralogenic stimulants were compared with those either hydraulic cement.
2.5
Western blot
Target genes that demonstrated significant fold increases in gene expressions were selected for further examination of protein expressions with Western blot. After 1 week, 2 weeks or 3 weeks of exposure to the cement disks in osteogenic medium, the cells were lysed using radioimmunoprecipitation assay buffer with a protease inhibitor cocktail (Sigma–Aldrich). Bicinchoninic acid assay was used to determine the protein concentration per specimen, using bovine serum albumin as standard. Protein denaturing and reduction was accomplished by adding 4X Laemmli sample buffer (Bio-Rad Laboratories, Hercules, CA, USA) to the specimens, after standardization of the total amount of protein to be loaded, followed by boiling the specimens at 100 °C for 5 min.
Total protein from each group (40 mg) was fractionated by sodium dodecyl sulphate-polyacrylamide gel electrophoresis (Criterion electrophoresis cell, Bio-Rad) and transferred onto a nitrocellulose membrane. The latter was blocked with 5% non-fat milk and incubated with primary antibodies (mouse anti-human) against DMP-1 (55.8 kDa; sc-73633, mouse monoclonal IgG 1 , 1:500 dilution, Santa Cruz Biotechnology, Dallas, Texas, USA), DSPP (95 kDa; sc-73632, mouse monoclonal IgG 2b , 1:500 dilution, Santa Cruz), and OCN (72 kDa; sc-365797, mouse monoclonal IgG 3 , 1:300 dilution, Santa Cruz). β-actin (43 kDa; sc-8432, mouse monoclonal, IgG 1 , 1:1000 dilution, Santa Cruz) was used as the loading control. Signals were revealed by incubation with horseradish peroxidase-conjugated secondary antibody (sc-2314, donkey anti-mouse, 1:5000 dilution, Santa Cruz) and enhanced chemiluminescence detection. Experiment was conducted in triplicates. Relative protein expression analyses were performed using the Image Studio™ Lite software (LI-COR Corp., Lincoln, NE, USA).
2.6
Alkaline phosphatase (ALP) activity
Intracellular release of ALP enzyme was measured using the Quantichrom ALP assay kit (Bioassay Systems, Hayward, CA, USA). Colorimetric determination was based on hydrolysis of p -nitrophenyl phosphate by ALP into inorganic phosphate and p -nitrophenol, a yellow-colored product. After exposure of the water-aged cement disks to hDPSCs cultured in osteogenic medium for 1 week, 2 weeks or 3 weeks, the cells were lysed with 0.2% Triton X-100 for 20 min. The cell lysate was mixed with assay buffer. Absorbance of p -nitrophenol at 405 nm was recorded every min for a maximum of 16 min using a microplate reader (Synergy HT, BioTek Instruments, Winooski, VT, USA). The level of ALP activity of the cell lysate in IU/L (μmol/L min) was calculated from the equation: (OD Sample t − OD Sample 0 ) × Reaction vol × 35.3/(OD Calibrator − OD H2O ) × Sample vol × t , where OD is the optical density at 405 nm, t is the time in min, OD Sample0 is the optical density at 0 min. Data were plotted on a graph, from which the peak value for each material was used for comparison among groups ( N = 6).
2.7
Extracellular calcium-containing deposits
Alizarin red S assay was used for qualitative and quantitative examination of the propensity of extracellular calcium-containing mineral deposits. On switching of the hDPSCs to osteogenic medium, the cells were simultaneously exposed to the cement disks for 1 week, 2 weeks or 3 weeks. The hDPSCs were then fixed in 10% formaldehyde and stained with Alizarin red S (40 mM solution; pH 4.2) for 20 min. Images of the specimens were taken for qualitative evaluation of the stained mineral deposits.
The stained calcium deposits were then dissolved in 10% acetic acid for 30 min and neutralized with 10% ammonium hydroxide prior to quantification. Absorbance of the extracts was spectrophotometrically determined at λ = 405 nm. The concentration of dye extracted from the specimens (mg/mL) was determined according to a linear regression equation derived from a calibration curve that correlated absorbance with known dye concentrations. Because one mole of dye binds to two moles of calcium to produce an Alizarin red S–calcium complex , the extent of calcium deposition was expressed as molar equivalent of calcium ( N = 6).
