Introduction
The aim of this study was to investigate the mechanism of how micro-osteoperforations (MOPs) accelerate tooth movement. We focused on inflammation, cell proliferation, and apoptosis of periodontal ligament cells and performed immunostaining of MOPs exposed to tumor necrosis factor-alpha (TNF-α), proliferating cell nuclear antigen (PCNA), and terminal deoxynucleotidyl transferase dUTP nick end labeling (TUNEL) during experimental tooth movement.
Methods
Eleven-week-old male Wistar rats were divided into 2 groups: (1) 10 g of orthodontic force applied to the maxillary first molar (TM) and (2) force application plus 3 small perforations of the cortical plate (TM + MOPs). On days 1, 4, 7, 10, and 14 after force application, we investigated tooth movement and alveolar bone microstructure using microcomputed tomography (n = 5). We also determined the expression of TNF-α and PCNA in the pressure sides of periodontal ligaments via an immunohistochemical analysis. The expression of apoptotic cells was also determined by the TUNEL method.
Results
The tooth movement in the TM + MOPs group was significantly greater on days 4 to 14 than in the TM group. The TM + MOPs group showed statistically significant decreases in bone volume/tissue volume ratio and bone mineral density compared with the TM group. The ratios of TNF-α positive cells in the TM + MOPs group were increased on days 1, 4. 7, and 10 compared with the TM group. The ratios of PCNA positive cells in the TM + MOPs group were increased on days 1, 4, and 7 compared with the TM group, and the ratios of TUNEL positive cells in the TM + MOPs group were increased on days 1 and 7 compared with the TM group.
Conclusions
These results suggest that MOPs may accelerate tooth movement through activation of cell proliferation and apoptosis of periodontal ligament cells.
Highlights
- •
Micro-osteoperforations (MOPs) significantly accelerated OTM.
- •
MOPs decreased BMD and BV/TV ratio of the alveolar bone.
- •
The expression of TNF-α in the pressure side of PDL was increased by MOPs.
- •
The expression of PCNA and apoptosis in the PDL were also increased by MOPs.
- •
MOPs may accelerate OTM by activating inflammation and the cell cycles of the PDL.
Orthodontic treatment usually requires a long period of time, and patients experience pain and discomfort during treatment. Accelerating orthodontic tooth movement (OTM) and reducing the length of orthodontic treatment may help to reduce not only discomfort but also possible dental and periodontal complications in patients.
OTM is a multistep biologic process characterized by sequential reactions of periodontal tissue against biomechanical forces. The recruitment of osteoclast and osteoblast progenitor cells, and the balanced activation of these cells around and within the periodontal ligament (PDL) are essential for alveolar bone remodeling. Various cell-signaling pathways are activated, leading to localized bone turnover. The rate of OTM is estimated at 0.8 to 1.2 mm per month when continuous forces are applied to the teeth. Krishnan and Davidovitch reported that factors limiting the rate of OTM include bone turnover, bone density, and the degree of hyalinization of the PDL.
To modulate these biologic processes, various techniques have been developed with the goal of accelerating OTM. Pharmacologic, physical, and surgical methods are among these techniques, and we focused our attention on surgical methods. In 1959, Kole introduced corticotomy as a means of accelerating OTM. His procedure involves the reflection of full-thickness flaps to expose buccal and lingual alveolar bone, followed by interdental cuts through the cortical bone and barely penetrating the medullary bone. The subapical horizontal cuts connecting the interdental cuts were osteotomy style, penetrating the full thickness of the alveolus. In 1991, Suya reported corticotomy-assisted orthodontic treatment of 395 adult Japanese patients. Suya’s technique differed from Kole’s with the substitution of subapical horizontal cuts thinning the cortical plate instead of complete cuts through the cortical plate to the medullary bone. In 2008, Wilcko et al introduced corticotomy with alveolar grafting in a technique referred to as periodontally accelerated osteogenic orthodontics, and Kim et al introduced the less-invasive procedure of corticision. This procedure is designed to insert the surgical blade into the alveolar bone interdentally and horizontal to a depth of 8 mm without flap elevation, thus accelerating tooth movement with an enhanced turnover rate of the surrounding structures. Furthermore, in 2009, Dibart et al introduced the minimally invasive procedure of piezocision using an ultrasonic tool called a piezo-surgery knife instead of the surgical blade. These surgical methods for accelerating OTM are based on the regional acceleratory phenomenon. Frost reported that bone remodeling is accelerated and the regional bone density reduced by a noxious stimulus to the bone in a process he termed the regional acceleratory phenomenon.
