Introduction
The main advantage of superelastic nickel-titanium (NiTi) products is their unique characteristic of force plateaus, which allow for clinically precise control of the force. The aims of this study were to define the mechanical characteristics of several currently available closed-coil retraction springs and to compare these products.
Methods
A universal test frame was used to acquire force-deflection diagrams of 24 NiTi closed-coil springs at body temperature. Data analysis was performed with the superelastic algorithm. Also, the influence of temperature cycles and mechanical microcycles simulating ingestion of different foods and mastication, respectively, were considered.
Results
Mechanical testing showed significant differences between the various spring types (ANOVA, ≤0.05), but constant intrabatch behavior ( t test). Four groups were formed according to the mechanical properties of the springs: strong superelasticity without bias stress, weak superelasticity without bias stress, strong superelasticity with bias stress, and weak superelasticity with bias stress.
Conclusions
In sliding mechanics, the strongly superelastic closed-coil springs with preactivation are recommended. In addition, we found that the oral environment seems to have only a minor influence on their mechanical properties.
Nickel-titanium (NiTi) materials have become more popular in the last decade. Their main advantage is their nonlinear force deflection behavior, resulting in the expression of force plateaus. Within these plateaus, NiTi products are relatively insensitive to imprecise activation. Their clinical applications are therefore much easier than with conventional alloys. However, plateau force levels vary widely, and product information from manufacturers is not always reliable. Clinicians must have accurate, unbiased information on the properties of the springs.
The superelastic (SE) coil springs we studied are mainly used for canine retraction. There is no consensus about the optimal force for canine distalization and probably never will be because of individual variations. Forces between 1 and 2 N are most commonly accepted for canine retraction. These quoted variations in applied forces are closely related to the bracket and wire system used, because they have different frictional forces to overcome. To apply forces in this range with conventional alloys, the mechanics dictate that they must be reactivated several times. In contrast, NiTi retraction coil springs allow for treatment with 1 activation, with the force maintained even over the distance of a whole extraction site. This has the following advantages: reduced chair time, optimal rates of distalization, and conservation of anchorage.
However, NiTi products are temperature sensitive, and small differences in alloy composition can lead to considerable differences in the mechanical characteristics of individual SE coil springs. It is important to consider oral temperature changes from ingestion of different types of food. Meeling and Odegaard investigated the torsional behavior of several SE archwires. They found that the influence of temperature changes on the activation curve is opposite to the deactivation curve and that temperature changes lead to clinically significant changes in force level.
In addition to oral temperature changes, repetitive mechanical microdeflections caused by tongue play or mastication might also cause changes in the force level of NiTi springs. However, we found no data reporting the influence of mechanical microcycles on SE materials in the literature. Theoretically, it might be expected that an increase of stress-induced martensite (SIM) would lead to a decrease of plateau force level. The amount by which the force could decrease, however, is unknown.
Because of the nonlinear force-deflection relationship of SE alloys, the prediction of mechanical properties becomes challenging. Several attempts have been made to characterize the plateaus of NiTi products such as archwires or coil springs. Melsen and Terp proposed a regression line connecting maximum force and force at 0.5-mm activation to compare SE products. However, this procedure allows only a rough approximation of the plateau. Another proposal is the SE ratio by Segner and Ibe, relating the maximum and minimum slopes on the deactivation curves. This procedure allows for classification into products with SE tendency (ratio, ≥2), superelasticity (ratio, ≥8), or no SE behavior. But this approach does not tell the orthodontic practitioner the typical force level of the plateau. A more sophisticated approach is described by the SE algorithm ( Fig 1 ). Based on a modification of the SE ratio, it allows for identification of the extension and the force level of the clinically relevant plateau by means of a mathematical calculation and is especially valuable for unbiased comparisons of different products. The aims of this study were to define and compare the mechanical characteristics of several currently available closed-coil retraction springs. Furthermore, the influence of temperature and mastication was investigated.