2.8
Attenuated total reflection-Fourier transform infrared spectroscopy (ATR-FTIR)
Extracellular mineralization by hDPSCs exposed to the water-aged cement disks was further analyzed with ATR-FTIR. Cells were cultured in osteogenic medium and exposed to the materials for 1 week, 2 weeks or 3 weeks. The hDPSCs were then fixed with 100% ethanol for 15 min, detached with a cell scraper and desiccated with anhydrous calcium sulphate. A Nicolet 6700 FT-IR spectrophotometer (Thermo Fischer Scientific) with ATR setup was used to collect infrared spectra between 4000–400 cm −1 at 4 cm −1 resolution using 32 scans ( N = 6). The acquired spectra were baseline-corrected and normalized along the collagen amide I peak (∼1635 cm −1 ). The extent of extracellular mineralization was estimated by the phosphate-to-collagen amide I ratio , using the formula: mineral = A900-1200/A1660; where A900-1200 represents the amount of phosphate in the mineralized matrix and A1660 the amount of amide I groups.
2.9
Transmission electron microscopy (TEM)
Lineage-committed hDPSCs from different groups were examined by TEM, at the end of the third week of culture in osteogenic medium, to confirm the formation of intracellular and extracellular mineral deposits. The cells were detached with 0.25% trypsin for 2 min. Pooled dislodged cells were centrifuged and fixed with 0.1 M Na cacodylate buffer (pH 7.2) containing 4% paraformaldehyde and 2% glutaraldehyde. The cell pellets were post-fixed with 1% osmium tetroxide, dehydrated in an ascending ethanol series (30–100%), transferred to propylene oxide and embedded in epoxy resin. Ninety nanometer thick sections were stained with uranyl acetate and Reynold lead citrate, and examined using a JEM-1230 TEM (JEOL, Tokyo, Japan) at 110 kV. Selected area electron diffraction was also performed on the electron-dense mineral deposits using the corresponding unstained sections.
2.10
Statistical analyses
Data sets acquired from each assay were evaluated for their normality (Shapiro–Wilk test) and equal variance assumptions (modified Levene test). If those assumptions appeared to be violated, the data sets were nonlinearly-transformed to satisfy the assumptions prior to the use of parametric statistical methods. For qRT-PCR, two-factor analysis of variance (ANOVA) was used to examine the effects of “material”, “exposure time” and the interaction of these two factors on fold regulations of individual target gene expression. Post-hoc comparisons were performed using Holm–Sidak multiple comparison procedures. Because the chance of finding one or more significant differences in testing 7 hypotheses ( i.e. , 7 genes) with the same specimens (type I error) is 0.3017 (30.17%), the Dunn–Sidak correction was used for controlling the familywise error rate in multiple comparison procedures. Accordingly, the critical value ( α ) was reduced to 0.0073 for each osteogenic marker, so that test results with P < 0.0073 were only considered significantly different from the GADPH endogenous control. Similar tests were used for analyzing the protein levels obtained from Western blot, using Dunn–Sidak correction of the α value to 0.0170 for controlling the familywise error rate in examining three hypotheses (DMP-1, DSPP and OCN expressions) with the same specimens (type I error without correction = 14.26%). For analysis of ALP enzymatic activity, calcium concentration derived from Alizarin red S staining or phosphate-to-amide I ratio derived from ATR-FTIR, two-factor ANOVA and Holm–Sidak multiple comparison procedures were used to examine the effects of “material”, “exposure time” and their interaction on the respective parameter, with statistical significance preset at α = 0.05.
2
Materials and methods
2.1
Human dental pulp stem cells
Previously characterized, undifferentiated hDPSCs with CD29 + /CD44 + /CD90 + /CD105 + /CD34 − /CD45 − immunophenotype were employed in the present study . The original cells were harvested from young healthy patients (18–25 years old) according to a protocol approved by the Ethics Committee of the Fourth Military Medical University, Xi’an, China. The majority of the hDPSCs retained their fibroblastoid morphology in colony-forming-unit assay, a feature that was consistent with other mesenchymal stem cell populations. The multipotency of those hDPSCs had been established previously using chrondrogenic, adipogenic and osteogenic culturing conditions.