Micro-osteoperforations (MOPs) are also a surgical method for accelerating OTM. Murphy introduced a method of transmucosal perforation in 2006, and Teixera et al validated the microscopic tissue response in this procedure with animal studies. Alikhani et al introduced MOPs with clinical replication in humans. MOPs are divided into 2 procedures: flap and flapless. In both procedures, alveolar bone remodeling is activated by inducing minimal surgical trauma via 3 small shallow perforations to the alveolar bone by the tooth to which the orthodontic force is being applied. The authors further showed that tooth movement was 2.3-fold faster with such perforations with flapless than with orthodontic force alone in 20 adults. They also reported little discomfort in the patients. Taken together, these reports suggest that MOPs are effective for accelerating OTM. Teixeira et al reported that perforations with a flap in the alveolar bone near the tooth accelerated OTM in animal studies. Perforations in the alveolar bone induced the expression of inflammatory cytokines and subsequently increased the osteoclast activity. Therefore, bone remodeling is enhanced after orthodontic force in this procedure. However, despite their proven efficacy, not much is known about the underlying mechanisms by which OTM is accelerated through MOPs.
OTM has been shown to be related to inflammation. Yoshimatsu et al reported that the deficiency of tumor necrosis factor-alpha (TNF-α), an inflammatory cytokine, decelerated OTM in animal studies. In addition, cell proliferation and cell death of the PDL are also known to be related to OTM.
Therefore, in this study, we focused on the relationship between accelerated OTM, inflammation, and the cell cycle in the PDL. Our null hypothesis was that accelerated OTM with MOPs would not be supported by increased apoptosis or cell proliferation. We investigated the expression of TNF-α and proliferating cell nuclear antigen (PCNA) and apoptosis in the pressure side of the PDL during experimental tooth movement with MOPs using an immunohistochemical analysis and the terminal deoxynucleotidyl transferase dUTP nick end labeling (TUNEL) method.
Materials and methods
The animal experimental protocol in this study was approved by by the ethics committee for animal experiments at the Nihon University School of Dentistry at Matsudo (approval number AP14MD008) in Japan. A total of 50 male 11-week-old Wistar rats (body weight, 300 ± 30 g; Sankyo-Lab Service, Tokyo, Japan) were used for the experiments. The animals were maintained in the animal center of the School of Dentistry in separate cages in a 12-hour light and dark environment at a constant temperature of 23°C and were given food and water ad libitum. The health status of each rat was evaluated based on daily body weight monitoring for a week before the start of the experiments. The rats were randomly assigned to 2 groups (via simple randomization); tooth movement was applied by 10 g of orthodontic force to the maxillary first molar (TM) in the control group, and the TM plus MOPs of the cortical plate (TM + MOPs). Twenty-five rats in each group were used. We calculated our sample size based on the method of Cheung et al, and the number of subjects was based on our pilot study. The experiments were performed for 14 days ( Fig 1 ).

In each group, the animals were anesthetized using an intraperitoneal injection of 3 mixed anesthetics, hydrochloric acid medetomidine (domitoru), mitazoramu, and butorphanol tartrate (betorufamu), (0.15 mg/kg body weight) for the application of orthodontic devices. Experimental tooth movement was induced using the method of Asano et al with a closed-coil spring (wire size, 0.005 in; diameter, 0.083 in; Accurate, Tokyo, Japan) ligated to the maxillary left first molar with a 0.008-in stainless steel ligature wire (Tomy International, Tokyo, Japan). The other side of the coil spring was also ligated, with holes in the maxillary incisors drilled laterally slightly higher than the gingival papilla using a round bur, applying the same ligature wire. The first molar was moved mesially by the closed-coil spring with a force of 10 g. The period of the experiment was 14 days ( Fig 2 , A ).

MOPs were induced using the method of Teixeira et al. In the TM + MOPs group, a soft tissue flap was raised around the first molar. The flaps were sealed with cyanoacrylate tissue adhesive (Vetbond; 3M, St Paul, Minn). Using a round bur and hand piece, 3 perforations were delivered to the buccal alveolar bone 5 mm mesial to the left first molar. The diameter and depth of each perforation was 0.25 ± 0.005 mm, which we measured using microcomputed tomography (μCT) ( Fig 2 , B ).
To quantify the tooth movement, the distance between the enamel on the most distal aspect of the first molar, and the mesial aspect of the second molar was measured using μCT in each animal. A quantitative imageology analysis of the amount of OTM was performed using an in-vivo μCT system (Rigaku-μCT, Tokyo, Japan). Rat molars were scanned using μCT with an x-ray source of 90 kV/88 μA at 0, 1, 4, 7, 10, and 14 days. After being deeply anesthetized with intraperitoneally injected sodium pentobarbital (35 mg/kg), each rat was placed on the object stage, and imaging was performed over a full 360° rotation with an exposure time of 17 seconds. An isotropic resolution of 30 × 30 × 30 μm voxel size was selected, which displayed the microstructure of the interdental distances between the first and second molars. The original 3-dimensional images were displayed, and the data were analyzed with the I-View software program (J. Morita, Kyoto, Japan).
