Introduction
The aims of this study were to determine the amounts of metallic ions that stainless steel, nickel-free, and titanium alloys release to a culture medium, and to evaluate the cellular viability and DNA damage of cultivated human fibroblasts with those mediums.
Methods
The metals were extracted from 10 samples (each consisting of 4 buccal tubes and 20 brackets) of the 3 orthodontic alloys that were submerged for 30 days in minimum essential medium. Next, the determination of metals was performed by using inductively coupled plasma mass spectrometry, cellular viability was assessed by using the tetrazolium reduction assay (MTT assay) (3-[4,5-dimethylthiazol-2-yl]-2, 5-diphenyltetrazolium bromide), and DNA damage was determined with the Comet assay. The metals measured in all the samples were Ti 47 , Cr 52 , Mn 55 , Co 59 , Ni 60 , Mo 92 , Fe 56 , Cu 63 , Zn 66 , As 75 , Se 78 , Cd 111 , and Pb 208 .
Results
The cellular viability of the cultured fibroblasts incubated for 7 days with minimum essential medium, with the stainless steel alloy submerged, was close to 0%. Moreover, high concentrations of titanium, chromium, manganese, cobalt, nickel, molybdenum, iron, copper, and zinc were detected. The nickel-free alloy released lower amounts of ions to the medium. The greatest damage in the cellular DNA, measured as the olive moment, was also produced by the stainless steel alloy followed by the nickel-free alloy. Conversely, the titanium alloy had an increased cellular viability and did not damage the cellular DNA, as compared with the control values.
Conclusions
The titanium brackets and tubes are the most biocompatible of the 3 alloys studied.
Glositis, metal taste, gingivitis, peeling lips, erythema multiforme, and gingival hypertrophy are frequently observed oral clinical manifestations in orthodontic treatment and are associated with corrosion products and ion release of the appliances used. These appliances remain in the mouth, a potentially corrosive environment, for an average of 2 years; in spite of their high resistance to corrosion, they release metallic ions into the tissues and fluids of patients wearing them. Heavy metals can cause damage to DNA by interacting directly with DNA or its replication, although an increased response to inflammation, the inhibition to antioxidant cellular defense, an elevated lipid peroxidation, or the inhibition of DNA repair can also contribute to mutations induced by the metals.
The most usual alloys used to manufacture stainless steel brackets are the austenites (AISI 302, 304, 316L). Contemporary stainless steels offer high resistance to corrosion because of the increased concentration of chromium of more than 12% to 13%, in the form of a transparent film of chromium oxide that is thin, uniform, continuous, resistant, and stable over the whole surface of the steel. This acts as a block to the spread of oxygen into the body of the alloy. This film, known as the passive layer, is self-repairing in the presence of oxygen in case of mechanical or chemical damage. Resistance to corrosion in these steels is also due to a low copper content and small amounts of molybdenum, which stabilizes the chromium and lend it greater resistance to pitting corrosion that is particularly frequent in brackets.
Nickel increases firmness, ductility, and resistance to cervical corrosion by competing with the chromium to form salts, allowing more chromium to be available to form the passive layer. The problem is that the union between nickel atoms and the intermetallic compounds is not strong, leading to nickel released from the alloy’s surface.
Several studies have found liberation of chromium, nickel, zinc, cobalt, and titanium from stainless steel alloys. For this reason, other alloys with greater biocompatibility, more stability in the oral environment, and less susceptibility to corrosion have been recently introduced. Nickel-free brackets (also called stainless steel with manganese or with low nickel content, less than 5%) showed greater resistance to corrosion than AISI 304 stainless steel alloy, because of the greater concentration of nickel and manganese ions released in the latter.
In most studies, orthodontic titanium alloys are made with nickel and titanium, and few with pure titanium. Biocompatibility and absence of cellular toxicity have been proved in titanium dental implants and miniplates used in rehabilitation, indicating a greater biocompatibility of titanium compared with the other alloys.
The hypothesis of this study was that the titanium alloy releases fewer metallic ions and causes less damage to the DNA of human fibroblasts that those of stainless steel and nickel-free alloys. Hence, we defined 3 objectives to (1) determine the amount of metallic ions released by 3 alloys—stainless steel, nickel-free, and titanium—to a culture medium; (2) evaluate the cellular viability of cultivated fibroblasts with the medium containing the metals released from the 3 alloys; and (3) evaluate the toxicity of those mediums on the DNA of the fibroblasts.