2.2
Mineralogenic stimulants
Two potential mineralogenic stimulants were employed. They included tricalcium silicate cement (White ProRoot MTA (WMTA); Dentsply Tulsa Dental Specialties, Tulsa, OK, USA) that was used as the control, and the experimental discoloration-free calcium aluminosilicate cement (Quick-Set2). For each cement-based stimulant, the powder was mixed with the proprietary hydrogel or deionized water according to the instructions of the respective manufacturer, using a liquid/powder ratio of 0.3. The mixed materials were placed in Teflon molds (5-mm diameter and 3-mm thick), covered with polyethylene terephthalate sheets, and allowed to set in a 100% humidity chamber for 24 h. Because of the initial cytotoxicity of both cements , set cement disks were incubated in deionized water for two weeks to facilitate outward diffusion of water-soluble cytotoxic components. All set materials were subsequently sterilized with ultraviolet light for 4 h prior to testing.
2.3
Cell culture
Sorted hDPSCs were cultured in complete growth medium consisting of α-modified Eagle medium (Gibco BRL, Gaitherburg, MD, USA) supplemented with 10% fetal bovine serum (Gibco), 100 units/mL penicillin and 100 mg/mL streptomycin (Sigma–Aldrich, St. Louis, MO, USA) in 5% CO 2 at 37 °C. Cultured cells from the third to sixth passages were used for subsequent experiments. The hDPSCs were seeded at 10 5 cells/cm 2 in 24-well plates and incubated at 37 °C in a humidified 5% CO 2 atmosphere. Seeded cells were cultured in complete growth medium to ∼60% confluency prior to the commencement of osteogenic differentiation in the presence of the water-aged cements. The osteogenic medium comprised the original growth medium supplemented with 50 mg/mL ascorbic acid, 10 mmol/L β-glycerophosphate and 100 nmol/L dexamethasone (Sigma–Aldrich). Each cement disk was placed individually inside a Transwell insert with 3-μm pore size (BD Falcon, Franklin Lakes, NJ, USA) to prevent direct cell contact. The cement disks were exposed to the plated cells for 1 week, 2 weeks or 3 weeks, with the osteogenic medium renewed every 3 days. The same procedures were adopted for the virgin hDPSC control, with the exception that no material was placed inside the Transwell insert during osteogenic differentiation.
2.4
Quantitative reverse-transcription polymerase chain reaction (qRT-PCR)
Expression of osteogenic/odontogenic differentiation markers by hDPSCs was examined in sextuplicates ( N = 6). The total RNA present in the hDPSCs derived from each experimental or control group was isolated and purified using RNeasy Mini Kit (QIAGEN, Valencia, CA, USA). Quality control of the extracted RNA was performed with 2 μL samples by measuring their UV absorbance with the NanoDrop ND-1000 UV–vis spectrophotometer (Thermo Fisher Scientific Inc., Waltham, MA, USA). Quantity of extracted RNA was determined from the RNA absorbance at 260 nm; RNA purity was determined from the ratio of the absorbance (A@260 nm/A@280 nm and A@260/A@230 >1.8 and <2.0). Equal amounts of total RNA (0.1 mg/mL) were reverse-transcribed into single-stranded complementary DNA (cDNA) using a High Capacity cDNA Reverse Transcription Kit (Applied Biosystems, Foster City, CA, USA) in a 7300 real-time PCR system (Applied Biosystems). Reverse transcription was performed using 25 °C for 10 min, 37 °C for 120 min, and 85 °C for 5 min. The cDNA was stored at −20 °C until use.
Osteogenic/odontogenic target markers employed for qRT-PCR were runt-related transcription factor 2 ( RUNX2 ), osterix ( OSX ), alkaline phosphatase ( ALP ), bone sialoprotein ( BSP ), osteocalcin ( OCN ), dentin matrix protein-1 ( DMP-1 ) and dentin sialophosphoprotein ( DSPP ). Glyceraldehyde 3-phosphate dehydrogenase ( GAPDH ) was used as the housekeeping gene. A FastStart Universal SYBR Green Master Kit (Roche Applied Science, Indianapolis, IN, USA) was employed for qRT-PCR, using forward and reverse primers for the aforementioned genes (Supplementary material) in the 7300 RT-PCR system. Plates were loaded in the thermocycler and run in regular cycles. Each cycle consisted of 50 °C for 2 min, 95 °C for 10 min, 40 cycles of denaturing/annealing at 95 °C for 15 s and 60 °C for 1 min. The comparative threshold cycle method (ΔΔ C t ) was employed for relative quantification of gene expression. The expression level of each target cDNA marker was normalized to the GAPDH endogenous control. Data output was expressed as fold regulations of mRNA expression levels, given by 2 −ΔΔ C t . Gene expressions of hDPSCs exposed to the two mineralogenic stimulants were compared with those either hydraulic cement.
