Abstract
Objective
Composite resins do not adhere well to dental alloys. This weak bond can result in failure at the composite-metal interface in fixed dental prostheses and orthodontic brackets. The aim of this study was to develop a new adhesive, based on diazonium chemistry, to facilitate chemical bonding between dental alloys and composite resin.
Methods
Samples of two types of dental alloys, stainless steel and cobalt chromium were primed with a diazonium layer in order to create a surface coating favorable for composite adhesion. Untreated metal samples served as controls. The surface chemical composition of the treated and untreated samples was analyzed by X-ray photoelectron spectroscopy (XPS) and the tensile strength of the bond with composite resin was measured. The diazonium adhesive was also tested for shear bond strength between stainless steel orthodontic brackets and teeth.
Results
XPS confirmed the presence of a diazonium coating on the treated metals. The coating significantly increased the tensile and shear bond strengths by three and four folds respectively between the treated alloys and composite resin. Conclusion: diazonium chemistry can be used to develop composite adhesives for dental alloys.
Significance
Diazonium adhesion can effectively achieve a strong chemical bond between dental alloys and composite resin. This technology can be used for composite repair of fractured crowns, for crown cementation with resin based cements, and for bracket bonding.
1
Introduction
Composite resins are widely used in modern dental practice due to their ability to bind to acid etched tooth structure, and their good handling, mechanical, and esthetic properties. Composites are used in a variety of dental applications such as temporary and permanent restorations, cementation and repair of indirect restorations as well as bonding of orthodontic brackets . However, the weak adherence of dental composites to dental alloys frequently leads to clinical problems such as failures at the resin-metal interface in fixed dental prostheses and de-bonding of orthodontic bracket .
Porcelain fused to alloys have been used for many years for the fabrication of esthetic fixed prostheses. These prostheses present good clinical performance with 88.7% survival rates over ten years for metal-ceramic crowns and 80.2% for fixed partial dentures . The most common cause for replacement of these restorations is dental caries followed by delamination of veneering porcelain . Over time delamination of veneering porcelain can occur in 3–13% of the restorations . This is usually caused by technical mistakes during their fabrication as well as difference between metals and ceramics in terms of modulus of elasticity, and coefficient of thermal expansion, moisture in the oral cavity, and mechanical fatigue of the ceramic itself . The life of defective fixed prostheses could be prolonged by repairing the porcelain veneer with resin based composites , using surface conditioning methods and coupling agents to bond the resin composites to the metal substrates . Surface conditioning can be done with hydrofluoric acid etching and air particle abrasion whereas the coupling agents are usually based on silane adhesives alone or in combination with acidic or sulfur-containing adhesive monomers . However, these procedures involve a number of drawbacks such as the hazardous intraoral use of hydrofluoric acid and air born abrasion, as well the special equipment needed for silica coating and the limited long-term performance .
In orthodontic treatments, metal brackets are usually made of stainless steel, and are bonded to teeth using commercially available resin based dental adhesives . These bonding systems adhere well to etched tooth enamel but very poorly to metallic brackets . Typically, bracket bases are designed with surface patterns that favor micromechanical interlocking to create mechanical retention at the resin-base interface . However, mechanical failure often occurs at the bracket-composite interface resulting in bracket de-bonding . Bracket de-bonding prolongs treatment duration and chair time, increasing treatment cost and causing inconvenience to the patient and the dentist . To compensate for the lack of adhesion between metallic brackets and composite , the majority of research has been focused on modifications to the bracket base surface pattern in order to increase micromechanical interlocking, which has only achieved minor improvements .
A series of metal adhesives have been explored to improve the shear bond strength between brackets and the tooth or ceramic crown and between composite repairs and porcelain fused to metal (PFM) crowns, this include among others silane coupling agents, and bifunctional acidic monomers such as 10-methacryloxy ethyl diphosphate monomer (MDP) .