Material and methods
To determine the cytotoxicity of orthodontic metals on fibroblasts, we used a human fibroblast cell line 142BR (90011806; European Collection of Cell Cultures, Salisbury, Germany). The cells were cultured in petri dishes (49 cm 2 , Sarstedt, Numbrecht, Germany) with minimal essential medium (MEM) (no. M2279) supplemented with 15% fetal bovine serum, 1% antibiotic solution (10,000 μ/mL of penicillin, 10 mg/mL streptomycin, 25 μg/mL amphotericin B), 200 mM of L-glutamine, and 1% nonessential amino acids. Cell cultures were kept in an incubator (Heracel 150; Heraeus, Hanau, Germany) at 37°C, 95% humidity, and 5% carbon dioxide in the air. All products, except those indicated, were purchased from Sigma-Aldrich (St Louis, Mo).
Metals were extracted from 10 samples of 3 different metallic materials used in standard orthodontic treatments. Each sample consisted of 4 buccal tubes and 20 brackets (slot, 0.22 in) all manufactured with the same materials, submerged for 30 days in 30 mL of MEM without phenol red (no. M3024), supplemented with 0.5% fetal bovine serum, and kept in a cell incubator. The control samples contained 30 mL of supplemented MEM without metallic elements and with the same conditions as the other groups. From these 30 mL, 15 mL was used for the determination of metal ions, and the other 15 mL to perform the tetrazolium reduction assay (MTT assay). (3-[4,5-dimethylthiazol-2-yl]-2, 5-diphenyltetrazolium bromide) and the Comet assays (single cell gel electrophoresis assay), a widely-used assay to microscopically detect DNA damage at the level of a single cell.
The experimental groups were as follows.
- 1.
Control (n = 10): each sample contained 30 mL of MEM without metals.
- 2.
Stainless steel (n = 10): each sample contained 20 Ultraminitrim brackets and 4 Ortho molar tubes (both, Dentaurum, Ispringen, Germany) immersed in 30 mL of MEM. The alloy is made of 0.10% carbon, 1.0% silcon, 2.0% manganese, 17.0% chromium, 10.0% nickel, 0.50% phosphorus, 69.35% iron, and 0.05% selenium.
- 3.
Titanium (n = 10): each sample contained 20 Orthos brackets and 4 buccal molar tubes (both, Ormco, Glendora, Calif), immersed in 30 mL of MEM. The alloy is made of 99% titanium and 1% chromium.
- 4.
Nickel-free (n = 10): each sample contained 20 Minisprint brackets and 4 Flat-line molar tubes (both, Forestadent, Pforzheim, Germany) immersed in 30 mL of MEM. The alloy is made of ≤0.10% carbon, ≤1.0% silicon, 16% to 20% manganese, 16% to 20% chromium, 1.8% to 2.5% molybdenum, ≤0.3% nickel, ≤0.05% phosphorus, and ≤0.05% sulfur.
The determination of metals was performed by using inductively coupled plasma mass spectrometry (Algilent 7500ce; Agilent Technologies, Santa Clara, Calif). For that, the samples (10 mL) were acidified with 0.25 mL of 69% nitric acid (Hiperpur, Panreac, Barcelona, Spain). The metals measured in all samples were those included in the metal composition lists from the companies: Ti 47 , Cr 52 , Mn 55 , Co 59 , Ni 60 , Mo 92 , and Fe 56 . In 3 samples of each group, we analyzed also Cu 63 , Zn 66 , As 75 , Se 78 , Cd 111 , and Pb 208 . All of these metals were determined in all experimental groups, independently of the alloy compositions provided by the manufacturers. We obtained 3 determinations per sample, so the result of a sample is the average of the 3 values. The metal concentration is in the range of 1 part per billion, equivalent to 1 μg per liter. Standard monoelemental solutions for each metal (Fluka, Madrid, Spain) prepared in the MEM were used for the calibration.