2.5
Western blot
Target genes that demonstrated significant fold increases in gene expressions were selected for further examination of protein expressions with Western blot. After 1 week, 2 weeks or 3 weeks of exposure to the cement disks in osteogenic medium, the cells were lysed using radioimmunoprecipitation assay buffer with a protease inhibitor cocktail (Sigma–Aldrich). Bicinchoninic acid assay was used to determine the protein concentration per specimen, using bovine serum albumin as standard. Protein denaturing and reduction was accomplished by adding 4X Laemmli sample buffer (Bio-Rad Laboratories, Hercules, CA, USA) to the specimens, after standardization of the total amount of protein to be loaded, followed by boiling the specimens at 100 °C for 5 min.
Total protein from each group (40 mg) was fractionated by sodium dodecyl sulphate-polyacrylamide gel electrophoresis (Criterion electrophoresis cell, Bio-Rad) and transferred onto a nitrocellulose membrane. The latter was blocked with 5% non-fat milk and incubated with primary antibodies (mouse anti-human) against DMP-1 (55.8 kDa; sc-73633, mouse monoclonal IgG 1 , 1:500 dilution, Santa Cruz Biotechnology, Dallas, Texas, USA), DSPP (95 kDa; sc-73632, mouse monoclonal IgG 2b , 1:500 dilution, Santa Cruz), and OCN (72 kDa; sc-365797, mouse monoclonal IgG 3 , 1:300 dilution, Santa Cruz). β-actin (43 kDa; sc-8432, mouse monoclonal, IgG 1 , 1:1000 dilution, Santa Cruz) was used as the loading control. Signals were revealed by incubation with horseradish peroxidase-conjugated secondary antibody (sc-2314, donkey anti-mouse, 1:5000 dilution, Santa Cruz) and enhanced chemiluminescence detection. Experiment was conducted in triplicates. Relative protein expression analyses were performed using the Image Studio™ Lite software (LI-COR Corp., Lincoln, NE, USA).
2.6
Alkaline phosphatase (ALP) activity
Intracellular release of ALP enzyme was measured using the Quantichrom ALP assay kit (Bioassay Systems, Hayward, CA, USA). Colorimetric determination was based on hydrolysis of p -nitrophenyl phosphate by ALP into inorganic phosphate and p -nitrophenol, a yellow-colored product. After exposure of the water-aged cement disks to hDPSCs cultured in osteogenic medium for 1 week, 2 weeks or 3 weeks, the cells were lysed with 0.2% Triton X-100 for 20 min. The cell lysate was mixed with assay buffer. Absorbance of p -nitrophenol at 405 nm was recorded every min for a maximum of 16 min using a microplate reader (Synergy HT, BioTek Instruments, Winooski, VT, USA). The level of ALP activity of the cell lysate in IU/L (μmol/L min) was calculated from the equation: (OD Sample t − OD Sample 0 ) × Reaction vol × 35.3/(OD Calibrator − OD H2O ) × Sample vol × t , where OD is the optical density at 405 nm, t is the time in min, OD Sample0 is the optical density at 0 min. Data were plotted on a graph, from which the peak value for each material was used for comparison among groups ( N = 6).
2.7
Extracellular calcium-containing deposits
Alizarin red S assay was used for qualitative and quantitative examination of the propensity of extracellular calcium-containing mineral deposits. On switching of the hDPSCs to osteogenic medium, the cells were simultaneously exposed to the cement disks for 1 week, 2 weeks or 3 weeks. The hDPSCs were then fixed in 10% formaldehyde and stained with Alizarin red S (40 mM solution; pH 4.2) for 20 min. Images of the specimens were taken for qualitative evaluation of the stained mineral deposits.