Silane coupling agents have been used to chemically bond resin composites to conditioned dental alloys, and ceramics. The silanol groups in silane coupling agents react with the OH group present on the oxidized metal surface, consequently bonding is to metal oxides and not to the metal itself . Silane coupling agents have been improved over the years in an attempt to optimize their properties while rendering them durable and less clinically demanding. Nowadays silanes are supplied in a pre-hydrolyzed form in ethanol/water solutions to facilitate their clinical use . However, Silane undergoes hydrolysis and this limits its shelf life and makes its long term efficiency doubtful , as its bond strength is sensitive to humidity, and decreases over time .
HEMA (2-hydroxy ethyl methacrylate) and adhesive acidic monomers that have a carboxylic group such as hydrolyzed 4-META(4-methacryloxyethyl trimellitic anhydride) or the phosphonic acid group [R–P( O)(OH) 2 ] with or without sulfur groups, are also capable of bonding to non-precious metal alloys resulting in a strong and durable bonding to various substrate materials. These monomers can also interact chemically with the metal oxide in base metal alloys , and they have superior hydrolytic stability, but they exhibit poor adhesion to precious metals and alloys and they require surface preparation of the substrate prior to their application .
Given the above-mentioned limitations of current bonding agents there is a need for the development of chemical adhesives that could increase the metal-composite bond strength and eliminate the difficulties encountered with the existing bonding strategies .
Aryldiazonium salts have been the subject of much recent research due to their ability to react with a wide range of surfaces including metals . Aryldiazonium salts are easy to prepare, and are rapidly reduced to stable radicals that allow strong covalent bonding . Diazonium ions can be produced from aromatic amines and grafted onto almost any surface using chemical reducing agents in acidic solutions . The reducing agents activate the aryldiazonium salts and form aryl radicals that covalently bind to the surface of interest . If an extra amino group is present on the aryldiazonium precursor, this group can be further activated and used to bind a second layer onto the original surface . The chemical bond formed between aryl diazonium salts and the different substrates is stable at temperatures as high as 200 °C, which is much higher than the maximum temperature reached in the oral cavity . It is very resistant to solvents and ultrasonication , and is electrochemically stable. Mechanically, the adhesive is also stable, it can withstand scratching, and just as other coupling agents, it is also capable of improving the bond strength between substrates .
The objective of this study was to develop a new surface treatment, based on diazonium chemistry, to facilitate chemical bonding between dental alloys and composite resins. This new simple and inexpensive bonding method would allow easy intraoral repair of fractured porcelain- fused-to-metal prosthesis and prevent de-bonding of orthodontic brackets.
2
Materials and methods
2.1
Materials
2.1.1
Treatment materials
P -Phenylenediamine (PPD), sodium nitrite (NaNO 2 ), sodium dodecyl sulfate (SDS), benzoyl peroxide (BP), bisphenol A-glycidyl methacrylate (Bis-GMA), hypophosphorous acid (H 3 PO 2 ), and hydrochloric acid (HCl) were purchased from Sigma Aldrich (St. Louis, MO) and were used without any further purification. Concentrated hydrochloric acid (HCl) was diluted in distilled water to a concentration of 0.5 M.
2.1.2
Mirror polished samples
Surface roughness can have a strong impact on adhesion accordingly, standardized mirror polished metallic specimens were prepared to minimize bias upon comparison between surface treatments. Two types of alloys were assessed in this study to gain an insight onto diazonium surface treatments. Cobalt chromium due to its wide spread use in dental prosthesis, and stainless steel due to its extensive use in orthodontics and prosthetic appliances. Cylindrical shaped cobalt chromium samples (CoCr alloy super 6; 66% Co, 28% Cr, 6% Mo, and 0.5% other elements) with average dimensions of 7.86 mm diameter and 19.05 mm height were obtained from US Dental Depot (Florida, USA) and used as is for the mechanical test. For elemental release and X-ray photoelectron spectroscopy analyses samples were cut into smaller sections (7.86 mm diameter and 4.8 mm height). Stainless steel samples (detailed elemental composition in Supplementary file) were obtained from McMaster-Carr (Cleveland, OH). They were cut into smaller sections (12.7 × 6.4 × 6.4 mm) using an abrasive cutter (Delta Abrasi Met, Buehler, Whitby, ON). All metal samples were polished to obtain a mirror finish. They were first ground with a water-cooled trimmer using 240–600 grit silicon carbide paper (Paper-c wt, AA Abrasive, Philadelphia, PA). Then they were polished using a polishing wheel (LapoPol-5, Struers, Rodovre, Denmark) and two types of polishing clothes (15–0.02 um; TexMet C, 1–0.02 um; ChemoMet) with colloidal silica suspension (<0.06 um; Master Met; Buehler, USA). All samples were then cleaned in an ultrasonic bath (FS20D Ultrasonic, Fisher Scientific, Montreal, Canada) with distilled water, then ethanol, and finally acetone for 5 min in each solution at room temperature .
