div epub:type=”chapter” role=”doc-chapter”>
S. Stübinger et al. (eds.)Lasers in Oral and Maxillofacial Surgeryhttps://doi.org/10.1007/978-3-030-29604-9_17
17. Additive Manufacturing and 3D Printing
Abstract
This chapter discusses recent applications and findings in additive manufacturing (AM), or 3D printing, applied in oral and maxillofacial surgery. The reader will get an introduction to the basics of AM technology followed by oral and maxillofacial applications like printing of anatomical models and the design and manufacturing of customised implants. Recent research on the biological response of some AM metal alloys is also discussed at the end of the chapter.
Additive manufacturing3D modellingAnatomical modelsBiological response
17.1 Introduction
Additive manufacturing (AM), or 3D printing, relates to a wide range of technologies where parts are built up by adding material in a layer-wise strategy, which is in contrast with subtractive methods where material is removed. One of the first patents in the area focused on prototyping using UV-curable polymer resins [1], and after that, new technologies were rapidly developed and a variety of materials that could be processed by AM followed. In the mid-1990s, some applications and machines for metal AM were developed, although history shows that these ideas were put on paper already in the 1970s by the French inventor Ciraud [2]. The area of AM has developed from the early days of prototyping to a technology that has begun to compete with traditional methods for serial manufacturing, and it is today seen as an important area for many countries and regions throughout the world for re-industrialisation through the increased competitiveness and sustainability offered by AM. Today, parts manufactured using AM are present in a wide range of different applications, and the technology is broad in the sense that it is applicable to everything from small scale (nanoprinting) to large scale (houses, structural elements for airplanes) and uses a large variety of materials, from food and living cells to electronics.
AM allows for freedom of design, short lead time, flexibility in manufacturing, consolidation of design, minimal material waste, and distributed engineering and manufacturing. These attributes have been shown to be important drivers for AM in general, as well as for medical applications, and AM is today used from individualised and small series manufacturing in order to meet specific needs for patients to serial production where manufacturing cost is the main driver. This chapter explains different technologies available for 3D printing, applications within oral and maxillofacial surgery, and recent findings in the biological response to such applications.
17.2 Technology Overview
Nowadays, a huge variety of 3D printing processes is available on the market. While a few years ago a 3D printer was rather unaffordable for home users, simple 3D printers can now be purchased for just a few hundred dollars (mainly FFF/fused filament fabrication). The considerable cost reduction of printers and printing materials and the increase in user-friendliness of 3D planning and printing software has led to the substantial spread of 3D printers in all areas, especially in industry, design, education, and medicine—however, industrial 3D printers can still cost hundreds of thousands of dollars. Subsequently, more and more medical professionals have become aware of the benefits of 3D printing for use in medicine. Especially in modern dentistry and oral and maxillofacial surgery, 3D printing has become an integral part of the digital medical treatment process (e.g. in the manufacture of sawing or drilling templates, dental models, orthodontic aligners, temporary restorations, or even as a basis for complex final prosthetic restorations or patient-specific implants). Many currently available 3D printing materials are biocompatible and are certified for medical applications and can therefore be used for contact with the human body or even as a substitute material for human tissues. The most widespread 3D printing processes have in common that a printing material (e.g. plastics/polymers, polymer resins, metals, ceramics, or other materials, including biological substances such as living cells) is applied layer by layer to a printing bed and cured by physical and/or chemical processes. These layers can be as thin as only a few micrometres, so the print result can be at a very high resolution and thus correspond exactly to the original computer-aided design (CAD) file. The principle is that the higher the resolution and the larger the building structure, the longer the fabrication time will be. In contrast to the already mentioned subtractive rapid prototyping procedures, which encounter significant limitations compared to the AM processes, 3D printing offers the advantage that almost all geometries can be produced. This offers the advantage that expensive raw material for printing can be saved and that very accurate, light, and resilient bodies that meet biomechanical requirements can be fabricated.
3D printing offers the advantage that engineers, developers, and physicians can segment and design 3D models on the computer and can hold them in their hands in no time at all, thanks to AM. Anatomical physical 3D models and patient-specific 3D-printed implants enable an unprecedented improvement of medical care in many areas. Above all, the fabrication of models with the exact geometry corresponding to the patient’s anatomy gives the surgeon or physician the opportunity to plan an invasive procedure in advance and to perform model operations. Taking into account the manufacturing costs of the anatomical biomodels, this leads to cost savings by improving the quality of results and shortening the operating time [3]. The physician can even test different surgical procedures on the basis of preliminary planning and choose the least invasive approach for the patient. 3D printing in medicine enables a cognitive process, which is also called “touch to comprehend” [4].