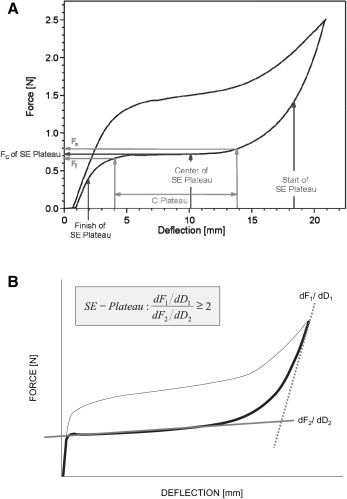
Material and methods
Table I lists all closed-coil springs included in this study. The mechanical properties were examined in a tensile test at a displacement rate of 1 mm per second –1 with a universal test frame (model 4444, Instron Corp, Wilmington, Del). The load frame was equipped with a ±100-N static load cell (serial number, UK480), and the temperature was kept constant at 37.0°C (±0.1°C) by submersing the samples and fixtures in a water bath. Temperature control and agitation of the water bath was achieved by a thermostat (FS 18 HP, Julabo, Seelbach, Germany). Closed-loop temperature control was achieved with an external resistive precision temperature sensor (PT100, Haraeus Sonsore-Nite GmbH, Kleinostheim, Germany) in the water bath. The sensor was calibrated at 0°C in a water-ice mixture ( Fig 2 ).
Number | Manufacturer | Product | Size (mm) | Force (N) | Catalog number | Lot |
---|---|---|---|---|---|---|
1a | Dentaurum | Rematitan Lite | 9 | — | 758-160-00 | 26649 |
1b | Dentaurum | Rematitan Lite | 12 | — | 758-161-00 | 27490 |
2a | Forestadent | Titanol Instant Zugfeder extra light | — | — | 311-1026 | 231 |
2b | Forestadent | Titanol Instant Zugfeder light | — | — | 311-1027 | 20218439 |
2c | Forestadent | Titanol Instant Zugfeder medium | — | — | 311-1028 | 061 tc |
3a | GAC | Coil springs ultra light | — | 0.25 | 10-000-26 | A5Z9 |
3b | GAC | Coil springs extra light | — | 0.50 | 10-000-25 | A322 |
3c | GAC | Coil springs light | — | 0.98 | 10-000-03 | A462 |
3d | GAC | Coil springs medium | — | 1.47 | 10-000-02 | A522 |
3e | GAC | Coil springs heavy | — | 1.96 | 10-000-01 | A222 |
3f | GAC | Coil springs extra heavy | — | 2.45 | 10-000-18 | A332 |
4a | Masel | Elastinol coil spring constant closed | 9 | 0.50 | 4107-319 | 87665 |
4b | Masel | Elastinol coil spring constant closed | 9 | 0.98 | 4107-320 | 78770 |
4c | Masel | Elastinol coil spring constant closed | 9 | 1.47 | 4107-321 | 76589 |
4d | Masel | Elastinol closed | 9 | 1.96 | 4107-322 | 5697 |
4e | Masel | Elastinol coil spring variable closed | 9 | — | 4107-309 | 86093 |
4f | Masel | Elastinol coil spring variable closed | 12 | — | 4107-312 | 5697 |
5a | Ormco | NiTi extension spring light force | — | — | 222-5610 | 02C114 |
5b | Ormco | NiTi extension spring medium force | — | — | 222-5612 | 02D74 |
5c | Ormco | NiTi extension spring heavy force | — | — | 222-5620 | 01L6 |
6a | Ortho Org. | Nitanium closing spring | 9 | — | 100-622 | 510873A02 |
6b | Ortho Org. | Nitanium closing spring | 12 | — | 100-623 | 332206 |
7a | RMO | Nitinol coil spring medium force | 9 | — | F0207 | 41916 |
7b | RMO | Nitinol coil spring medium force | 12 | — | F0208 | 45650 |
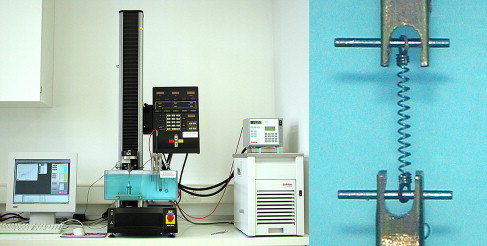
In the tensile procedure, the specimens were prestressed to a force level of 0.1 N to eliminate experimental error from mechanical play in the fixtures. Starting from the 0.1-N prestress displacement, the specimens were consecutively strained to displacements of 4, 8, 15, 20, 25, and 30 mm. Straining was done twice, and only the second data set was used for evaluation to prevent further experimental error from mechanical setting in the fixtures and the crimping connections between the NiTi coil components and the stainless steel attachments of the retraction coils. Five coil springs were measured for each group.