2.1.3
Brackets
To assess the effect of diazonium treatment on composite-bracket adhesion maxillary central incisor stainless steel orthodontic brackets were used in this study (Unitek Miniature Twin Bracket Roth 3M Unitek, Monrovia, CA) ( Fig. 2 b).
2.1.4
Resin based adhesive
In order to mimic the procedures most commonly adopted clinically, two types of resin adhesive were used to verify the effect of the new coupling agent. A chemical-cured resin cement (Concise™ Orthodontic Chemical Cure Adhesive 3M; London, ON), was used on the mirror polished samples, and a light-cured adhesive (Transbond™ XT Light Cure Adhesive; 3M, London, ON) was used on the orthodontic brackets. Table 1 shows the chemical composition of both resin cements. For better assessment of the direct bond strength provided by the new diazonium coupling agent, both cements used plain composite resins (inorganic matrix and filler) free of adhesive monomers or metal primers that could enhance bonding.
Material | Monomer type | % | Initiator | Filler type | Filler content |
---|---|---|---|---|---|
Light cure adhesive | Bis-GMA | 10–20% | None | Silica | <2% |
Bis-HDMA | 5–10% | Quartz | 70–80% | ||
Chemical cure adhesive | |||||
Paste 1 | Bis-GMA | 10–20% | None | Silica | <2% |
TEGDMA | 1–10% | Quartz | 75–85% | ||
Paste 2 | Bis-GMA | 10–20% | BP | Silica | <2% |
TEGDMA | 1–10% | <0.5% | Quartz | 70–80% |
2.2
Methods
2.2.1
Surface treatment method
All metal samples were divided into three test groups: controls, which did not undergo any treatment; primed, which were treated with the primer solution ( Fig. 1 a–c); and Bis-GMA coated, which were treated with both the primer and the Bis-GMA adhesive solution ( Fig. 1 a–f). Both solutions were prepared at room temperature in acidic deionized water at pH ≤2, because diazonium cations are stable at pH ≤2.5 . The first solution (primer) was prepared by dissolving PPD (0.054 g; 0.05 M) and NaNO 2 (0.034 g; 0.05 M) in a beaker containing 10 ml of 0.5 M HCl ( Fig. 1 a). The samples were introduced into the solution then H 3 PO 2 (0.66 ml) was added to the primer solution as a reducing agent to form aryl radicals ( Fig. 1 b). They were left to react for 15 min before ultrasonicating them in distilled water and acetone for 5 min each. This treatment process created a film of diazonium on the metal samples ( Fig. 1 c) . The metal samples after this step are referred to as “primed metal samples”. For the Bis-GMA treated group, the samples were first introduced into the primer solution as mentioned above ( Fig. 1 a–c), and then they were introduced into the adhesive solution. This solution was prepared by dissolving NaNO 2 (0.034 g; 0.05 M) in a beaker containing 10 ml of HCl (0.5 M). The primed metal samples were introduced into the solution then 0.66 ml of H 3 PO 2 ( Fig. 1 d) was added. The Bis-GMA monomer (2.3 g/2.0 ml of ethanol) was then added into the solution with sodium dodecyl sulfate (SDS 0.026 g; 9 × 10 −3 M) which functioned as a surfactant to emulsify Bis-GMA . After 10 min of sonication, an initiator (benzoyl peroxide (BP), 40 mg/ml) was added to accelerate the polymerization reaction. The resulting solution was further sonicated for 15 min ( Fig. 1 e). The reaction was allowed to continue in the fumehood for 45 min. Finally, the samples were ultrasonicated in distilled water followed by acetone for 5 min each to discard any ungrafted material. After this step metal samples are referred to as “Bis-GMA coated”.
