For additional ancillary materials related to this chapter, please visit thePoint.
Objectives
After studying this chapter, the student will be able to do the following:
1. List the phases into which materials are classified. Discuss the varying amounts of attraction between the molecules and atoms of each phase. Recall the differentiating characteristics of each phase.
2. Explain the basic difference between primary and secondary bonds.
3. Name the three types of primary bonds and describe the differences between them.
4. Summarize the similarities and differences of secondary bonds, which include permanent dipoles, hydrogen bonds, and fluctuating dipoles.
5. Contrast the bonding characteristics of metals, ceramics, plastics, and composites.
6. Compare any similarities and differences of colloids and emulsions.
Key Words/Phrases
amorphous
ceramic
colloids
composites
covalent bonds
cross-linking
crystalline
dental materials
emulsions
fluctuating dipole
hydrogen bonds
ionic bonds
long-range order
materials science
metallic bonds
metals
permanent dipole
polymers
primary bonds
secondary bonds
short-range order
valence electrons
Introduction
Many different kinds of materials are used to make the products we use in everyday life and in the profession of dentistry. Understanding the behavior of materials is important in the selection, placement, and maintenance of dental materials. The behavior of any given material is based on the atoms and the atomic bonds in that material. Many will think the material covered in this chapter is too theoretical for dental hygiene students. If so, the authors urge you to skip this chapter and read only the summary at the end.
I. Materials Science
Materials science is the part of the physical sciences that seeks to explain the properties and performance of materials by examining their internal structure. Materials science is a combination of chemistry, physics, and engineering rather than a separate scientific domain. Materials science tries to explain why materials behave as they do, based on the atoms and molecules in materials and the bonds that exist between these atoms and molecules. Materials science also tries to understand the effects of manufacturing processes on materials and any changes in materials that may occur during the useful life of a product. In dentistry, a subgroup of materials science has developed. This subgroup, called dental materials, is part of the larger field of biomaterials and, at times, is called dental biomaterials. Whatever term we use, however, the goal is to understand why materials behave the way they do and how the clinician can maximize the performance of these materials.
In this book, the handling (processing) of dental materials will be stressed, and a discussion of the underlying materials science will be included as well. At times, simply memorizing step-by-step procedures will seem to be much easier, but understanding their nature will simplify the use of the vast number of materials currently on the market. No other branch of scientific inquiry has a greater impact on our day-to-day lives than the development of new materials and the innovative ways in which these materials are being used.
Another branch of materials science, food science, is important to our everyday lives. The same laws of nature that govern dental materials also make ice cream creamy, fudge chewy, and Jell-O jiggle. Whenever possible, everyday examples of these concepts are included in this text.
II. Atomic Bonding
How do teeth withstand the forces that occur when we bite and grind food? To understand the strength of teeth, we need to understand the nature of atomic bonds. Teeth and restorative materials need to be stronger—and to have stronger atomic bonds—than the food we eat.
A. Phases
We commonly classify materials into one of the three phases: solid, liquid, or gas. Familiarity with these phases will provide a foundation for understanding the nature of the forces that hold atoms together in materials. Later in this chapter, we discuss colloids, which are a mixture of two of these phases.
1. Gases
The atomic bonds between gas molecules are very weak. These bonds are easily broken by the normal microscopic vibrations of atoms at room temperature. These atomic vibrations are the result of the thermal energy of the material. Gases have no molecular organization and will take on the three-dimensional shape of the container that they fill. If thermal energy is removed by cooling, gases condense into liquids. An example is the condensation of water vapor on the outside of a mug of ice-cold root beer on a hot, humid day.
2. Liquids
Liquids have stronger attraction between molecules than gases do, but this attraction is not strong enough to carry a load or to maintain a shape without support. The attraction between molecules results in short-range order. Short-range order is a consistent spatial relationship among atoms or molecules 5 to 10 neighbors apart. Liquids lack long-range order. Molecular attraction keeps liquids from boiling, but not always from evaporating. Other characteristics of a liquid are vapor pressure, boiling temperature, viscosity, and surface tension.
3. Solids
Solids exhibit the strongest attraction between atoms and molecules. The atomic bonds of solids maintain the shape of objects and resist external forces placed on them. Solids can be classified as crystalline or amorphous. Crystalline solids have a consistent spatial relationship of atoms or molecules repeated hundreds to thousands to millions of times that is called long-range order (Fig. 2.1A). The distances and angles among such atoms or molecules are uniform, much like rooms in a dormitory or hotel. Table salt, diamonds, and the hydroxyapatite of teeth are crystalline solids. Crystalline solids have both short-range and long-range order of their atoms or molecules.
FIGURE 2.1. A two-dimensional depiction of the arrangement of atoms in a crystal A. and an amorphous solid B.