Time, force, and displacement data were acquired electronically with data-acquisition and machine-control software based on Origin7pro (RockWare, Golden, Colo) through the machine’s IEEE488 interface. The data acquisition rate was more than 10 samples per second –1 , corresponding to a displacement resolution of better than 0.1 mm at the chosen speed.
The same setup was used for further evaluation of certain NiTi springs. Two specimens from each coil type were tested for their behavior under repetitive mechanical microcycles of 2 mm expansion and environmental temperature changes. For the evaluation of mechanical microcycles, the coil springs were activated to 15 mm and deactivated to the plateau midpoint evaluated by the data analysis of the above-described mechanical testing. The springs were expanded 20 times. For the thermal evaluation, the handling of the specimens was identical to the setup for the mechanical microcycles. Instead of mechanical influences, 2 thermocycles between 4°C and 60°C were applied to the specimens, starting with the heating side.
The acquired data were analyzed for the clinically important plateau phase by using the SE algorithm ( Fig 1 , A ). Unlike previous methods, this algorithm allows for a mathematical definition of the orthodontically relevant force plateau and is therefore suitable for product comparisons. It is based on the SE ratio defined by Segner and Ibe. This ratio describes the relationship between the maximum slope in the terminal region of the deactivation curve and the minimal slope in the plateau region. A ratio ≥2 is defined as “SE tendency.”
Because of integrated preactivation in some products, the SE ratio is not suitable for the evaluation of all products and had to be modified. A reliable relationship can be achieved by comparing the maximum slope of the initial parts of the deactivation curve with the plateau slope instead of the final slope of the deactivation curve ( Fig 1 , B ). By displaying all data points with a modified SE ratio ≥2 on the graph, a plateau-like region can be generated. The clinically relevant “C plateau” is then defined by evaluating the deactivation curve for the range with the largest span on the deflection axis and a force range of 20% of the SE plateau midpoint force.
Statistical analysis
Statistical evaluation was performed by evaluating means and standard deviations for plateau extension, slope, force level, and plateau midpoint. In addition, analysis of variance (ANOVA) (the Student-Newman-Keuls test) was calculated for the deflection of 15 mm, classifying the springs at a probability level of 0.05 for the 2 criteria of plateau length and plateau slope.
Results
Figure 3 displays the clinical plateaus for an activation of 15 mm, evaluated by the SE algorithm. According to the SE algorithm, only products achieving an SE ratio of at least 2 (SE tendency) were evaluated. The results show that plateau height and localization varied considerably with different activation levels. Four groups were formed according to the mechanical properties of the springs ( Table II ): (1) strong superelasticity without preactivation, (2) weak superelasticity without preactivation, (3) strong superelasticity with preactivation, and (4) weak superelasticity with preactivation.