The stained calcium deposits were then dissolved in 10% acetic acid for 30 min and neutralized with 10% ammonium hydroxide prior to quantification. Absorbance of the extracts was spectrophotometrically determined at λ = 405 nm. The concentration of dye extracted from the specimens (mg/mL) was determined according to a linear regression equation derived from a calibration curve that correlated absorbance with known dye concentrations. Because one mole of dye binds to two moles of calcium to produce an Alizarin red S–calcium complex , the extent of calcium deposition was expressed as molar equivalent of calcium ( N = 6).
2.8
Attenuated total reflection-Fourier transform infrared spectroscopy (ATR-FTIR)
Extracellular mineralization by hDPSCs exposed to the water-aged cement disks was further analyzed with ATR-FTIR. Cells were cultured in osteogenic medium and exposed to the materials for 1 week, 2 weeks or 3 weeks. The hDPSCs were then fixed with 100% ethanol for 15 min, detached with a cell scraper and desiccated with anhydrous calcium sulphate. A Nicolet 6700 FT-IR spectrophotometer (Thermo Fischer Scientific) with ATR setup was used to collect infrared spectra between 4000–400 cm −1 at 4 cm −1 resolution using 32 scans ( N = 6). The acquired spectra were baseline-corrected and normalized along the collagen amide I peak (∼1635 cm −1 ). The extent of extracellular mineralization was estimated by the phosphate-to-collagen amide I ratio , using the formula: mineral = A900-1200/A1660; where A900-1200 represents the amount of phosphate in the mineralized matrix and A1660 the amount of amide I groups.
2.9
Transmission electron microscopy (TEM)
Lineage-committed hDPSCs from different groups were examined by TEM, at the end of the third week of culture in osteogenic medium, to confirm the formation of intracellular and extracellular mineral deposits. The cells were detached with 0.25% trypsin for 2 min. Pooled dislodged cells were centrifuged and fixed with 0.1 M Na cacodylate buffer (pH 7.2) containing 4% paraformaldehyde and 2% glutaraldehyde. The cell pellets were post-fixed with 1% osmium tetroxide, dehydrated in an ascending ethanol series (30–100%), transferred to propylene oxide and embedded in epoxy resin. Ninety nanometer thick sections were stained with uranyl acetate and Reynold lead citrate, and examined using a JEM-1230 TEM (JEOL, Tokyo, Japan) at 110 kV. Selected area electron diffraction was also performed on the electron-dense mineral deposits using the corresponding unstained sections.
2.10
Statistical analyses
Data sets acquired from each assay were evaluated for their normality (Shapiro–Wilk test) and equal variance assumptions (modified Levene test). If those assumptions appeared to be violated, the data sets were nonlinearly-transformed to satisfy the assumptions prior to the use of parametric statistical methods. For qRT-PCR, two-factor analysis of variance (ANOVA) was used to examine the effects of “material”, “exposure time” and the interaction of these two factors on fold regulations of individual target gene expression. Post-hoc comparisons were performed using Holm–Sidak multiple comparison procedures. Because the chance of finding one or more significant differences in testing 7 hypotheses ( i.e. , 7 genes) with the same specimens (type I error) is 0.3017 (30.17%), the Dunn–Sidak correction was used for controlling the familywise error rate in multiple comparison procedures. Accordingly, the critical value ( α ) was reduced to 0.0073 for each osteogenic marker, so that test results with P < 0.0073 were only considered significantly different from the GADPH endogenous control. Similar tests were used for analyzing the protein levels obtained from Western blot, using Dunn–Sidak correction of the α value to 0.0170 for controlling the familywise error rate in examining three hypotheses (DMP-1, DSPP and OCN expressions) with the same specimens (type I error without correction = 14.26%). For analysis of ALP enzymatic activity, calcium concentration derived from Alizarin red S staining or phosphate-to-amide I ratio derived from ATR-FTIR, two-factor ANOVA and Holm–Sidak multiple comparison procedures were used to examine the effects of “material”, “exposure time” and their interaction on the respective parameter, with statistical significance preset at α = 0.05.