Amorphous solids (Fig. 2.1B) have the same strong atomic bonds present in crystalline materials, but have only short-range order, much like liquids. The long-range order of an amorphous solid is more irregular or disorganized. The glass in a window or a dental mirror is an amorphous solid.
Some solid objects are strong, while others are weak. The difference is determined by the type of atoms that make up the material and the strength of the atomic bonds that hold the material together.
B. Atomic Bonds in Solids
How does a strong solid object know how much force it needs to resist the load of an object resting on its surface? If you place a 2-lb book on a table, the table pushes up with 2 lb of force, just enough to support the book—not too little and not too much. How does the table know to push up with 2 lb of force? This “smart” behavior is a characteristic of solid objects and a function of the nature of atomic bonds in materials.
Atomic bonds are a result of electromagnetic (EM) force. For this discussion, we will focus on the electronic part of the EM force. The electronic force causes positive charges to attract negative charges and negative charges to attract positive charges. Positive charges are repelled by positive charges, and negative charges are repelled by negative charges. The magnetic force exhibits similar attraction and repulsion and is easily demonstrated with two magnets. We will use the familiar magnetic force to help us understand attraction and repulsion. The north pole of a magnet attracts the south pole and repels the north pole. It takes force to bring the north poles of two magnets into proximity. The closer they get, the harder you have to push, much like a spring. In fact, the atomic bonds between atoms act much like the springs of a mattress or a car.
To compress a spring, it must be pushed. To stretch a spring, it must be pulled. When no force is applied to a spring, it is said to be unloaded and has an equilibrium length. When force is applied, the spring changes length and resists the applied force by developing an equal and opposite force. Solids can be thought of as a collection of millions and millions of springs or atomic bonds. When you place a book on a table, the atomic bonds of the table are compressed and oppose the force of the book. The heavier the book, the more the bonds are compressed and the greater the force that is developed in the table. Is this really true? We do not see the table change height when we put the book on it. We must remember that the table has millions and millions of atoms and, therefore, millions and millions of atomic bonds. Each bond is compressed a submicroscopic distance. The total of the change in length of these bonds is still microscopic, too small to be seen by the unaided eye, but the change in height of the table can be measured with specialized equipment. Again, the atomic bonds of the table are compressed when a book is placed on it, and these bonds act like springs. When one stretches a rubber band, the same phenomenon occurs, but it can be seen by the unaided eye. Different kinds of internal atomic bonds respond to external forces in different manners.
The change in the height of the table when a book is placed on it is an example of something that happens we cannot see. These changes may be microscopic, or they may occur very slowly and, thus, may not be noticed. Such changes occur in dentistry: teeth compress when biting, erupt into the mouth, change color during a person’s lifetime, wear, or dissolve in acid, forming caries.
C. Primary Bonds—Optional
Primary bonds are the strong bonds between atoms that involve the transfer or sharing of electrons between atoms. In high school and college chemistry classes, the ionic and covalent bonds are presented. Another bond that is important to the understanding of certain materials is the metallic bond. All bonds are a result of the EM force and the distribution of positive and negative charges of atoms and molecules.
The atom is made of protons, neutrons, and electrons. The protons and the neutrons make up the nucleus; the electrons move around the nucleus in shells. Electrons in the outer shell of atoms are involved in chemical reactions and atomic bonding. They are called valence electrons. When discussing atomic bonding, it is easier to group the nucleus with the nonvalence electrons into what is called the “positive core.” The remaining valence electrons are those that are principally involved in atomic bonds. We can therefore restrict our discussion to the positive core of the atom and the valence electrons. Explaining why some elements form metallic bonds and others form covalent bonds is beyond the scope of this text (and its authors’ understanding of the subject). However, understanding how atoms fill up their outer shell with electrons from other atoms will help us to understand the nature of atomic bonds and give us some insight regarding why materials behave as they do.
1. Ionic Bonds
Ionic bonds are the result of an electron being given up by one atom and being accepted by another. Why does an atom give up or accept an electron? Atoms are “content” when their outer shell of electrons is full; in this situation, they have a lower state of energy. An element like sodium has one valence electron in its outer shell, and it will readily give up this valence electron. If a sodium atom gives up this valence electron, the next inner shell becomes the outer shell and is a full shell. The atom then becomes an ion with a charge of +1. An element such as chlorine, however, is one electron short of filling its outer shell. Therefore, chlorine readily accepts an electron and becomes an ion with a charge of −1. When sodium and chlorine atoms have full outer shells, the opposite electrical charges of these ions attract each other and form atomic bonds. The result is NaCl or sodium chloride (table salt).