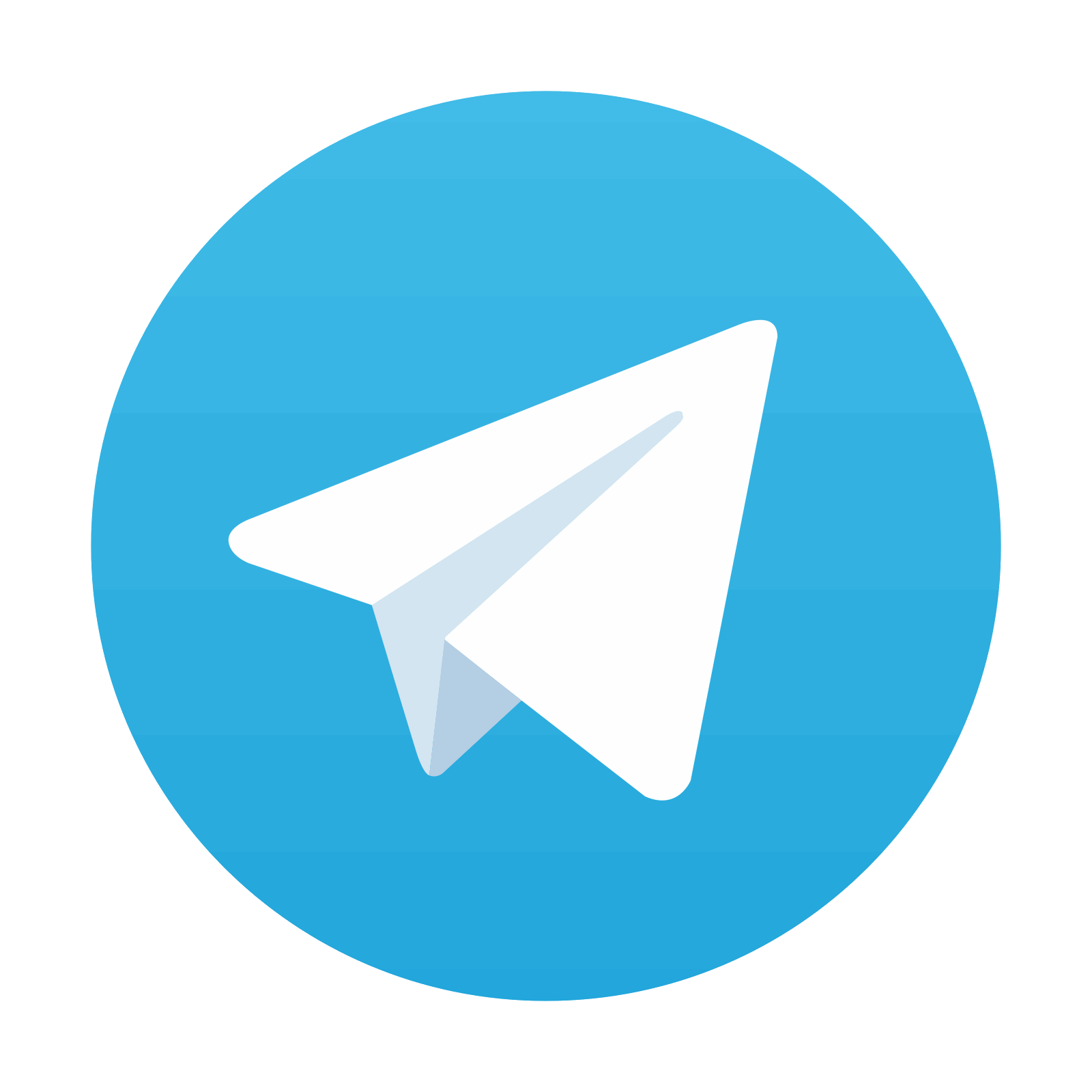
Stay updated, free dental videos. Join our Telegram channel

VIDEdental - Online dental courses
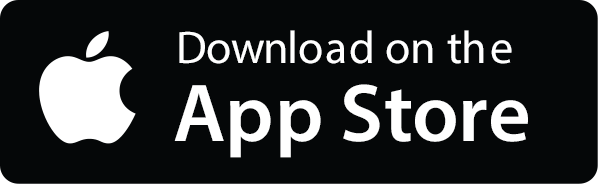
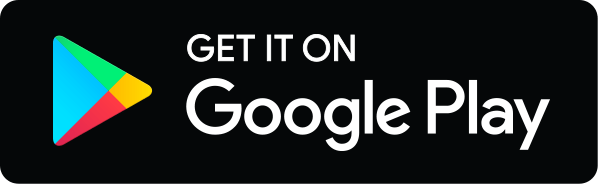