Furthermore, to evaluate the differences in the bone volume/tissue volume (BV/TV) ratio and bone mineral density (BMD) between the TM and TM + MOPs groups, the region of interest was delineated to encompass the alveolar bone region surrounding the first molar, with limits at 1 mm mesial of the first molar to the mesial aspect of the second molar. Volumetric quantification of the root volume of the first molar was performed in accordance with the previously established protocol of Furfaro et al. After highlighting the root structure, reconstruction of slices produced a 3-dimensional representation of the root structure so that 3 buccal roots and 2 palatal roots could be digitally resected and their volumes analyzed individually. A global threshold of 80 was applied to all scans to extract physiologically accurate representations of the alveolar bone phase.
The experimental period was set at 14 days after tooth movement began. The animals were deeply anesthetized by 3 mixed anesthetics and then transcardially perfused with 4% paraformaldehyde in 0.1 M phosphate buffer; then the maxilla was immediately dissected and immersed in the same fixative for 18 hours at 4°C. The specimens were decalcified in 10% disodium ethylenediamine tetraacetic acid (pH 7.4) solution for 4 weeks, and the decalcified specimens were then dehydrated through a graded series of ethanol washes and embedded in paraffin using the usual methods for preparation. Each sample was sliced into 4-μm thick sections continuous in the horizontal direction and then prepared for hematoxylin and eosin, immunohistochemical staining, and the TUNEL assay. The periodontal tissues in the mesial part of the distal-buccal root of a maxillary first molar were observed. The animals in which the tooth did not move were assigned to the control group ( Fig 2 , C ).
For immunohistochemical staining, the sections were deparaffinized, and the endogenous peroxidase activities were quenched by incubation in 0.5% hydrogen peroxide in methanol for 30 minutes at room temperature. After washing in tris-buffered saline solution, the sections were incubated with TNF-α antibodies (polyclonal goat, working dilution, 1:100; R&D Systems, Minneapolis, Minn) and PCNA antibodies (PC10, mouse monoclonal, working dilution, 1:100; Cell Signal Technology, Tokyo, Japan) for 18 hours at 4°C.
TNF-α was stained using the Histofine Simple Stain MAX-Po (G), and PCNA was the Histofine Simple Stain MAX-Po (M) kit (Nichirei Biosciences, Tokyo, Japan) in accordance with the manufacturer’s protocol. The sections were rinsed with tris-buffered saline solution, and the final color reactions were performed using the 3, 3′-diaminobenzidine tetra-hydrochloride substrate reagent (DAB). The sections were then counterstained with Mayer’s hematoxylin. As immunohistochemical controls, several sections were incubated with either nonimmune rabbit IgG or 0.01 M phosphate-buffered saline solution instead of the primary antibody. Negative reactivity was observed for the controls.
Apoptosis was detected by the TUNEL assay using the TACS 2TdT-DAB In Situ Apoptosis Detection Kit (Trevigen, Gaithersburg, Md). The paraffin sections (4 μm) were incubated with proteinase K (Trevigen), diluted 1:200 at 37°C for 15 minutes, washed with deionized water, incubated with 0.5% hydrogen peroxide for 5 minutes, and then washed with deionized water again. For the positive control, the sections were incubated with DNase I at 37°C for 30 minutes. All sections were incubated with terminal deoxynucleotide transferase and Biotin-dUTP at 37°C for 1 hour (negative control sections were not incubated with the terminal deoxynucleotide transferase enzyme). After washing, the sections were incubated in strep-HRP solution at 37°C for 10 minutes. After washing, DAB was added to demarcate the apoptotic cells. The sections were counterstained with 1% methyl green and finally dehydrated and mounted.
The ratio of TNF-α, PCNA, or TUNEL positive cells was calculated as the number of positive cells in all cells observed in both the pressure and tension sides at each time period, using the following formulae.
- 1.
Ratio of TNF-α positive cells = (number of PCNA positive cells/number of all cells) × 100.
- 2.
Ratio of PCNA positive cells = (number of TUNEL positive cells/number of all cells) × 100.
- 3.
Ratio of TUNEL positive cells = (number of TUNEL positive cells/number of all cells) × 100.
Three sections were counted for both observed areas at each time period.
Statistical analysis
The Mann-Whitney U test was used to compare the medians of the groups, with values of P <0.05 considered to indicate a significant difference. The values are represented with a box-and-whisker plot.
