Introduction
Palatal expansion can potentially affect alveolar bone and circummaxillary sutures. In this study, we characterized their mechanical strain during acute expansion.
Methods
Eight 3- and 6-month-old fresh pig heads received acute palatal expansion with hyrax expanders. Strain gauges were used to measure strain at the buccal alveolar bone of anchor and adjacent nonanchor teeth, and at maxillary-premaxillary, maxillary-zygomatic, and zygomatic-temporal sutures during expansion. Intermolar width changes were measured from dental casts.
Results
Intermolar width increased less than expander activation, and the midpalatal sutures were only opened slightly. Alveolar bone strain increased linearly with expander activation and decayed by 20% to 30% during postactivation intervals. Compressive strain at anchor-tooth alveolar bone locations was directed occlusally and apically, related to tooth tipping, and significantly higher than that at nonanchor tooth locations. With expander activation, suture strains increased monotonically and tended to plateau. Suture strain magnitude was generally similar to physiologic (masticatory) strains reported in the literature. The dominant strain polarity was compression at the maxillary-zygomatic and zygomatic-temporal sutures, but there was tension at the maxillary-premaxillary suture.
Conclusions
In these pigs, palatal expansion can cause significant occlusal-apical compression at buccal alveolar bone and physiologic-level strains at circummaxillary sutures.
Rapid palatal expansion (RPE) is a commonly used technique in orthodontics for patients with maxillary constriction or arch-length deficiency. The effectiveness of expansion screw appliances to open the midpalatal suture has been confirmed by extensive studies. Typically cemented on maxillary posterior teeth, the expander must counteract tissue resistance from the midpalatal suture and surrounding structures such as the dentoalveolus, nasal cavity, and circummaxillary sutures. As a result, in addition to opening the midpalatal suture, the expansion force might also affect these surrounding structures.
The effect of RPE on alveolar bone was recently investigated with computed tomography imaging. By using different measuring methods, these independent studies reached a common finding that the buccal cortical bone height of the posterior teeth was reduced after RPE, and the reduction was more severe at the anchor-tooth locations. This bone reduction has been attributed to tooth tipping, which often accounts for a large portion of total RPE expansion. The biologic change that leads to this reduction, however, is most likely initiated by the mechanical loading applied to the alveolar bone during RPE.
RPE mechanics might also influence the circummaxillary sutures. A number of clinical studies have suggested that RPE can disarticulate the circummaxillary sutures. It was also proposed that opening the midpalatal suture might move the maxilla forward and downward, supported by the finding that RPE created separation of bony segments around the spheno-occipital synchondrosis in monkeys. Because of these potential effects, RPE was advocated by some as an assisting treatment for maxillary facemask protraction for patients with maxillary retrognathia (or hypoplasia). A recent random controlled trial and a meta-analysis, however, did not find that RPE significantly enhanced maxillary protraction. One important question to ask about these conflicting findings is what changes RPE can bring to the circummaxillary sutures.
To understand the biologic changes at the alveolar bone and circummaxillary sutures in response to palatal expansion, it is necessary to characterize the mechanics received by these structures during palatal expansion. With finite element models generated from dry human skulls, 2 recent studies reported that, during simulated midpalatal suture expansion, maximal compressive stress was on the alveolar bone, and the maxilla was displaced downward and forward. However, these computational findings based on dry human skulls need to be validated by direct measurements. Strain gauge readings from bone are the gold standard for such validation and have been used before in craniofacial physiology and orthopedics.
The purpose of this study, therefore, was to directly characterize mechanical strain received by the alveolar bone and circummaxillary sutures during acute midpalatal suture expansion by using strain gauges. Because of the invasiveness of the technique, a pig model, which has strong similarity in craniofacial anatomy and function to the human, was used in this study. Specifically, we hypothesized that (1) the buccal alveolar bone is strained during palatal expansion, and the anchor tooth location receives heavier strain than the nonanchor tooth location; and (2) the circummaxillary sutures are strained during palatal expansion, and their strain pattern supports the theory that RPE can mechanically disarticulate circummaxillary sutures.
Material and methods
Domestic pig ( Sus scrofa ) heads were used in this study. The pig differs from the human with a long snout and a distinct premaxilla connected to the maxilla by the maxillary-premaxillary suture. In the human, the maxillary-premaxillary suture is completely fused at the facial aspect by 3 to 5 years of age and partially fused at the internal and palatal aspects by the teen years; therefore, a premaxilla is not distinct in human postnatal life. Despite this difference, however, the molar, maxillary, and zygomatic regions and the masticatory muscles of the pig are similar to those in the human in anatomy and function. The pig midplatal suture is also anatomically comparable with that of humans and has been used for RPE studies. In addition, physiologic strains of many pig craniofacial structures during mastication are already available, allowing comparison of the mechanics caused by RPE and normal function. These reasons made the pig a suitable model for this study. To reduce the difference between live and dead tissues, all animal heads used in this study were stored at –20°C and thawed overnight at room temperature before the experiments.
