Introduction
The masticatory muscles are considered to be important determinants of facial form, but little is known of the muscle spindle reflex characteristics and their relationship, if any, to face height. The aim of this study was to determine whether spindle reflexes, evoked by mechanical stimulation of an incisor and recorded on the masseter muscle, correlated with different facial patterns.
Methods
Twenty-eight adult volunteers (16 women; ages, 19-38 years) underwent 2-N tap stimuli to their maxillary left central incisor during simulated mastication. Reflexes were recorded during local anesthesia of the stimulated tooth to eliminate the contribution from periodontal mechanoreceptors. Surface electromyograms of the reflex responses of the jaw muscles to these taps were recorded via bipolar electrodes on the masseter muscle and interpreted by using spike-triggered averaging of the surface electromyograms. Lateral cephalometric analysis was carried out with software (version 10.5, Dolphin, Los Angeles, Calif; and Mona Lisa, Canberra, Australia).
Results
Two-newton tooth taps produced principally excitatory reflex responses beginning at 17 ms poststimulus. Correlation analysis showed a significant relationship between these muscle spindle reflexes and facial heights: specifically, shorter face heights were associated with stronger spindle reflexes. This correlation was strongest between the derived measure of masseter length and the spindle reflex strength during jaw closure (r = –0.49, P = 0.008).
Conclusions
These results suggest that a similar muscle spindle stimulus will generate a stronger reflex activation in the jaw muscles of patients with shorter faces compared with those with longer faces. This finding might help to explain the higher incidence of clenching or bruxism in those with short faces and also might, in the future, influence the design of orthodontic appliances and dental prostheses.
Although substantial work has been done to evaluate the nature of various trigeminal reflexes, limited research has considered the influence of facial morphometry on such reflexes. Muscle spindles transduce muscle length changes and muscular tension during mastication, providing an important source of feedback to the masticatory control system. The efficacy of this feedback is undoubtedly modulated by dynamic interactions between facial geometry (which determines the length and orientation of the receptors) and the direction of jaw movement (which determines the sensitivity of the receptor as a function of muscle tension). Currently, there is little information about muscle spindle reflex modulation during jaw movements, primarily because of the inherent complexity of studying jaw reflexes during mastication.
Most often, the method used to elicit a muscle spindle reflex in the jaw involves a brief mechanical depression of the mandible, with either a tendon hammer or, if a more controlled stimulus is required, a computer-modulated stretching device. A significant limitation of such approaches is that their application introduces potential confounding receptor activations: perturbing joint and pressure receptors in concert with the muscle spindles. Furthermore, jaw depression cannot be applied during jaw movement without interrupting the ongoing movement cycle. An alternative method to the jaw-jerk reflex, the periodontal-masseteric reflex, has been used to assess jaw muscle spindle excitability. In these studies, a maxillary tooth is tapped, and the jaw muscle spindles are excited via vibration transmitted through the maxilla. Sensitivity of spindles to vibration is well established; hence, it is not surprising that vibration applied to a tooth can activate spindles in jaw muscles.
In this study, the maxillary incisor tap method of activating the jaw muscle spindles was used to avoid generating jaw movement that would stimulate other potentially confounding proprioceptors. Local anesthetic was used to block all periodontal mechanoreceptor activity from canine to canine in both jaws. These 2 precautions gave us the best method of studying jaw muscle spindle reflexes in subjects with different face heights.
To the best of our knowledge, no authors have previously investigated muscle spindle response and facial height proportions during dynamic masticatory movements. The aim of our study was to investigate possible correlations between representative facial height measurements and jaw muscle spindle reflex responses from the masseter muscle. Our hypothesis was that muscle spindle reflex responses are not similar in all face types. The rationales for this proposal were that the length of the spindle and the length of the muscle are positively related, and the same amount of vibration stimulus applied to a muscle will more effectively activate shorter spindles. In practice, this would mean that subjects with short faces will have a stronger tonus in the jaw muscles compared with those with long faces, and might therefore have more likelihood of developing muscle contraction-related problems. If this hypothesis is proven, it might help to validate the thickness chosen for plates used to manage bruxism and explain why orthodontic treatments (primarily functional orthopedic appliances) vary in their response between patients.
Material and methods
Experiments were conducted with approval from the University of Adelaide human ethics committee and conformed to the Declaration of Helsinki. A total of 28 adult volunteers (16 women; ages, 19-38 years) were recruited for these experiments.
All participants had natural and healthy dentitions, no dental symptoms at the time of the experiments, and no discernable craniofacial dysmorphic traits.