Increased interest from industry led to the need to categorise different machine technologies and processed materials, so in 2010 an ASTM initiative called “ASTM F42—Additive Manufacturing” formulated a standard where AM was divided into seven different categories—material extrusion, material jetting, binder jetting, vat photopolymerisation, powder bed fusion, sheet lamination, and directed energy deposition. Some of the most common 3D printing technologies are briefly described below.
It must be emphasised that the print result depends mainly on the quality of the digital data set, which typically originates from computed tomography (CT) or magnetic resonance imaging (MRI), and only secondarily on the printing process used. In addition, all AM processes have advantages and disadvantages that must be taken into account when selecting them for appropriate medical applications. For example, the material extrusion process is very well suited for the fabrication of anatomical 3D biomodels but less appropriate for the production of high-precision dental models or splints.
17.2.1 Material Extrusion
Due to its technical simplicity and relatively low purchase costs, material extrusion is widely used for simple 3D printers, and it includes technologies such as FFF and fused deposition modelling (FDM). With this technology, one or more plastic filaments wound on a spool are heated by one or more extruders up to the melting temperature of the material, and the material is then applied to the print bed. There, the material bonds with the layers underneath, cools, and hardens. The print bed is lowered and the next layer is applied in sub-millimetre thickness. The advantage of multi-extruder 3D printers is that different printing materials can be used at the same time. For example, support structures that are necessary for the production of overhangs can be printed with a water-soluble support material such as PVA (polyvinyl alcohol). Some research groups are currently working on FFF printing of high-performance medical polymers such as PEEK (polyether ether ketone) . Initial results are very promising and offer a wide range of applications, for example, in the cost-effective production of patient-specific PEEK implants in facial reconstructive surgery [5].
17.2.2 Vat Photopolymerisation
Vat photopolymerisation (often called stereolithography, SLA) is a process in which light (e.g. a UV laser) crosslinks molecules and cures them to solid polymers. Here, too, the building platform is raised and lowered for each cured layer in a vat of liquid polymers and exposed to the laser beam, so that the finished solid 3D model can be removed at the end of the printing process. These methods are especially suitable for very high-resolution structures and exact models. For post-processing, the 3D models often have to be washed in isopropyl alcohol and hardened by UV light to achieve their final strength and durability.
17.2.3 Powder Bed Fusion
This method includes printing processes in which granular materials (polymers, metals, etc.) are evenly distributed on the print bed with a roller and are then selectively fused layer by layer using either a laser or an electron beam. Basically, materials in powder form are melted by heat layer by layer until the model is finished. Laser-based methods include selective laser melting (SLM) and selective laser sintering (SLS), and these can be used for both polymers and metals. Electron beam melting (EBM) has been developed only for metals, and here the raw material (e.g. titanium alloy) is melted in a high vacuum by an electron beam.
17.2.4 Binder Jetting
In the binder jetting process, the powder is selectively bonded by a liquid binding agent sprayed on the powder bed using an inkjet-like technology. By using print heads with many nozzles, many dots can be cured at the same time. In this way, it is possible to fabricate solid models very quickly compared to other methods like FFF/FDM, and typical materials for this process are plastics and metals.
17.2.5 Material Jetting
Here multiple print heads deposit liquid polymers layer wise on a print bed in an inkjet-like fashion. After application of the material, it is cured with UV light. Wax-like, water-soluble, or solvent-soluble structures are used as supports for the printing polymers, and these supports can be easily removed after the printing process. These printing processes enable the processing of a wide variety of polymers with different material properties at very high precision, and some of the technologies are PolyJet and MultiJet printing.
In addition to the printing processes mentioned above, there are also a number of other processes. For the sake of simplicity, and in order not to go beyond the scope of this chapter, the mentioned technologies are discussed only in general terms. Further information can be found in the relevant technical literature.