Two age groups, 3 and 6 months, were used in this study. These 2 groups were chosen because, based on overall craniofacial skeletal maturity, they are comparable with human preteens (9-12 years) and late teens (>16 years), respectively, and we wanted to compare alveolar bone and circummaxillary suture strains between these 2 ages. The exact fusion time of the pig midpalatal suture is currently unclear, but the midpalatal sutures in 3- to 6-month-old pigs are most likely still patent because the maxilla is still growing in the transverse dimension, and the suture is still visible on computed tomography images of 200-week old pigs. The pig heads were obtained from the Ohio State University Laboratory Animal Resources (3-month group [A]) and commercially from The Herman Falter Packing Company, Columbus, OH (6-month group [B]).
In clinical practice, palatal expansion appliances are often anchored on the first maxillary permanent molars. For the 3-month-old pigs, these teeth are not available because they are still largely unerupted ( Fig 1 , A ). The third deciduous molar (Dm3), which has similar location and morphology to the first permanent molar, therefore, was used as the alternative anchor tooth for the expansion appliance for both ages. Dm3 typically does not exfoliate until 12 to 15 months of age in pigs, and these teeth are still stable at ages 3 and 6 months, making them mechanically acceptable for anchor expansion appliances. For 6-month-old pigs, although their maxillary first permanent molars were already erupted, Dm3 was used to for consistency with the 3-month-old pigs.
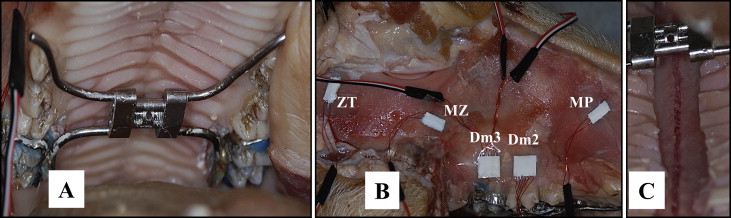
To enhance appliance retention, notches (1 mm deep) were made on the buccal and lingual enamel surfaces of the anchor teeth (Dm3) with a dental bur, followed by fitting orthodontic bands. An impression of the maxillary posterior teeth was taken and poured into casts. A hyrax expander was then fabricated by soldering the bands to the posterior arms of the appliance. The anterior arms were bent to contact the mesial surface of the first deciduous molars (Dm1). There was no direct contact with the lingual surface of the second deciduous molars (Dm2), which were used as the nonanchor teeth. The lack of direct contact of the expansion appliance with these teeth allowed us to differentiate alveolar bone bending from tooth tipping. The fabricated hyrax appliance was cemented with Band-Lok (Reliance Orthodontic Products, Itasca, Ill) ( Fig 1 , A ).
The soft tissue covering the right maxilla and zygomatic bone was reflected. Bone surfaces at the desired strain gauge placement locations ( Fig 1 ) were treated with conditioner and neutralizer (Vishay Measurements Group, Raleigh, NC) and dried with air following a standard procedure. Two rectangular rosette gauges (C2A-06-030WW-350, Vishay) were attached to the cervical middle third of the buccal alveolar bone of Dm3 (anchor tooth) and Dm2 (nonanchor tooth). Single element gauges (C2A-06-125LW-350) were attached perpendicularly to the maxillary-premaxillary, maxillary-zygomatic, and zygomatic-temporal (horizontal) sutures. No gauge was attached to the midpalatal suture because displacement at this suture was expected to be several millimeters, too large for strain gauges to measure. Before attaching the gauges to the sutures, a 2-mm-wide ultra thin plastic strip was placed above the sutures to shield them from being glued ( Fig 1 , B ). The wires of the strain gauges were connected to a strain gauge amplifier (A2, Vishay) and then to the MP150 system (Biopac Systems, Coleta, Calif) and a computer running AcqKnowledge III software (Biopac). As a dimensionless quantity of tissue deformation, strain will be reported as microstrain (μϵ) in this study, and each 1000 μϵ equals 0.1% deformation of the original length.
The pig jaws were held open (gape, about 30°) with a block placed between the upper and lower anterior teeth for expander access. All gauges were balanced, and a baseline recording was taken. Activation of the expander was performed with care to avoid moving the head or changing the position of the maxilla. A 90-second resting interval separated every 2 activation turns (0.25 mm expansion turn according to the appliance design). Strains from alveolar bone and sutures were continuously recorded. All animals received 20 turns of expander activation except for the first animal, which had only 12 turns because of appliance failure.