After their written informed consent, the subjects were prepared for the experiment. The skin over the left masseter muscle belly was prepared with a 70% alcohol swab to ensure an interelectrode impedance of less than 5 kΩ. Adhesive electromyographic (EMG) electrodes (Duotrode, Myo Tronics, Seattle, Wash) with a 2-cm interelectrode distance, were affixed to the skin over the left masseter in a superior-inferior configuration.
Grounding of participants was achieved with an ear-clip electrode. Bipolar EMG signals were amplified (1000-10,000 times), filtered (20-1000 Hz), and sampled at 2000 Hz. EMG recordings presented to participants as online feedback for the static segments of the experiment were full-wave rectified and low-pass filtered (cutoff frequency, 1 Hz). The use of feedback-controlled EMG recordings during the static segments was necessary to make sure that the background electrical activity of the muscle was matched between the static and dynamic segments of the study. Because reflex activations are known to be proportional to the level of instantaneous background excitability, matching EMG levels between conditions is an essential step to ensure that meaningful comparisons of reflexes between conditions can be made.
Each subject was seated comfortably in a dental chair adjusted for height so that the horizontal plane of the maxillary arch was aligned with the horizontal plane of the maxillary biteplate ( Fig 1 ) of the measuring apparatus. This device has been described in detail previously. The biteplates were coated with a semirigid dental impression material (Express, 3M ESPE, St Paul, Minn) molded to each participant’s teeth. The maxillary impression had a small rectangular region cut away to expose the left central incisor so that it could be contacted on the labial surface and tapped by a tooth stimulator.
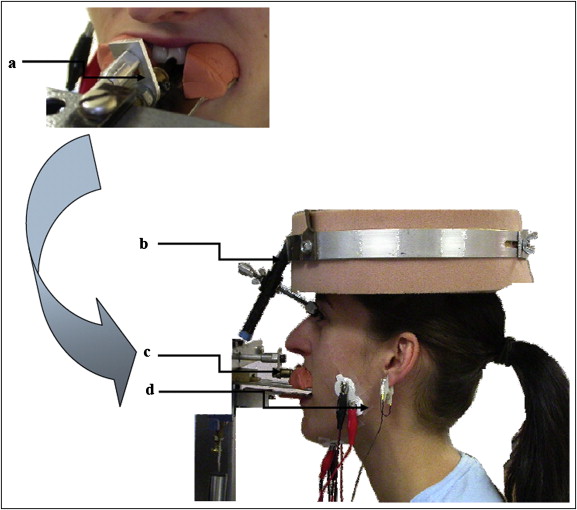
The experiments were divided into 2 main portions: static and dynamic. During static conditions, participants contracted at 2 different EMG feedback-controlled isometric levels: static closing (corresponding to the EMG evoked during jaw closing around midgape) and static opening (corresponding to the EMG evoked during jaw opening around midgape). During the isometric contraction, the dynamic lower bite bar was set in position-hold mode at the participant’s midgape jaw position. The stimulus pulse (half sinusoidal 2-N pulse with a 10 ms rise time) was superimposed on a constant tooth preload force of 0.1 N. The tooth was stimulated 50 times for each condition (static closing or static opening) with a random interstimulus interval (3-8 seconds) between stimulus pulses.
During the dynamic phase, participants chewed against a spring-like resistance provided by a servo-controlled motor. Resistance to jaw closing was inversely proportional to jaw gape: ie, minimal at maximal opening and maximal (about 60 N) at occlusion. The participants were instructed to chew at a natural pace. Two predetermined stimulus waveforms were output by a dedicated proportional integral derivative loop that controlled the tooth stimulator. These were superimposed on a constant tooth preload force (0.1 N) and consisted of either a half sinusoidal 2-N pulse or a pulse of 0 N (no stimulus) delivered in response to a trigger that occurred each time the jaw passed through the midgape point (about 14 mm measured interincisally). Two hundred stimuli were delivered during the dynamic trial: 50 pulses of 2 N during closing and opening, and 50 pulses of 0 N during closing and opening.
To ensure that only a muscle spindle response was elicited by the maxillary incisor stimulation, approximately 4 mL of local anesthetic solution (Xylocaine, Dentsply International, York, Pa) was infiltrated around the teeth, canine to canine, of both jaws. This was done to block the periodontal mechanoreceptor outflow from the incisors. All reflexes analyzed in this study were from stimulus-triggered surface EMG averages obtained during effective local anesthesia.