Chemical reactions, in which atoms fill their outer shells with electrons and form bonds, are examples of nature lowering the energy of a system. Other examples of systems lowering their energy include a ball rolling downhill, water flowing over a waterfall, a candle burning, and a battery powering a flashlight. The conversion of energy from one form to another (whether chemical, electrical, mechanical, or thermal) is governed by the laws of thermodynamics. Again, such forbidding subjects are beyond the scope of this text.
Let’s get back to the ionic bond. A grain of table salt (sodium chloride) has billions and billions of sodium and chloride ions. Ionic bonds hold the ions together. Oppositely charged ions attract each other (negative chloride ion and positive sodium ion). Ions with like charges repulse each other. The strength of the salt grain is the sum of the attraction of opposite charges minus the repulsion of like charges.
The distance between ions has an important effect on the strength of the attraction or repulsion. The strength of both is inversely proportional to the square of the distance between the ions. Thus, the force between the ions falls rapidly as the distance between the pair increases.
The arrangement of ions in sodium chloride results in the negatively charged chloride ions surrounding each positively charged sodium ion. Likewise, each negative ion is surrounded by positive ions. The familiar checkerboard pattern is a two-dimensional example of such an arrangement. As a result, the attraction between oppositely charged “next-door neighbors” is strong because they are close together. The repulsion of similarly charged ion pairs is weaker because they are farther apart (Fig. 2.2). The attraction forces overpower the repulsive forces, and the result is a strong material.
FIGURE 2.2. A two-dimensional depiction of an ionic material. The green arrows pointing toward each other represent the attraction of unlike-charged ions. These ions are closer; therefore, the force is stronger. The red arrows pointing away represent repulsion of like-charged ions. The like-charged ions are farther apart; therefore, the force is weaker.
2. Covalent Bonds
Covalent bonds between two atoms are the result of two atoms sharing a pair of electrons (Fig. 2.3). Sharing electrons with other atoms allows an atom to fill its outer shell with electrons and, thus, to lower its energy. How does sharing a pair of electrons bond two atoms together?
FIGURE 2.3. Three representations of covalent bonds between carbon atoms. In A. lines represent the bonds. In B. pairs of shared electrons represent the bonds. In C. the distribution of the electrons is shown. Note in C the alternating negative charges of the electrons and positive charges of the cores.
The physical space that an electron occupies around the nucleus is called an orbital, and it is centered around the nucleus when not involved in an atomic bond. When two atoms share an electron, the shape of the orbital changes. When a pair of electrons is shared and forms a covalent bond, the orbital surrounds both atoms. Now the electrons spend some time in the physical space between the two atoms. By spending time between the two atoms, bonding of the two atoms occurs. A partial negative charge is created by the presence of the electron pair between the two atoms. The partial negative charge of the bond is attracted to the positive cores of the two atoms that are sharing the electron pair. The positive core of the first atom is attracted to the relatively close partial negative charge (because of the electron pair spending time there) between the atoms. The same is true for the second atom that shares the electron pair.
The attraction of the partial negative charge to the two positive cores is greater than the repulsion between the positive cores because the distance between the two positive cores is greater. We have probably set organic chemistry back a century with this simplistic description of the covalent bond, but it will serve our purposes and help to explain the behavior of polymers (and it’s the best the authors can do).
Covalent bonds between the two atoms sharing the electron pair are strong and very directional. However, few materials are bonded with only covalent bonds. One well-known material that is covalently bonded is diamond. Each carbon atom is bonded to four other carbon atoms by a covalent bond. One of the hardest materials known results from this bonding. Many materials are the result of long chains of covalently bonded atoms. The chains are strong, but the materials are not always strong. This is because their properties are determined by the manner in which the long chains are bonded to each other. Polymers are long chains of covalently bonded carbon atoms. Examples of polymers include man-made plastics and rubbers as well as many biologic macromolecules, such as proteins and DNA. The varied properties of polymers and plastics will be better explained in the discussion of secondary bonds.
3. Metallic Bonds
Metals have characteristic properties that allow us to easily identify a material as being a metal. Metals are typically dense, heavy materials. They are good conductors of both electricity and heat, are cold to the touch at room temperature, and, if shaped properly, will ring like a bell if struck. All these properties of metals are a result of metallic bonds. Metallic bonds are similar to covalent bonds in that valence electrons are shared between atoms. The difference is that the electrons in a metal object are not shared by two atoms; instead, they are shared by all the atoms that make up that object (Fig. 2.4). We can illustrate this difference by comparing a marriage to students in a school. In a marriage, the relationship is between two people, and the sharing is between those two. Within a school, however, all students are part of the student body. They have a less intense relationship, but they all share the feeling of being part of the school.
FIGURE 2.4. A two-dimensional representation of the metallic bond. Note that the positive cores (nuclei and nonvalence electrons) are surrounded by an electron “cloud.”