For the MTT assay, cultured fibroblasts were detached from the petri dishes with a 0.25% trypsin-EDTA solution (5 minutes at 37°C) and seeded in 96-well plates at a concentration of 5000 cells per well. Counting procedures were performed with a Neubauer camera (Laboroptik, Bad Homburg, Germany) by using the trypan blue exclusion technique that stains dead cells. The first column contained only MEM (no cells) and served as the blank. The plates were kept in the cell incubator; after approximately 72 hours, they reached 90% to 100% of confluence. Next, the fetal bovine serum concentration in the MEM without phenol red was changed from 15% to 0.5% and maintained for 72 hours. Finally, we started the assay with the following distribution.
- 1.
Blank column: 200 μL of MEM without cells.
- 2.
Control column: 200 μL of MEM from the control group obtained in the previous protocol. This was our positive control, or 100% viability.
- 3.
Toxic column: 160 μL of MEM plus 40 μL of absolute ethanol.
- 4.
Stainless steel column: 200 μL of MEM from the stainless steel group.
- 5.
Titanium column: 200 μL of MEM from the titanium group.
- 6.
Nickel-free column: 200 μL of MEM from the nickel-free group.
In the toxic column, we applied ethanol, a known cellular toxin previously tested in our laboratory to cause dose-dependent toxicity. As in our previous results (data not shown), 25% ethanol also produced great damage to the fibroblasts.
The 96-well plates were maintained in the cell incubator for 7 days with the media containing metals obtained in the previous protocol. After that, the media were removed and replaced by MTT solution (5 mg/mL, in MEM) for 4 hours under the same conditions. Yellow MTT (3-[4,5-dimethylthiazol-2-yl]-2,5-diphenyltetrazolium bromide) is reduced to purple formazan in living cells. Then the excess of the MTT solution (nonreduced MTT) was eliminated from the wells. Next, 100 μL of dimethyl sulfoxide was added to dissolve the purple formazan into a colored solution, and the absorbance was quantified in a spectrophotometer (Labsytems multiskan MCC/340, Helsinki, Finland) at 570 nm with a reference wavelength of 690 nm. The percentage of survival was calculated considering the mean absorbance of the treated cells with respect to the control cells, subtracting before the mean absorbance of the blank column to both of them. Each assay was performed in triplicate.
For the Comet assay, conventional microscope slides (Menzel-Glässer, Braunschweig, Germany) were treated with 2 layers of agarose. The bottom layer was prepared by dipping the slides into 1.0% normal melting agarose (Biorad, Madrid, Spain), allowing it to solidify at 4°C for a minimum of 5 minutes. Then, the top or cell-containing layer consisted of 100 μL of a fibroblast suspension prepared in low melting-point agarose at 0.5%, containing 5000 to 10,000 fibroblasts per milliliter (15 μL of cell suspension + 85 μL agarose). After covering, the slide was kept at 4°C for 5 minutes.
Next, the second coverslip was removed, and the fibroblasts were incubated (24 hours at 37°C) with the MEM containing the different metallic compounds obtained in the first protocol. Thus, the experimental groups (n = 5) were control, toxic, stainless steel, titanium, and nickel-free. In the toxic group, the slides were additionally treated with 1000 μmol/L of hydrogen peroxide (30 minutes at 37°C). Then, all slides were rinsed with a 0.4 mol/L tris base solution (pH 7.5). Except when indicated, all incubations were performed directly on the cell layer with 100 μL of the various solutions, and the slides were kept in a moist dark chamber.
After that, the cells were subjected to lysis with 0.25% trypsin in phosphate-buffered saline solution (30 minutes at 37°C), washed with 0.4 mol/L tris base solution, and treated with proteinase K (1 mg/mL; Roche, Madrid, Spain) for 1 hour. After this, the slides were rinsed, immersed in lysis solution (2.5 mol/l sodium chloride, 0.1 mol/L EDTA, 10 mmol/L tris base; 1% Tritón X-100 (Fluka, Madrid, Spain), and 10% dimethyl sulfoxide; pH 10) for another hour at 4°C and washed again with 0.4 mol/L of tris base solution.
The Comet assay was run by using a horizontal gel electrophoresis tank (Biorad) containing freshly prepared cold (4°C) electrophoresis buffer (1 mmol/L Na2 EDTA and 10 mmol/L NaOH, pH 9) with the slides submerged side by side in the gel tray and left for 20 minutes to produce single-stranded DNA (unwinding). Later, the electrophoresis was run at 25 V and 300 mA for 20 minutes, and the slides were rinsed with tris solution.