2.2.2
X-ray photoelectron spectroscopy (XPS)
A monochromatic X-ray photoelectron spectrometer K Alpha (Thermo Fischer Scientific Inc., East Grinstead, UK) was used to determine the surface chemical composition of all metal alloy samples.
For XPS analysis, metal samples (both mirror-polished and brackets) were divided into three groups: control, primed and Bis-GMA-coated. Each group consisted of three specimens, and each specimen was read at three different points. Survey scans were obtained over the range of 0–1350 eV with a pass energy of 200 eV at a step of 1.0 eV, while high resolution scans for carbon were collected with a pass energy of 50 eV at a step of 0.1 eV. Data analysis and peak fitting were performed using Avantage (5.41v, Thermo Fischer Scientific Inc., East Grinstead, UK) chemical surface analysis software.
The high resolution XPS C1s peak was deconvoluted into four components for the control groups and five components for the primed and Bis-GMA-treated groups. The peak at 284.8 eV corresponds to both aliphatic and aromatic hydrocarbons (C C, C H), that refer to adventitious hydrocarbon due to unavoidable surface contamination. The peak at 286.3 eV refers to carbon single bonded to oxygen or nitrogen (C N/C O) that can be related to adventitious carbon in the control groups and the confirmation of the presence of PPD and Bis-GMA in the primed and adhesive-treated groups. The component at 288.6 eV corresponds to carbon double bonded to oxygen (C O). The component at 291.5 eV is related to shake up satellite peaks that confirm the presence of aromatic carbon on the surface. The component at 283.3 eV refers to carbide bonds (metal–carbon bond) that can be related to adventitious carbon in control groups and the coating in the primed and adhesive-treated groups.
2.2.3
Mechanical tests
2.2.3.1
Mechanical test for mirror polished samples
The mechanical performance of the bonding between mirror-polished samples and composite resin was assessed using tensile test for three groups (control, primed and Bis-GMA- treated). A hole with a diameter of 2.5 mm was drilled in both types of samples as shown in Fig. 2 a to provide a grip for the tensile bond test. Each group consisted of ten samples. The chemically cured resin cement was mixed and applied as a thin film to one of the samples and the other sample (of the same type) was then attached to it ( Fig. 2 a). Excess cement was removed using a plastic spatula and the bonded samples were put aside to allow the resin to set. After 24 h, the samples were pulled apart using a universal testing machine (Instron, 5569, Grove City, PA) set at a constant speed of 10 mm/min ( Fig. 2 b).
2.2.3.2
Mechanical test for the orthodontic brackets
A light-cured Bis-GMA composite adhesive was used in this section to simulate the clinical procedure of bracket bonding. The mechanical performance of the bond between brackets and composite was assessed using shear strength test ( Fig. 2 c) for the three test groups (control, primed and Bis-GMA adhesive treated).
2.2.3.3
Teeth and mold preparation
Following The McGill University Health Center Ethical Committee approval and patient consent, extracted compromised human molars with intact enamel were collected and immersed in 10% formalin solution (BF-FORM, Fisher Scientific, Canada) for 1 week. The teeth were then cleaned with distilled water in an ultrasonic bath (FS20D Ultrasonic, Fisher Scientific, Canada) for sixty minutes at 25 °C and polished for one minute with a low-speed dental hand piece (M5Pa, KAB-Dental, USA) using SiC cups (Pro-Cup, Kerr, Italy) and low abrasive dental prophylaxis pumice (CPRTM, ICCARE, USA). The teeth were then rinsed in an ultrasonic bath before storage in Eppendorf tubes with 10% formalin solution . Post-storage, the teeth were cleaned with water and air-dried. In preparation for shear strength testing, each tooth was partially embedded in a mold of Plaster of Paris as per Fig. 2 c.
