Abstract
Objective
To evaluate the effect of aging on the microstructural, mechanical, and optical properties of an experimental zirconia-toughened alumina composite with 80%Al 2 O 3 and 20%ZrO 2 (ZTA Zpex) compared to a translucent zirconia (Zpex) and Alumina.
Methods
Disc-shaped specimens were obtained by uniaxial and isostatic pressing the synthesized powders (n = 70/material). After sintering and polishing, half of the specimens underwent aging (20 h, 134 °C, 2.2 bar). Crystalline content and microstructure were evaluated using X-ray diffraction and scanning electron microscopy, respectively. Specimens underwent biaxial flexural strength testing to determine the characteristic stress, Weibull modulus, and reliability. Translucency parameter (TP) and Contrast ratio (CR) were calculated to characterize optical properties.
Results
ZTA Zpex demonstrated a compact surface with a uniform dispersion of zirconia particles within the alumina matrix, and typical alumina and zirconia crystalline content. ZTA Zpex and alumina exhibited higher CR and lower TP than Zpex. ZTA Zpex and Zpex showed significantly higher characteristic stress relative to alumina. While aging did not affect optical and mechanical properties of ZTA Zpex and alumina, Zpex demonstrated a significant increase in translucency, as well as a in characteristic stress. Alumina reliability was significantly lower than others at 300 MPa, ZTA Zpex and Zpex reliability decreased at 800 MPa, except for aged Zpex.
Significance
While aging did not affect the mechanical nor the optical properties of ZTA Zpex and alumina, it did alter both properties of Zpex. The results encourage further investigations to engineer ZTA as a framework material for long span fixed dental prostheses specially where darkened substrates, such as titanium implant abutments or endodontically treated teeth, demand masking.
1
Introduction
Yttria-stabilized tetragonal zirconia polycrystals (Y-TZP) were introduced as an alternative to metal–ceramic prostheses for esthetic dental rehabilitations and have received remarkable popularity because of its excellent properties such as improved aesthetic, biological acceptance, chemical durability and outstanding mechanical properties [ ]. Zirconia occurs naturally in three allotropic crystalline structures, which are stable according to a given temperature range: monoclinic (m; room temperature up to 1170 °C), tetragonal (t; 1170 °C until 2370 °C), and cubic (c; 2370 °C up to the melting point, 2710 °C) [ ].
The excellent mechanical properties of zirconia systems are related to a phenomenon known as transformation toughening mechanism, which can be explained by the ability of tetragonal zirconia grains to undergo a stress-mediated phase transformation to the monoclinic phase. This phenomenon is responsible for the highest fracture toughness presented by Y-TZP related to all-ceramic systems currently used in dentistry by consistently evoking the R-curve behavior, i.e. the increase of fracture toughness during crack growth [ ]. Despite the evident advantage of such mechanism, this material undergoes spontaneous tetragonal to monoclinic phase transformation at low-temperature in the presence of water, a phenomenon known as low-temperature degradation (LTD) [ ], which may eventually impair the probability of survival and mechanical properties of prosthetic rehabilitations in the long-term.
Several efforts have been made in the ceramic field to increase the resistance to LTD of zirconia ceramics by various techniques [ ]. A possible alternative to enhance the hydrothermal stability of the tetragonal phase of Y-TZP is the addition of alumina to inhibit the propagation of phase transformation into the material. Zirconia-toughened alumina (ZTA) contains zirconia particles dispersed as a second phase in an alumina matrix, bringing together the beneficial features of both materials [ ], which contributes to the stability of zirconia [ , ]. It has been established that LTD phenomenon occurs by a nucleation and growth process; where the transformation occurs on isolated grains on the surface and propagates from grain to grain. Therefore, previous literature findings have shown that alumina limits the interconnectivity of zirconia grains within the matrix, restricting phase transformation and decreasing LTD phenomenon [ , ].