The cells were stained with 75 μL of a 20 μg per milliliter solution of ethidium bromide (Biorad) and covered, and the comets were visualized in a fluorescence microscope (Leica, Wetzlar, Germany) by using a barrier filter of 590 nm. The images were taken with a time exposure of 2 seconds, a magnification lens of 10 times, and a resolution of 3900 × 3900. Thus, each image was formed of 12,051 megapixels, and each pixel corresponded to a detected light intensity.
We analyzed 50 randomly chosen nuclei from each slide using the free computerized image analysis system Comet Score (Tritek CometScore Freeware, version 1.5; http://autocomet.com/products_cometscore.php ). The main measure of DNA damage was the olive moment automatically obtained by the software and defined as the product of the distance between the centers of gravity of the tail (CGt) and the head (CGh) along the x-axis of the comet, and the fraction of total DNA in the tail (represented by the intensity of DNA in the tail). Olive moment = (CGt − CGh) ∗ DNA %/100.
Four slides per sample were evaluated from the experimental groups. From the repeated experiments, the averaged olive moment was calculated for each slide, each sample, and each group. Before any measurement, we calibrated geometrically the imaging software system to know the number of pixels per micrometer of our lens.
Statistical analysis
Data were expressed as means and standard deviations. The cell viability values and the olive moment passed the normality test of Kolmogorov-Smirnov. The variance in homogeneity was confirmed by the Levene test. Differences between groups were compared by 1-way analysis of variance (ANOVA); when significantly different, the means were further compared by the Tukey test. When the data did not follow a normal distribution ( Tables I and II ), we applied the ANOVA on ranks; when significantly different, medians were further compared with the Dunn test. Statistical significance was considered at a P level lower than 0.05.
Control | Stainless steel | Titanium | Nickel-free | Statistical analysis | |
---|---|---|---|---|---|
Ti 47 | 6.64 ± 2.00 | 9.03 ± 2.08 | 5.35 ± 3.96 | 3.79 ± 3.11 | 1-way ANOVA, Tukey test |
Cr 52 | 0.36 ± 0.33 | 8.97 ± 6.73 a,b | 0.06 ± 0.05 | 0.91 ± 0.28 | Kruskal-Wallis, Dunn tests |
Mn 55 | 1.02 ± 0.40 | 39.40 ± 17.85 a,b | 0.44 ± 0.28 | 43.99 ± 21.29 a,b | Kruskal-Wallis, Dunn tests |
Co 59 | 0.00 ± 0.00 | 1.38 ± 1.25 a,b,d | 0.03 ± 0.01 | 0.00 ± 0.00 | Kruskal-Wallis, Dunn tests |
Ni 60 | 0.00 ± 0.00 | 416.97 ± 133.54 a,b,d | 0.00 ± 0.00 | 5.79 ± 2.07 | Kruskal-Wallis, Dunn tests |
Mo 92 | 0.80 ± 0.27 | 3.11 ± 1.47 a,b,d | 0.11 ± 0.07 | 0.60 ± 0.21 | Kruskal-Wallis, Dunn tests |
Fe 56 | 42.33 ± 27.06 | 520.10 ± 210.10 a,b | 49.67 ± 29.29 | 179.49 ± 99.16 | Kruskal-Wallis, Dunn tests |
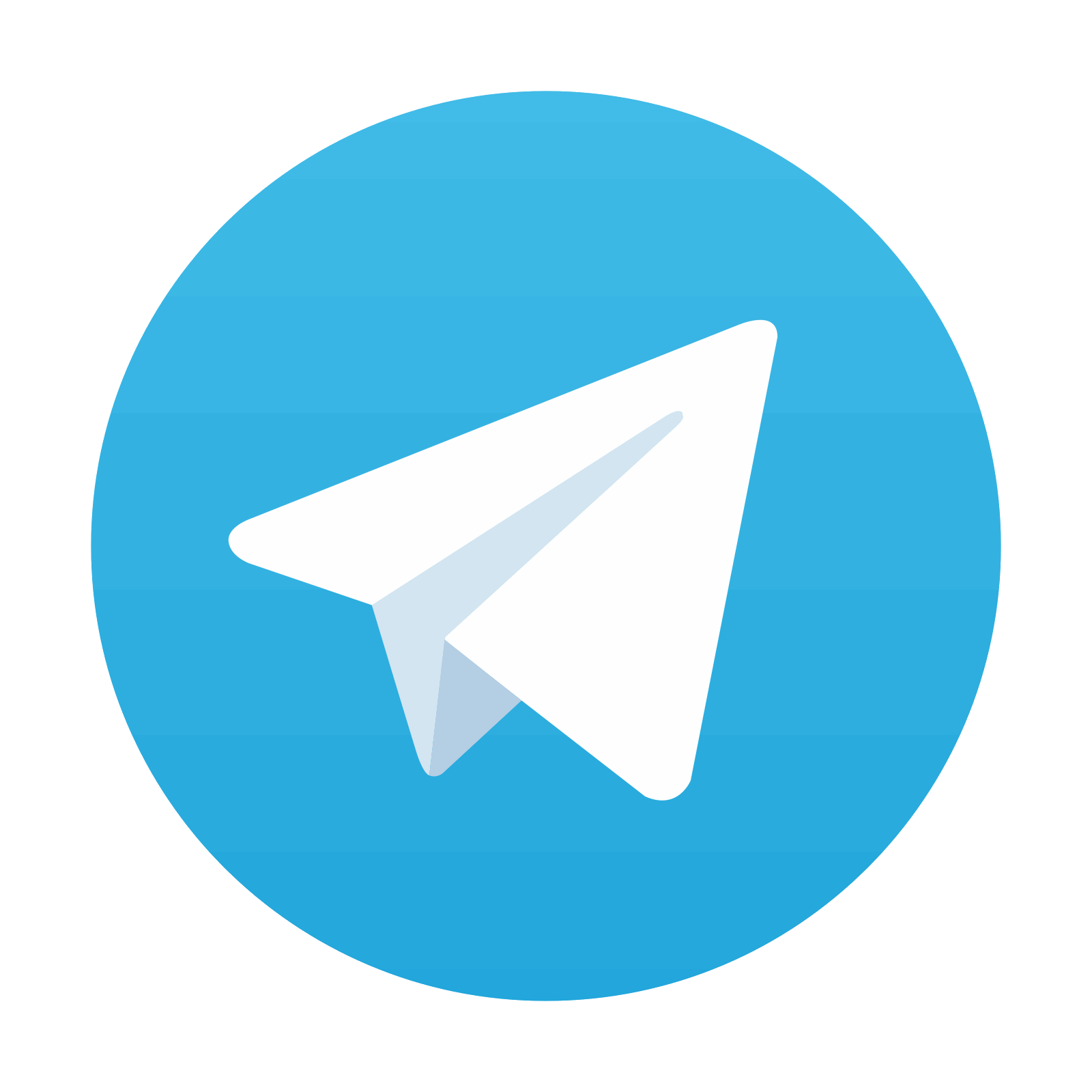
Stay updated, free dental videos. Join our Telegram channel

VIDEdental - Online dental courses
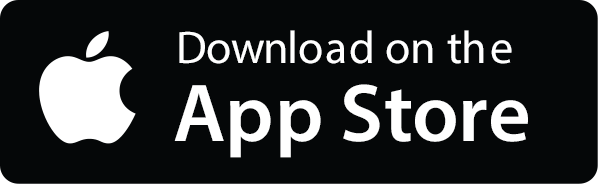
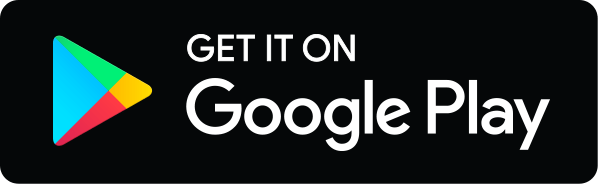
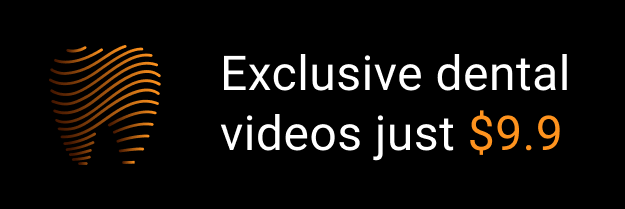