div epub:type=”chapter” role=”doc-chapter”>
S. Stübinger et al. (eds.)Lasers in Oral and Maxillofacial Surgeryhttps://doi.org/10.1007/978-3-030-29604-9_6
6. Biophotonic Based Orofacial Rehabilitation and Harmonization
Abstract
Dentistry is a field that has been evaluated to act beyond the teeth. Treatment of diseases such as gingivitis, neuralgia, and others is common nowadays. The constant demand created by the highest life expectations of men and women requires constantly changing procedures. Geriatric dentistry is, today, a specialty among the general dental activities. This constant evolution is directing dentistry to offer patients much more than the conventional curative procedures, also migrating to rehabilitation and to aesthetic restoration to promote full harmonization. Based on modern technologies, with special attention to those promoted by biophotonics, orofacial rehabilitation and harmonization is becoming a reality. In this chapter, we describe a collection of procedures and technologies that has allowed orofacial biophotonics-based rehabilitation and harmonization to become a new specialty for modern dentistry.
LaserLEDDentistryRehabilitationOrofacialBiophotonics
6.1 Introduction
Biophotonics is the use of light in the areas of health, for the purposes of the diagnosis, treatment, and maintenance of biological systems. Within dentistry, the competence of the dental surgeon is required to prevent, diagnose, treat, and preserve patients’ orofacial health. It is part of this profession to be up to date with all the innovative, scientifically established procedures.
Biophotonics, using different light sources, associated or not with other therapies (dermocosmetics, peelings, kinesiotherapies, etc.) came to add and contribute at different levels e.g., from the diagnosis of tissues to the treatment and treatment plan itself; the microbiological control of infections (e.g., caries, periodontitis, acnes, herpes), loss of sensation (paresthesias), loss of motor activity (paralyses), acute and chronic pain (photodynamic therapy); photoactivation of biomaterials (resin restorative materials and bleaching agents); selective ablation of hard and soft tissues and of biomaterials; preservation of healthy structures, and also in the management of dysfunctions, which may or may not be established in the orofacial region.
In this way, lasers and LEDs, intense pulsed light (IPL) and radiofrequency (RF), high-intensity focused ultrasound (HIFU) and high-intensity laser therapy, ablative or non-ablative, fractional or non-fractional, will also aid us in tissue and muscular toning, integumentary whitening/decontamination, and aesthetic orofacial corrections.
Considering non-invasive procedures, photobiomodulation is the area of biophotonics where light sources operating at low or medium intensity are used.
6.2 Photonic Therapies for Orofacial Rehabilitation and Harmonization
Considering the use of laser and LED light, it is possible to work from real-time optical diagnosis, with violet LEDs, performing dental lightening, with photodynamic therapy and photoactivation, laser surgery for ultra-conservative plastics and removal of carious tissue. But without doubt, the most frequently explored area that has a much broader field of action has been photobiomodulation and its combination with other therapies, such as chemical and mechanical treatments, both for the integumentary system and for the orofacial neuromuscular system.
In this way, we will review the indications of the most commonly used spectral bands and their associations with kinesiotherapies (photokinesiotherapy) and with chemical–physical treatments (photopeelings).
The intention of these therapies is to minimize the effects of aging using all the knowledge of anatomy, reconstituting the joviality without giving a plasticized, artificial aspect, with rhytidoplasty considered the gold standard.
Face squaring most often starts from the age of 30, and, depending on genetic standards and the neutralizing ability of each person’s free radicals, wrinkles may or may not arise early.
Prophylaxis for patients with high muscular strength and very expressive people prevents the early appearance of static wrinkles. For this purpose, it is possible to use photobiomodulation with light-emitting red (600–700 nm) and low total energy per application point (1 J) or infrared laser with high total energy per application point (around 5 J), associating with the functional elastic bandages (Phototaping) or even using botulinum toxin.
Orofacial rehabilitation/harmonization is, at the moment, a field of dentistry rather than a specialization. It brings together various very specific areas that intersect with each other and that seek the same goal of managing senescence and preventing the senility of the stomatognathic system.
6.2.1 Photobiomodulation
Photobiomodulation has become established as an intelligent “instrument” in managing the cellular, tissue and systemic physiological responses, in different directions, but all with the same objective: to return the synesthetic and holistic homeostasis, resulting in systemic functionality.
Phototherapy, called photobiomodulation since 2015 [1], a term that encompasses all light sources that modulate systemic physiological responses without ablating (removing) tissues, has been shown to be a very efficient option within dentistry. Relief of acute and chronic pain, drainage of inflammatory processes and tissue repair can be performed or supplemented with light at low intensity. Using higher and controlled doses and protocols, it is also able to improve the quality of the tissues under repair, avoiding keloids and apparent scars, lightened patches of skin, mucous and dental surfaces, promoting the microbiological and tumor control of regions affected by infections or uncontrolled mitoses, recovering cellular functions damaged by time.