The maximum zirconia fraction to limit the propagation of transformation is associated to the interconnectivity of the zirconia phase, namely the percolation threshold [ ]. Zirconia grains interconnectivity within the alumina matrix have been investigated in attempt to establish the proportion of tetragonal zirconia that may limit LTD process. Composites with a proportion between 15% and 30% of zirconia in an alumina matrix has demonstrated promising mechanical results, with a significant increase in strength after aging, which makes it a material candidate for application in areas that requires high mechanical resistance [ ]. Nonetheless, the opacity of ZTA composites may pose a challenge to dental technicians, demanding elevated skills to achieve esthetic porcelain veneering, justifying further innovations [ , ].
Therefore, in an attempt to reduce the risk for long-term complications due to the metastability of Y-TZP, this study aims to evaluate the microstructure, optical and mechanical properties of an experimental zirconia-toughened alumina (ZTA) composite fabricated with 80% Al 2 O 3 and 20% translucent Y-TZP and to compare them to their isolated counterparts (translucent Y-TZP or Al 2 O 3 ) before and after aging. The postulated null hypothesis was that the aging protocol would not affect the microstructural, mechanical, and optical properties of ZTA Zpex 80/20 composite.
2
Material and methods
2.1
Specimen preparation
A total of two hundred and ten disc-shaped specimens were fabricated from two experimental ceramics (n = 70/material), as follows: (i) ZTA Zpex 80/20: zirconia-toughened alumina (ZTA) composite (80%Al 2 O 3 /20%Zpex), (ii) Zpex: translucent Y-TZP zirconia and (iii) Alumina (α-high-purity Al 2 O 3 ).
A translucent zirconia (Y-TZP Zpex, Tosoh Corp., Japan) and high-purity alumina (α-Al2O3, Baikalox Regular CR10, Baikowski) powders were used to produce the specimens of Alumina, Zpex and ZTA Zpex 80/20 groups. For composite synthesis, the ceramic powders were mingled in ethanol suspensions, then blended and homogenized in a friction mill for 4 h with high purity alumina balls. A rotary evaporator (801, Fisaton, SP, Brazil) was used to dry the slurry, and the obtained powder was manually granulated with the aid of an alumina Mortar and Pestle set and sieved with a 0.475-mm (No. 40) sieve. The composition characteristics and the theoretical density values were calculated based on the density of alumina (3.986 g/cm 3 ) and zirconia (6.10 g/cm 3 ).
Subsequently, the ZTA Zpex 80/20, Apex, and Alumina powders were uniaxially pressed for 30 s at 1148 Kgf. A tungsten carbide cylindrical matrix (9-cm height and 5-cm diameter) with a retractable base and an internal piston for disc pressing, and a 15-mm diameter internal hole, was used to achieve 1.8-mm thickness disc-shaped green body samples. Then, they were double enveloped in a vacuum sealer and subjected to isostatic pressing (National Forge, Pennsylvania, USA) at room temperature.
The sintering of the green body samples was performed at 1600 °C for 1 h in a Zyrcomat (Vita Zahnfabrink, Bad Säckingen, Germany) furnace with a heating and cooling rate of 4 °C per minute. After sintering, both disc sides were polished by using a series of abrasive diamond disks (#220, 120, 90, 40, 25, 9, 6 and 1 μm, ALLIED High Tech Products, California, USA) in a semi-automatic polishing machine (Automet 2000, Buehler, Illinois, USA) with diamond suspensions up to 1 μm until the final dimension of 12 mm × 1 mm (diameter × thickness) had been achieved (ISO 6872:2015). The final thickness of each disc was assessed with a digital caliper (Starrett 799A-6/150 Digital Caliper, Athol, MA, USA).
2.2
Simulated LTD aging
The aging protocol was performed in autoclave (Vitale Class CD 12L, PR, Brazil) for 20 h in water vapor at 134 °C and 2.2 bar to induce tetragonal-to-monoclinic phase transformation, according to ISO13356:2015 (n = 30/group) [ , ].
2.3
X-ray diffraction (XRD)
X-ray diffraction (XRD) (X’pert Power PANalytical, Netherlands) was used to analyze the crystalline content and progression of the t -m transformation due to accelerated aging. The scanning was performed on the Bragg θ -2 θ geometry, equipped with a graphite monochromator and Cu Kα radiation ( λ = 1.5406 Å), operating at a voltage of 40 kV and a current emission of 40 mA. The XRD analysis was performed using step scan with a step size of 0.02° and between 20 and 70° (2 θ ).