Rectified electromyograms were extracted around the trigger time (−50 to +75 ms). The extracted electromyograms were sorted with regard to phase of movement (closing or opening). In a similar method to that used by Hück et al, an electromyogram with stimulation was normalized by the electromyogram without stimulation to remove the trend created by the direction of movement. Briefly, the averaged rectified dynamic-with-stimulus trial was divided point by point by its respective no-stimulus analog. A 20-ms window starting 15 ms after stimulus was constructed, and the reflex area (the integral above or below the prestimulus mean) was calculated. The strength of the reflex was recorded by expressing it as a percentage of the prestimulus area between −5 and −25 ms ( Fig 2 ).
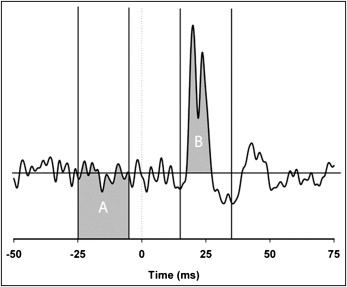
Each subject had a standard lateral cephalogram taken with a magnification factor of 1.083 by using the Nador 2 SR system (Siemens, Bensheim, Germany) with specifications of 220 V, 50 Hz, and 10 A. The focus-to-film distance was 150 cm, and midsagittal plane-to-film distance was 11.5 cm. The exposure time was 0.64 seconds with 75 kv and 20 mA.
Cephalometric analysis software systems (Dolphin, Los Angeles, Calif; and Mona Lisa, Canberra, Australia) were used to evaluate linear, angular, and proportional facial form variables on scanned lateral cephalograms ( Fig 3 ).
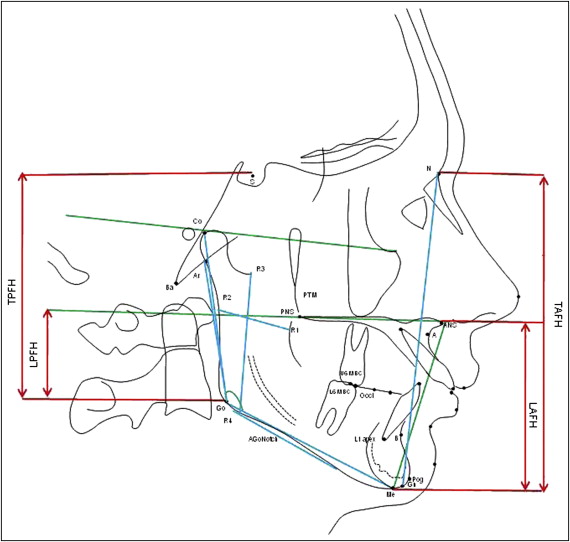
Variable | Abbreviation | Mean | SD | Range |
---|---|---|---|---|