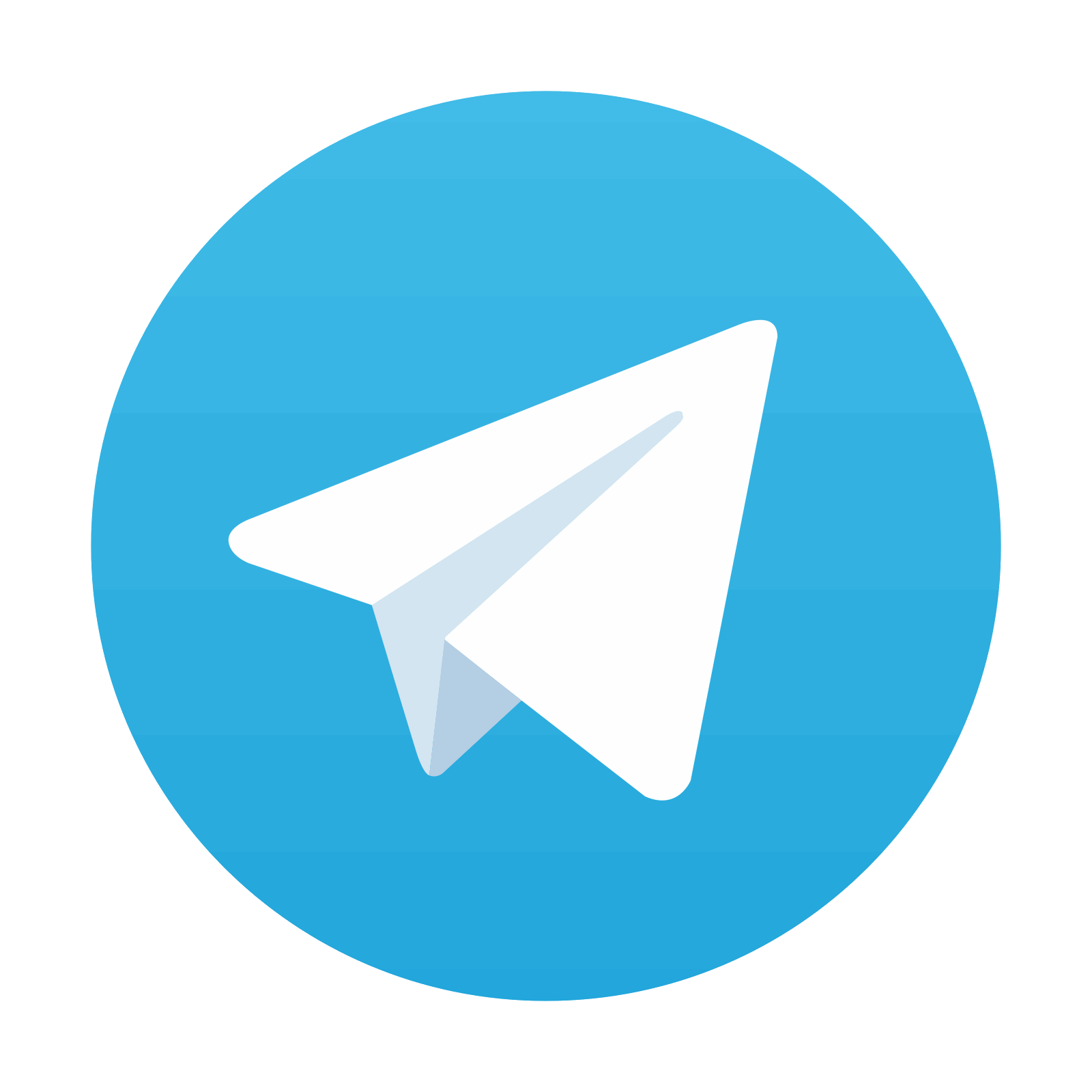
Stay updated, free dental videos. Join our Telegram channel

VIDEdental - Online dental courses
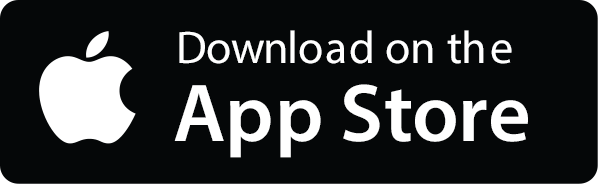
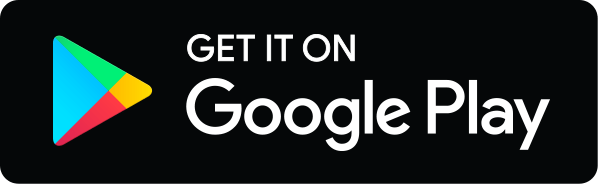