After the expansion was complete, a second impression was taken. Due to time constraints, only 1 midpalatal suture was directly examined for disarticulation immediately after the procedure. The other pig heads were refrozen and examined later.
Intermolar width was measured from preactivation and postactivation casts to evaluate the expansion. For each pair of teeth (Dm3 and Dm2), the distance was measured from the distal and mesial cusp tips, and the average values were calculated. The evaluator (B.C.T.) was blinded to the identity of the casts.
The recorded strain data were analyzed by using Acqknowledge III. The average strain magnitudes before, during, and after each activation of the expander were quantified. Strain polarity on the sutures was directly read from the strain gauge recordings; the directions of the principal alveolar bone strains were calculated based on the 3 channels of the rosette gauges.
Results
Intermolar width increases after 20 turns of activation are shown in Table I . No age-group difference was found, so the data of all animals were combined. All animals had smaller intermolar width increases than the theoretical amount of expander opening (5 mm). Compared with Dm2 (nonanchor teeth), Dm3 (anchor teeth) had significantly greater intermolar width increase (paired t test, P <0.05).
Animal ∗ | Intermolar width increase (mm) | Tensile strain | Compressive strain | Shear strain † | ||||
---|---|---|---|---|---|---|---|---|
8–turn activation | Dm3 | Dm2 | Dm3 | Dm2 | Dm3 | Dm2 | Dm3 | Dm2 |
A1 | ND | ND | 221.1 | 119.5 | –204.1 | –23.9 | 425.2 | 143.4 |
A2 | ND | ND | 233.1 | 40.2 | –565.7 | –7.7 | 798.9 | 47.8 |
A3 | ND | ND | 156.6 | 142.2 | –165.1 | –38.3 | 321.6 | 180.5 |
A4 | ND | ND | 67.6 | 109.0 | –238.2 | –32.3 | 305.7 | 141.2 |
B1 | ND | ND | 105.2 | 130.8 | –194.6 | –1.6 | 299.8 | 129.2 |
B2 | ND | ND | 259.1 | 249.6 | –184.0 | –38.0 | 443.0 | 287.6 |
B3 | ND | ND | 76.8 | 111.3 | –77.3 | –17.4 | 154.1 | 128.8 |
B4 | ND | ND | 31.5 | 18.8 | –30.9 | –5.8 | 62.5 | 24.6 |
Mean | 143.9 | 69.7 | –207.5 | 14.7 | 351.4 | 80.5 | ||
SD | 86.0 | 0.6 | 160.3 | –0.7 | 221.1 | 0.6 | ||
Dm3 vs Dm2: P ‡ | 0.34 | 0.01 | 0.03 | |||||
20–turn activation | ||||||||
A1 | ND | ND | ND | ND | ND | ND | ND | ND |
A2 | 3.05 | 2.09 | 447.4 | 186.2 | –977.7 | –33.9 | 1425.1 | 220.2 |
A3 | 2.46 | 0.43 | 334.2 | 234.6 | –452.8 | –51.5 | 787.1 | 286.1 |
A4 | 1.32 | 0.17 | 124.8 | 224.6 | –407.2 | –52.1 | 532.0 | 276.8 |
B1 | 2.96 | 0.98 | 283.2 | 392.5 | –522.1 | –18.3 | 805.3 | 410.8 |
B2 | 3.30 | 1.21 | 531.9 | 516.0 | –365.5 | –63.5 | 897.3 | 579.5 |
B3 | 0.78 | 0.19 | 73.4 | 49.8 | –183.1 | –9.9 | 256.5 | 59.7 |
B4 | 1.55 | 0.76 | 66.3 | 25.2 | –75.1 | –5.3 | 141.4 | 30.4 |
Mean | 2.20 | 0.68 | 265.9 | 232.7 | –426.2 | –33.5 | 692.1 | 266.2 |
SD | 0.98 | 0.63 | 188.6 | 157.3 | 255.5 | 22.1 | 374.6 | 166.1 |
Dm3 vs Dm2: P | 0.51 | 0.01 | 0.02 |
∗ A , 3-month group; B , 6-month group
† Shear strain is the magnitude of maximum strain (usually positive) minus the magnitude of minimum strain (usually negative)
‡ Difference between Dm3 and Dm2 locations was examined by paired t tests.
Direct examination of the palate after removing overlying soft tissue showed that all animals still had patent midpalatal sutures. The midpalatal suture was disarticulated at the palatal surface in all animals except for 2 in the older group (B3 and B4). An example of midpalatal suture disarticulation is shown in Figure 1 , C . The palatal openings of all disarticulated midpalatal sutures ranged between 0.5 and 1.5 mm, smaller than the intermolar width increase at Dm3.