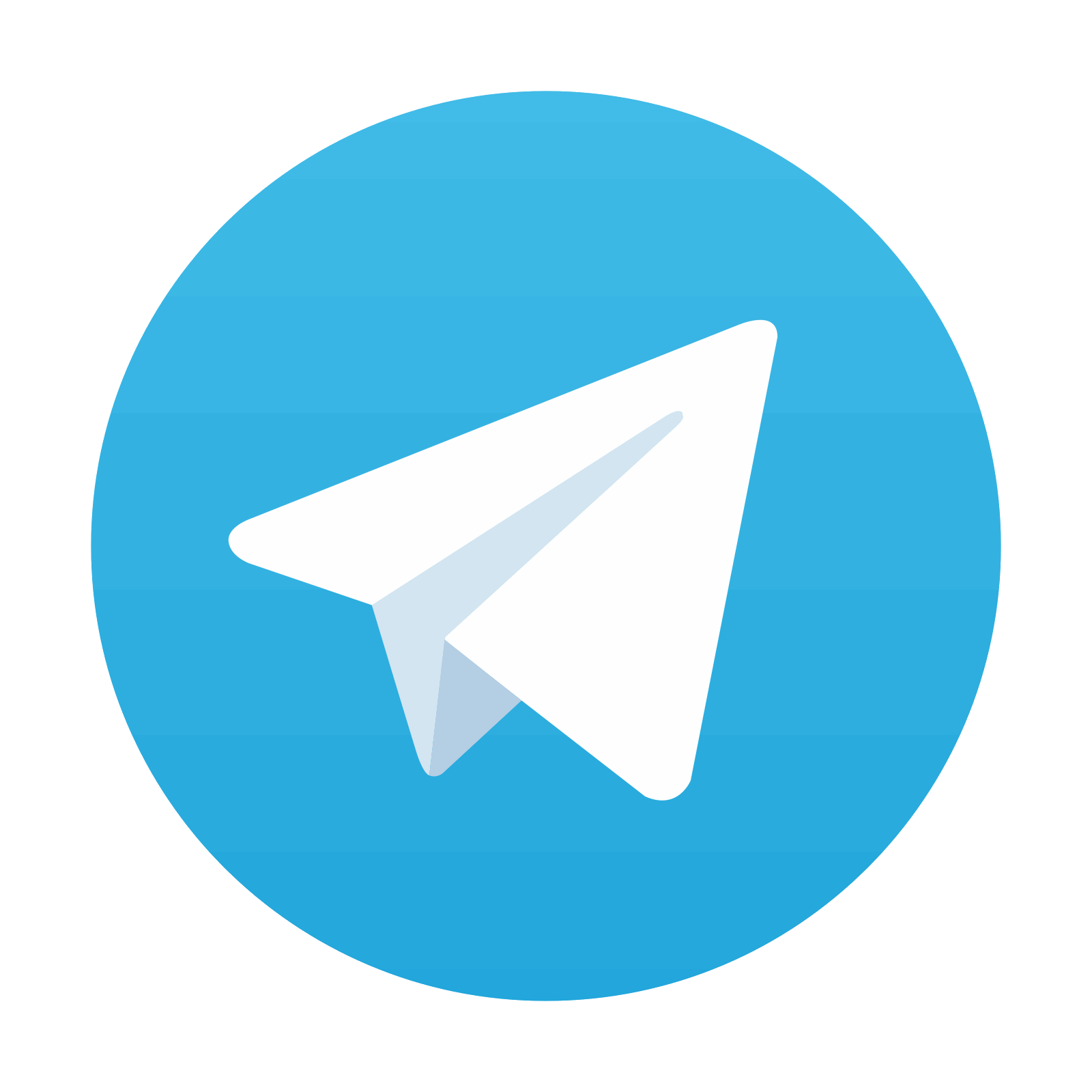
Stay updated, free dental videos. Join our Telegram channel

VIDEdental - Online dental courses
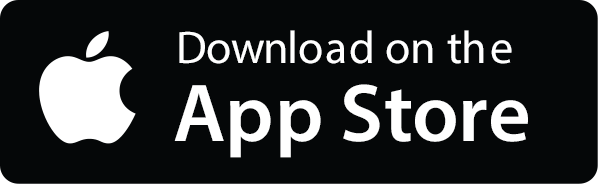
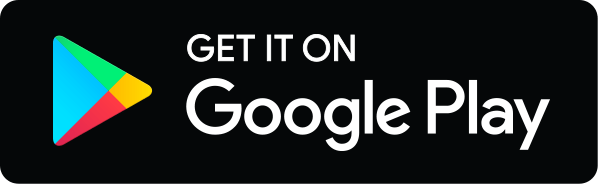