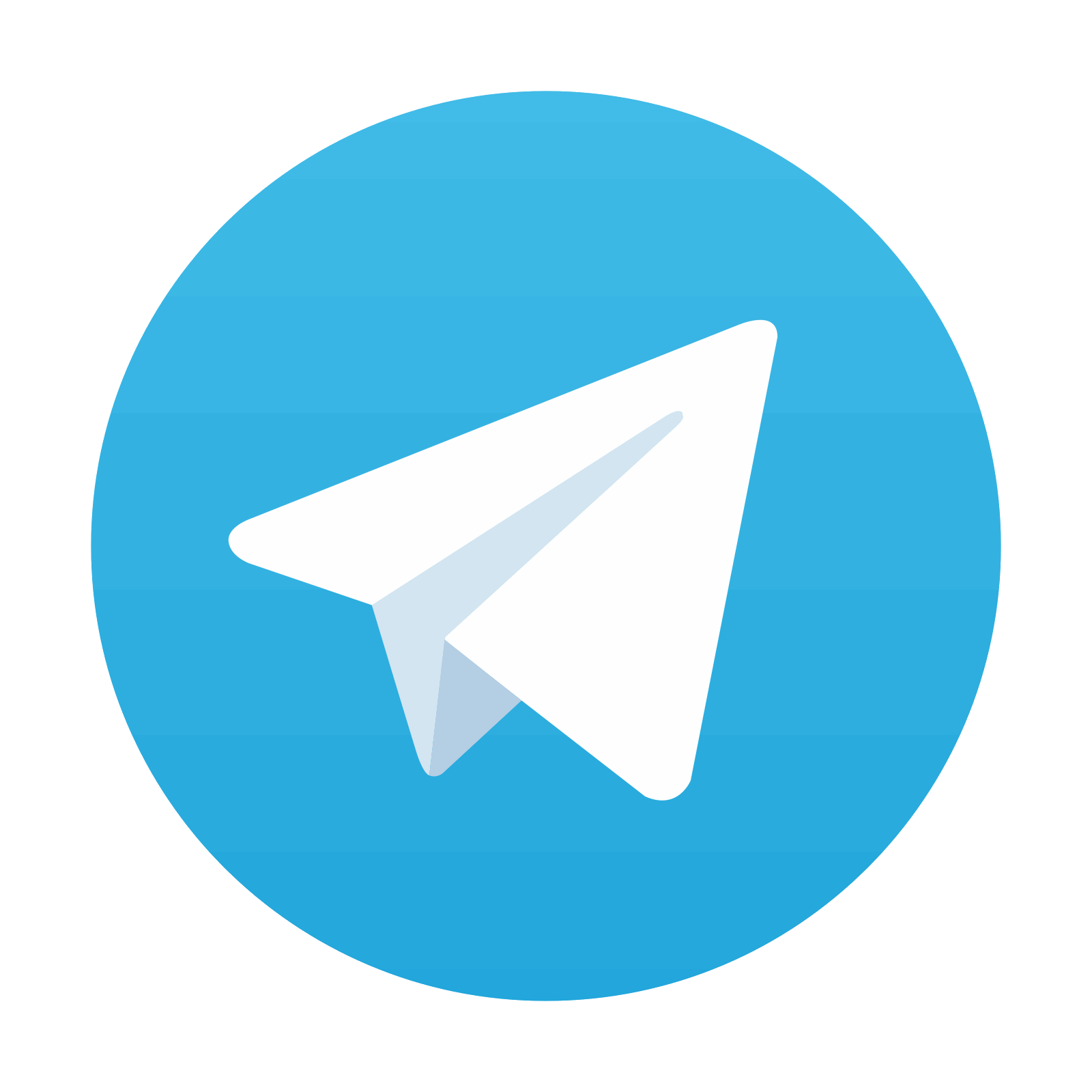
Stay updated, free dental videos. Join our Telegram channel

VIDEdental - Online dental courses
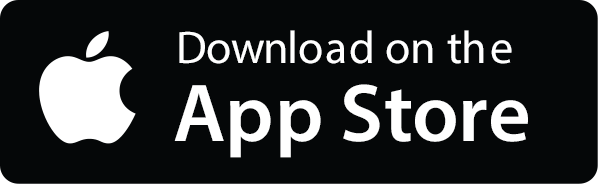
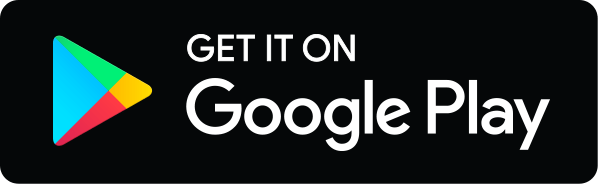