Longitudinal change of average alveolar bone strain throughout the acute expansion is exemplified in Figure 2 , A . At Dm3, principal strains, both compression and tension, increased in a strong linear fashion (Pearson tests, r >0.97) in all animals except one (B3). Tensile strain at the Dm2 location also demonstrated a strong linear increase (Pearson tests, r >0.96) in all animals except one (B4), and compressive strain at the Dm2 location remained near 0 for all animals.
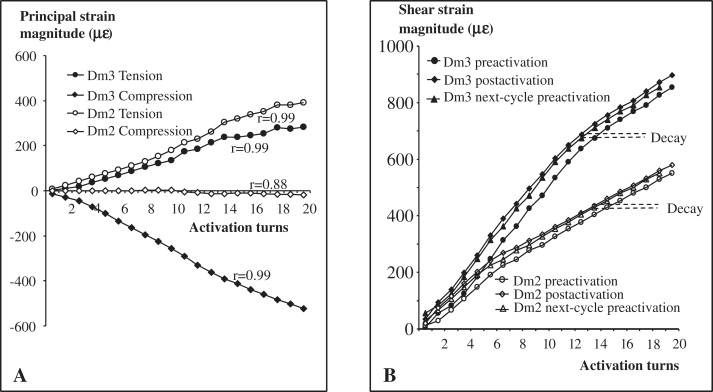
During the 90-second interval between 2 consecutive activations, bone strain showed some decay ( Fig 3 , B ). After each activation, strain decayed by 27% (range, 15%-43%) at Dm3 and 17% (range, 5%-35%) at Dm2 on average (with the 2 age groups combined, no group difference was found).
Alveolar bone strain values after 8 and 20 turns of expander activation are shown in Table I . Overall, alveolar bone strain varied greatly among the animals. Two animals (B3 and B4) had substantially smaller strains than the others. With age, the average strain values tended to decrease at Dm3, but this difference was mainly caused by animals B3 and B4. Therefore, the 2 age groups were combined for strain comparison between the Dm3 and Dm2 locations. Both compressive and shear strains were significantly higher at Dm3 than at Dm2 (paired t tests, P <0.05), whereas tensile strain values were similar between locations. The compressive strain at Dm2 was so low that it was only comparable with gauge baseline levels.
The directions of the principal compressive and tensile strains were calculated from 3 channels of the rosette gauges ( Table II ). Most animals maintained a constant orientation of the principal strains throughout the entire expansion course, as shown by small standard deviations among activation cycles. Regardless of age, the principal compression at Dm3 was in an occlusal-apical direction approximately perpendicular to the occlusal plane ( Fig 3 ). For Dm2, the principal tensile strain was parallel to the occlusal plane ( Fig 3 ).
Bone principal strain direction (°) ∗ | Suture strain polarity | ||||
---|---|---|---|---|---|
Animal | Dm3 compression | Dm2 tension | MP | MZ | ZT |
A1 | 104.8 ± 1.6 † | − 53.3 ± 1.4 | – | – | – |
A2 | 75.1 ± 2.4 | − 5.6 ± 15.5 | + | – | – |
A3 | 75.6 ± 3.1 | 14.1 ± 3.5 | + | – | – |
A4 | 82.5 ± 3.3 | − 12.9 ± 1.9 | + | – | – |
B1 | 105.4 ± 7.2 | 10.8 ± 4.2 | + | – | – |
B2 | 80.7 ± 0.4 | 28.3 ± 0.9 | – | – | – |
B3 | 90 ± 5.5 | − 16 ± 3.6 | + | + | – |
B4 | 103 ± 16 | 18 ± 8.4 | – | + | – |
Mean ± SD | 89.6 ± 13.1 | − 2.1 ± 25.9 |
∗ Degrees are relative to the occlusal plane ( Fig 3 ) with 0° pointing forward. Positive and negative values indicate counterclockwise and clockwise rotations, respectively, away from 0°
† Means and standard deviations at 20 turns of expander activation.
An example of longitudinal suture strain change during the acute expansion is shown in Figure 4 , A . All locations increased monotonically during the first 8 to 10 turns of activation and then tended to reach a plateau or to decline.
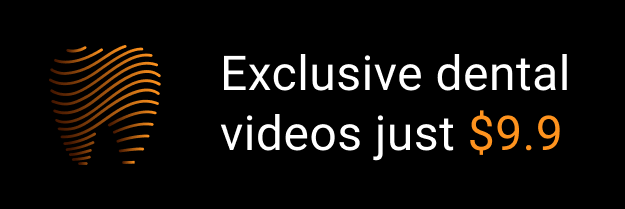