The monoclinic phase content of surfaces was calculated using the Toraya and Yoshimura method. [ ]
Xm=Im-111+Im111Im-111+Im111+It101
Vm=1.311Xm/1+0.311Xm
where X m , the integrated intensity ratio, is defined by the I m (−111) and I m (111) that represent the monoclinic peaks intensity (2 θ = 28° and 2 θ = 31.2°, respectively) and I t (101) that indicates the intensity of the tetragonal peak (2 θ = 30°); V m represents the monoclinic volumetric content.
The residual stress was also determined by means of XRD analysis (Empyrean, PANalytical, The Netherlands), with the same equipment described above, where the scanning was performed from 90 to 100° setting 10 s per scan step [ ]. The evaluation of the residual stress by XRD is based on measuring the change of the interplanar spacing of crystal lattice. Thus, the measurement of the residual stress using XRD consisted of varying the beam penetration by changing the angle of incidence (Psi) to analyze the displacement of the peak as a function of depth. Hence, several spectra were obtained varying the Psi for the different ceramic materials. The peak displacement was analyzed at 95°, which was determined considering the value of 2 θ corresponding to the center of the peak. With the values of the peak position as a function of the incidence Psi angle, the planar distance (d) was calculated. Thus, correlating the variation of d of different Psi enables residual stress (σ ϕ ) calculation. This equation relates the σ ϕ as a function of the square sine of the incidence angle – Sin 2 (Psi) [ ]:
σϕ=dψ-d0d0E1+υ1sin2ψ