Linear | ||||
Condylion-gonion | Co-Go | 64.3 mm | (6.0) | 55.4-79.7 |
Antegonial notch | AGo-Notch | 1.9 mm | (0.6) | 0.9-3.2 |
Articulare-gonion | Ar-Go | 45.8 mm | (5.2) | 35.3-55.4 |
Gonion-menton | Go-Me | 73.7 mm | (4.6) | 65.4-80.8 |
Nasion-gnathion | N-Gn | 107.7 mm | (6.8) | 96.9-121.3 |
Ricketts R1-R2 | R1-R2 | 29.2 mm | (3.9) | 21.7-36.2 |
Ricketts R3-R4 | R3-R4 | 48.0 mm | (6.5) | 39.6-58.0 |
Masseter length | ML | 56.3 mm | (5.6) | 44.6-65.8 |
Nasion-menton | N-Me | 110.4 mm | (7.4) | 99.2-126.6 |
Angular | ||||
Maxillary-mandibular plane | Max-Man | 21.3° | (6.2) | 10.2-36.5 |
Frankfort horizontal-mandibular plane | FH-Man | 21.8° | (6.3) | 13.2-36.1 |
Upper gonial angle | Go Angle U | 49.9° | (5.5) | 41.6-63.6 |
Lower gonial angle | Go Angle L | 68.3° | (5.2) | 60.1-82.1 |
Gonial angle | Go Angle | 118.2° | (7.8) | 102.7-134.6 |
Overbite depth indicator | ODI | 73° | (7.8) | 56.5-92.3 |
Proportional | ||||
Nasion-gnathion/articulare-gonion | NGn/ArGo | 2.2 | (0.2) | 1.8-2.7 |
Articulare-gonion/gonion-menton | ArGo/GoMe | 0.7 | (0.1) | 0.6-0.9 |
Lower anterior face height | LAFH/TAFH | 57.1% | (3.9) | 47.1-63.3 |
Lower posterior face height | LPFH/TPFH | 73.4% | (6.4) | 59.1-84.2 |
Jarabak ratio | J Ratio | 83.6% | (6.5) | 71.0-95.7 |
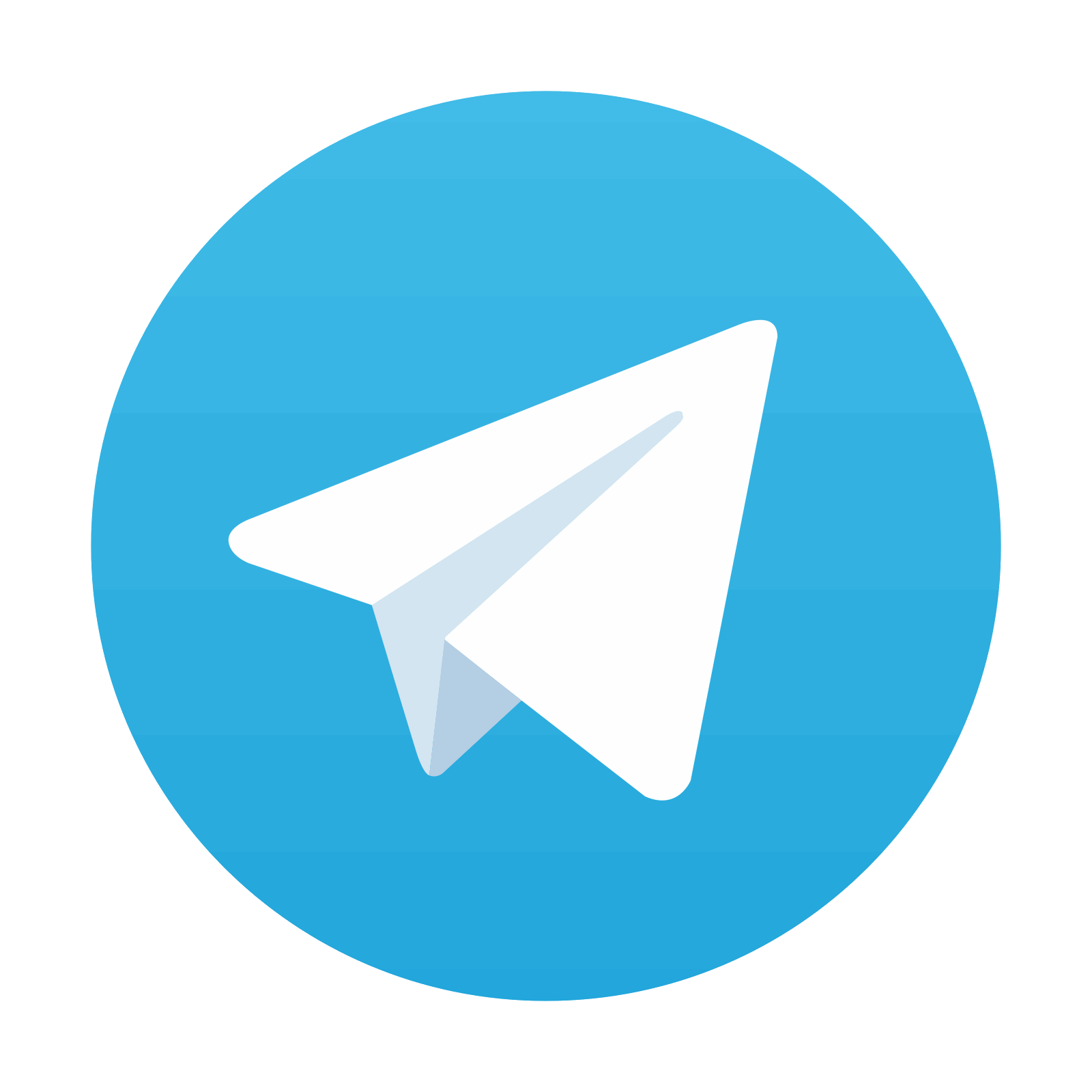
Stay updated, free dental videos. Join our Telegram channel

VIDEdental - Online dental courses
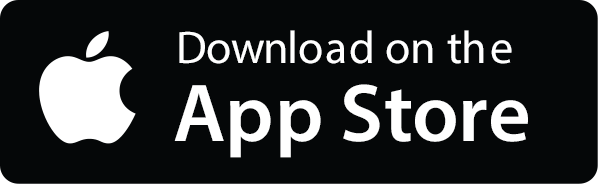
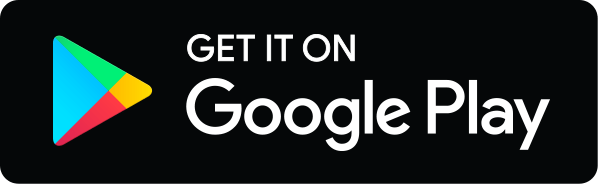