In order to evaluate the shear strength of the bond between teeth and composite bonded brackets, each tooth surface was first etched with phosphoric acid gel (EZ Etch; Dentsply, York, PA) for 30 s, rinsed with distilled water and air-dried. Transbond adhesive primer (3M, London ON) was then applied to the tooth surface and light cured. The light cure resin cement was then applied to the base of a bracket that was then positioned on the tooth surface. The composite resin was then light cured using ESPE Premier, Norristown, PA light cure unit (1000 mW cm −2 ) from different angles to ensure complete polymerization. After 24 h of storage (both dry and water storage), a load, parallel to the long axis of the tooth was applied using the universal testing machine (Instron, 5569, Grove City, PA) set at a constant speed of 10 mm/min. This generated a shear force at the tooth-bracket interface ( Fig. 2 c).
2.2.4
Analysis of released elements
Mirror polished samples were divided into three groups (control, primed and Bis-GMA-treated) as discussed above. Each sample was incubated in 20 ml of Phosphate Buffered Solution (PBS) at 37 °C in an air atmosphere containing 5% CO 2 . A blank sample was also prepared to assess the elements present in the background of the analyzed solutions. The media was collected after 7 days of incubation for further analysis using inductively coupled plasma atomic emission spectrometry (ICP-AES) and total organic carbon (TOC) analysis.
Elemental composition of the collected media was analyzed using ICP-AES (PerkinElmer City, Woodbridge, ON.) by taking 4.5 ml of each sample incubation solution and digesting it with 0.2 ml 4% nitric acid, which was then diluted to 5 ml using deionized water and then analyzed in ICP-AES. The incubation media of cobalt chromium alloy was analyzed for cobalt, chromium, and molybdenum and the stainless steel samples were tested for iron, chromium, and nickel.
Total organic carbon measurements were performed in order to detect whether there was a difference in the release of organic carbon from the control and treated alloy extracts. The remaining 15 ml from each of the previously used solutions was used in this analysis. TOC analyzer (Shimadzu TOC-V CSH/CSN, Columbia, USA) was used to detect total organic carbon and total nitrogen released from the samples.
2.2.5
Statistical analysis
The statistical analyses were performed using SPSS software (version 22; SPSS Inc., IBM corporation, Chicago IL) and Origin (version 9; Origin Laboratory, Northampton, MA, USA). All data was presented as average and standard deviation. Normality test was carried out for all acquired data. All data representing a normal distribution was analyzed using One Way ANOVA tests, otherwise non-parametric Kruskal–Wallis test was used. Statistical significance was set at p < 0.05.
2
Materials and methods
2.1
Materials
2.1.1
Treatment materials
P -Phenylenediamine (PPD), sodium nitrite (NaNO 2 ), sodium dodecyl sulfate (SDS), benzoyl peroxide (BP), bisphenol A-glycidyl methacrylate (Bis-GMA), hypophosphorous acid (H 3 PO 2 ), and hydrochloric acid (HCl) were purchased from Sigma Aldrich (St. Louis, MO) and were used without any further purification. Concentrated hydrochloric acid (HCl) was diluted in distilled water to a concentration of 0.5 M.