where ν is the Poison ratio and E the elastic modulus.
2.4
Scanning electron microscope (SEM)
To characterize the topography and microstructure of the experimental materials, high-resolution scanning electron microscopy by (FEG-SEM MIRA3 TESCAN, Czech Republic) analyses (Magellan 400L, FEI Company, Brno, Czech Republic) were performed, with a secondary electron (SE) detector under magnification of 20,000×.
2.5
Biaxial flexural strength and fractographic analysis
Thirty specimens for each group and condition (immediate and aged) were subjected to biaxial flexural strength test using a piston-on-three balls device (BFST), according to ISO 6872:2015 guidelines and demonstrated in previous studies [ ]. BFST was carried out in an ElectroPulsTM E3000 Linear-Torsion (Instron, Norwood, Massachusetts, EUA) equipment at a crosshead speed of 0.5 mm/min, until failure. Fracture load (N) data were collected and submitted to specific equations (ISO 6872:2015) to obtain the flexural strength (MPa), as follows:
σ=-0.2387.pX-Yb2
where σ is the maximum tensile stress (MPa), <SPAN role=presentation tabIndex=0 id=MathJax-Element-5-Frame class=MathJax style="POSITION: relative" data-mathml='p’>?p
p
is the total load to fracture (N), b is the thickness at fracture origin (mm), and X and Y are calculated according to:
X=1+vln(r2/r3)2+[(1-v)/2](r2/r3)2
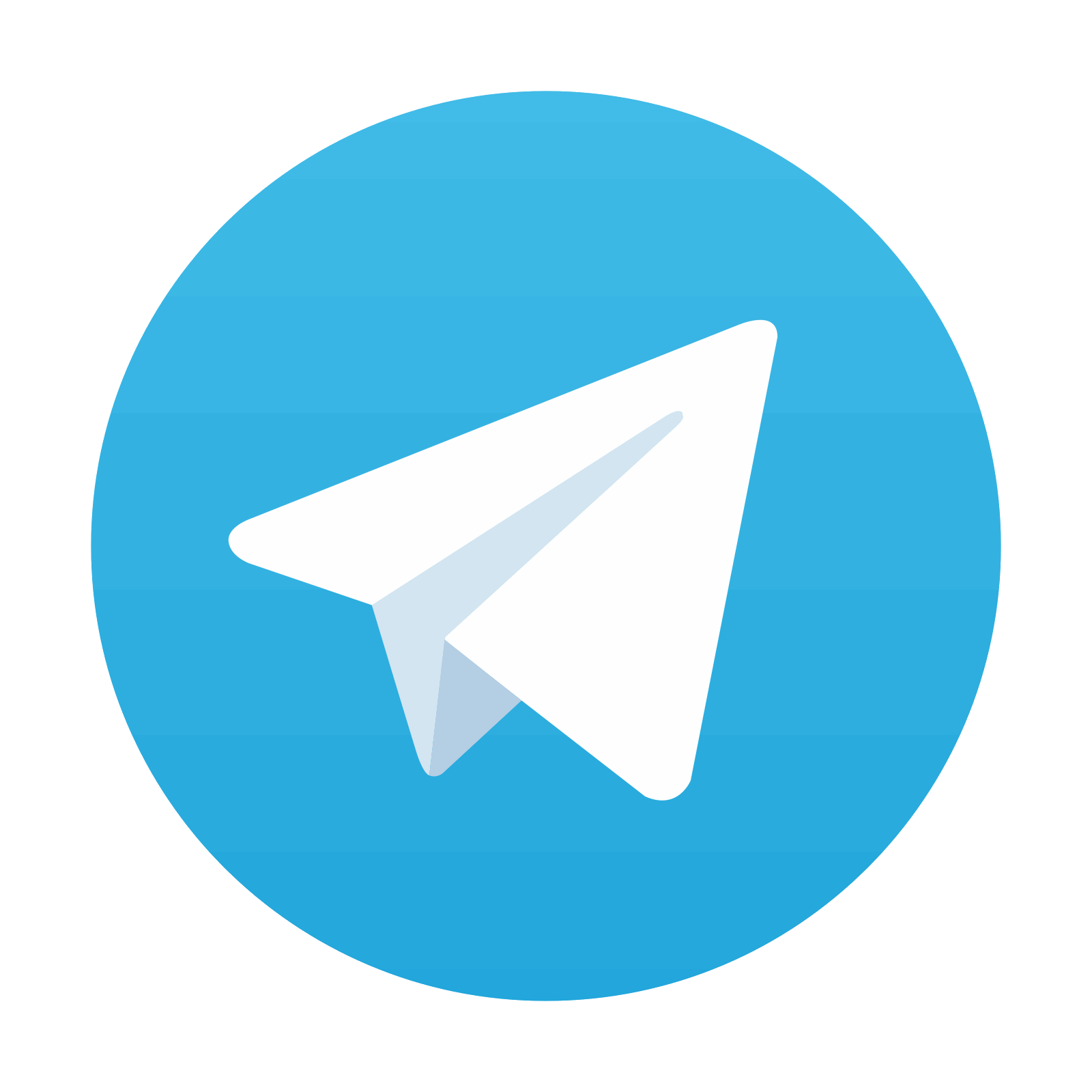
Stay updated, free dental videos. Join our Telegram channel

VIDEdental - Online dental courses
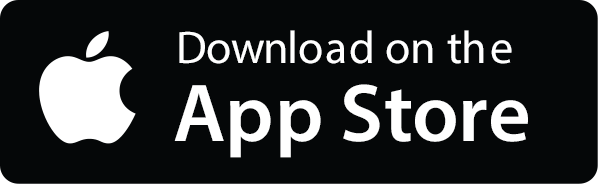
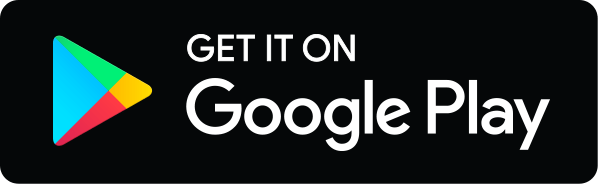
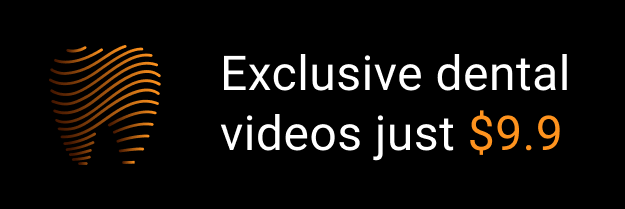