17.3 From Medical Imaging Data to Manufacturing and Post-processing

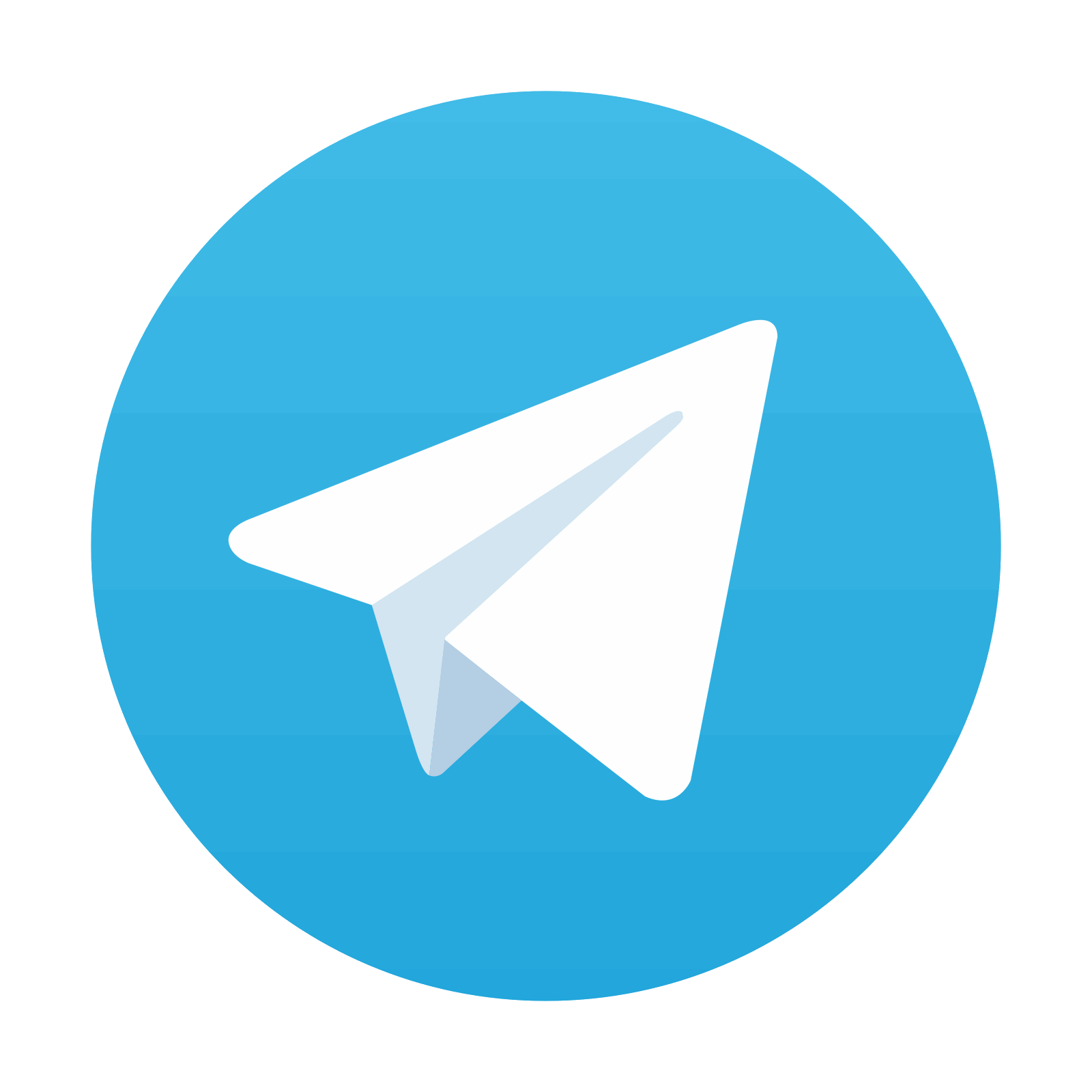
Stay updated, free dental videos. Join our Telegram channel

VIDEdental - Online dental courses
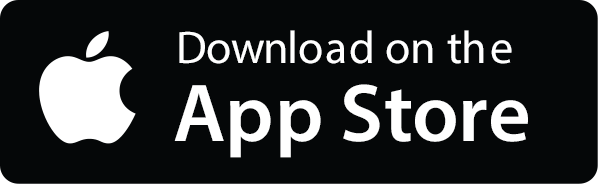
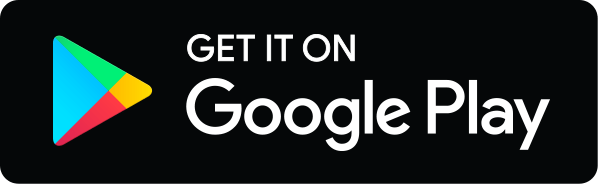