2.1.2
Mirror polished samples
Surface roughness can have a strong impact on adhesion accordingly, standardized mirror polished metallic specimens were prepared to minimize bias upon comparison between surface treatments. Two types of alloys were assessed in this study to gain an insight onto diazonium surface treatments. Cobalt chromium due to its wide spread use in dental prosthesis, and stainless steel due to its extensive use in orthodontics and prosthetic appliances. Cylindrical shaped cobalt chromium samples (CoCr alloy super 6; 66% Co, 28% Cr, 6% Mo, and 0.5% other elements) with average dimensions of 7.86 mm diameter and 19.05 mm height were obtained from US Dental Depot (Florida, USA) and used as is for the mechanical test. For elemental release and X-ray photoelectron spectroscopy analyses samples were cut into smaller sections (7.86 mm diameter and 4.8 mm height). Stainless steel samples (detailed elemental composition in Supplementary file) were obtained from McMaster-Carr (Cleveland, OH). They were cut into smaller sections (12.7 × 6.4 × 6.4 mm) using an abrasive cutter (Delta Abrasi Met, Buehler, Whitby, ON). All metal samples were polished to obtain a mirror finish. They were first ground with a water-cooled trimmer using 240–600 grit silicon carbide paper (Paper-c wt, AA Abrasive, Philadelphia, PA). Then they were polished using a polishing wheel (LapoPol-5, Struers, Rodovre, Denmark) and two types of polishing clothes (15–0.02 um; TexMet C, 1–0.02 um; ChemoMet) with colloidal silica suspension (<0.06 um; Master Met; Buehler, USA). All samples were then cleaned in an ultrasonic bath (FS20D Ultrasonic, Fisher Scientific, Montreal, Canada) with distilled water, then ethanol, and finally acetone for 5 min in each solution at room temperature .
2.1.3
Brackets
To assess the effect of diazonium treatment on composite-bracket adhesion maxillary central incisor stainless steel orthodontic brackets were used in this study (Unitek Miniature Twin Bracket Roth 3M Unitek, Monrovia, CA) ( Fig. 2 b).
2.1.4
Resin based adhesive
In order to mimic the procedures most commonly adopted clinically, two types of resin adhesive were used to verify the effect of the new coupling agent. A chemical-cured resin cement (Concise™ Orthodontic Chemical Cure Adhesive 3M; London, ON), was used on the mirror polished samples, and a light-cured adhesive (Transbond™ XT Light Cure Adhesive; 3M, London, ON) was used on the orthodontic brackets. Table 1 shows the chemical composition of both resin cements. For better assessment of the direct bond strength provided by the new diazonium coupling agent, both cements used plain composite resins (inorganic matrix and filler) free of adhesive monomers or metal primers that could enhance bonding.
Material | Monomer type | % | Initiator | Filler type | Filler content |
---|---|---|---|---|---|
Light cure adhesive | Bis-GMA | 10–20% | None | Silica | <2% |
Bis-HDMA | 5–10% | Quartz | 70–80% | ||
Chemical cure adhesive | |||||
Paste 1 | Bis-GMA | 10–20% | None | Silica | <2% |
TEGDMA | 1–10% | Quartz | 75–85% | ||
Paste 2 | Bis-GMA | 10–20% | BP | Silica | <2% |
TEGDMA | 1–10% | <0.5% | Quartz | 70–80% |
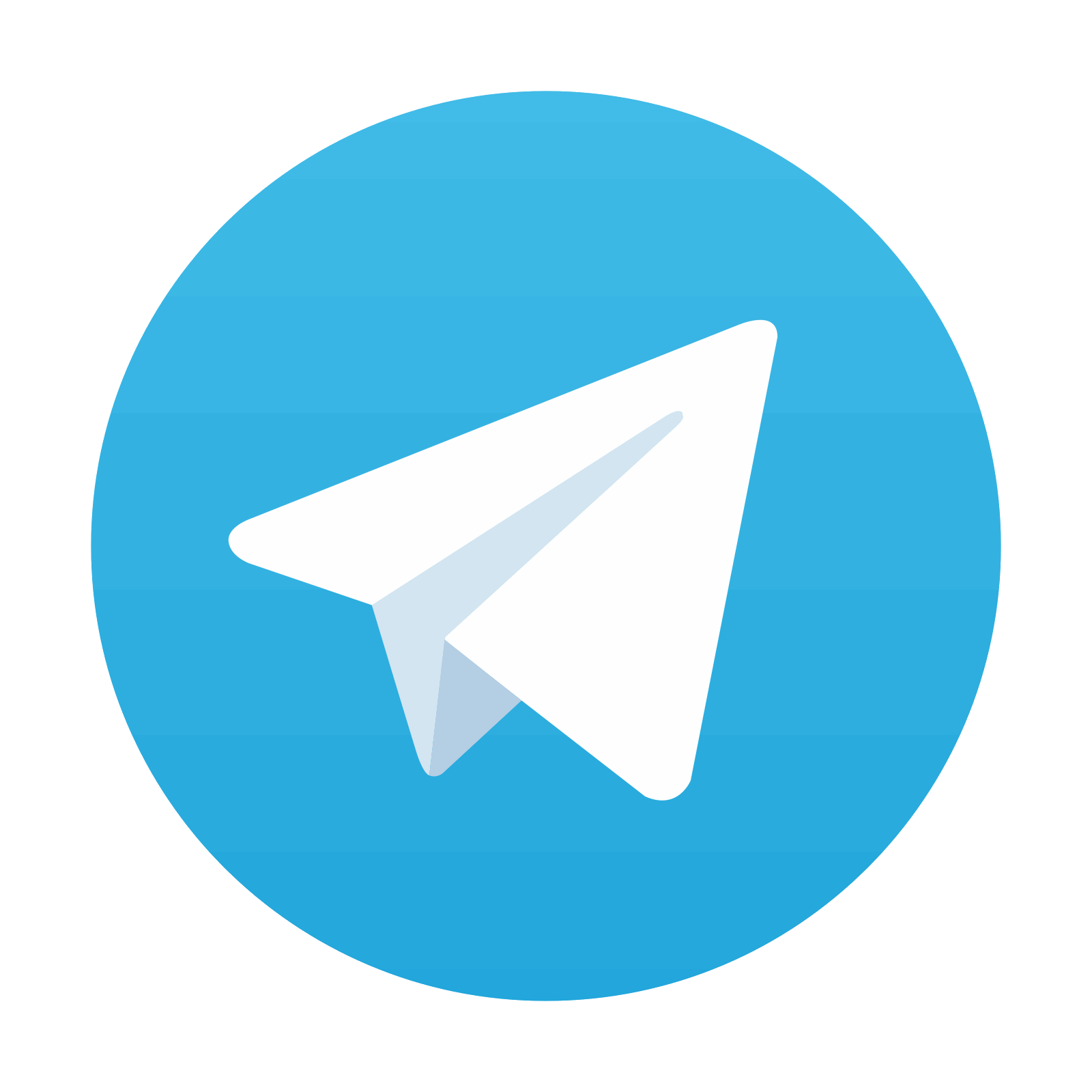
Stay updated, free dental videos. Join our Telegram channel

VIDEdental - Online dental courses
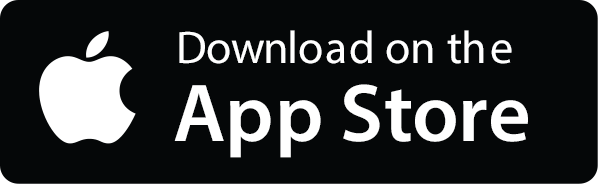
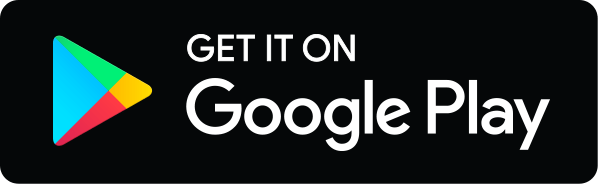
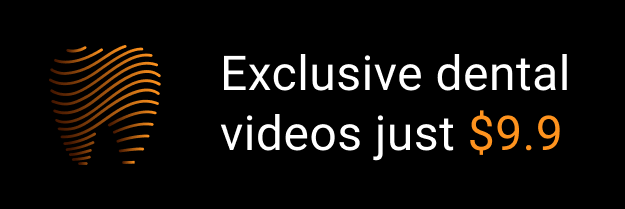