Currently, we consider the visible spectral range and the near infrared as the lights whose chromophores in the biological tissue absorb them and, therefore, result in the promotion of a photobiomodulatory effect; however, a recently published article by Hamblin [2], also cites the possibility of light emitting in the medium infrared, where there is a considerable temperature rise, also to be absorbed, but by the water molecules and, as a consequence, increases the influx of calcium ions into the intracellular environment.
Here, we highlight some interesting photobiomodulatory characteristics of each spectral range, from violet to near infrared. According to Daghastanli [3], the lights in the visible portion have the following spectral bands: violet from 390 to 455 nm, blue from 455 to 492 nm, green from 492 to 577 nm, yellow from 577 to 597 nm, amber from 597 to 622 nm, red from 622 to 780 nm. Above 780 nm, the infrared spectrum begins, the next one considered to be 780 to 1500 nm, an average of 1500 to 5600 nm and distance of 5600 to 10,600 nm.
6.2.1.1 Red Light (622–780 nm)
Red light is absorbed by the cytochrome c-oxidase, then causing oxidation of nicotinamide adenine dinucleotide (NAD) and consequently changing the state of mitochondrial and cytoplasmic oxy-reduction. This change in the electron transport velocity of the respiratory chain generates an increase in the proton-motor force, in the electrical potential of the mitochondrial membrane, in the acidity of the cytoplasm, and in the amount of endocellular adenosine tri-phosphate (ATP).
The increase in intracellular H + ion concentration results in changes in the sodium (Na+) and potassium (K+) pump in the cell membrane, increasing the permeability to calcium ions (Ca2+) to the intracellular medium. The increased amount of this cation affects the level of the cyclic nucleotides that modulate the synthesis of RNA and DNA [4]. It changes the oxy-red state of the cell, promoting chemical and enzymatic reactions for the dissociation of internal components, immediately releasing the nitric oxide molecules previously attached to the cytochrome c-oxidase, and then accelerating the metabolism; this reflects vasodilation at the tissue level [5].
It is a spectral band that can be used in protocols for nutrition, oxygenation, and tissue repair, facilitating all other steps provided in the protocols, so that it can be associated in several treatments.
In addition, we know that for the light to be absorbed by cytochrome c-oxidase, phototoxication of nitric oxide occurs, which is responsible for the immediate increase in vasodilation and improvement in tissue oxygenation.
In addition, in an editorial of Photomedicine and Laser Surgery, Sommer and Trelles [6] developed the following idea: the near infrared light (670 nm—red) at low intensities for biostimulation is fundamental to regulating the ATP content in cells, and when applied in a pulsed mode, to the activation of cellular metabolism.
Thus, red light can promote the treatment of vitiligo—in very low doses 3 J/cm2 – reorganization of fibroblasts, melanocytes, keratinocytes, and melanoblasts ([7, 8]; be antioxidant [9, 10]; decrease the expression of metalloproteinases; and promote an “FPS 15-like” effect by preventing post-inflammatory hyperpigmentation by increasing nerve growth factor (NGF), protecting melanocytes from UVB [11].
Moshkovska and Mayberry [9] and Huang et al. [12] already mentioned the benefits of intravascular therapy with red laser, also being an antioxidant and systemically treating some diseases.
Red laser is low intensity, because it penetrates less, mainly by the mechanism of absorption through which it interacts with the biological tissue is indicated for superficial lesions such as tissue repair (healing and local drainage), muscle relaxation, or to photoactivate biomaterials/dermocosmetics for topical application.
Red light (laser or LED) at low intensity also promotes mitochondrial biogenesis, that is, it restores the conditions for the cells to rebalance their metabolism. Recent work shows that 630 nm (37 mW/cm2, 75 mW) LED irradiation with 16 J/cm2 radiant exposure was able to induce a proliferative response in stem cells of the pulp tissue of deciduous teeth without affecting mitochondrial function or inducing senescence [13].
A recent study by Oh et al. [14] showed that the LED, emitting in the range of 660 nm, under an energy density of 10–20 J/cm2, was able to inhibit tyrosinase activity, reducing UVB-induced melanogenesis in the skin (in vitro and in vivo). They concluded that the red LED ± 660 nm is a potential integumentary depigmentation.
6.2.1.2 Near Infrared Light (780–1500 nm)
Light emitting in the spectral range of the near infrared can be absorbed by the cytochrome c-oxidase in the mitochondrial respiratory chain, too. However, it can also be absorbed in the biomembranes, the photophysical polarity of the membranes that results in the alteration of the conduction of neural stimuli and the permeability of these biomembranes.
Cytoplasmic membrane permeability increases in relation to the Ca2+, Na+ and K+ ions, determining an increase in cell membrane receptor activity. As a result, endorphin synthesis and the action potential of neural cells increase, whereas the amount of bradykinin as well as the activity of C-fibers leading to painful stimuli diminishes. This sequence of events results in relief of pain symptoms [4]. Infrared laser/LED are wavelengths that act in the relief of acute and chronic pain, lymphatic drainage, and bone and neural repair, being considered, in aesthetic treatment, essential when the cause is the accumulation of free radicals in the system. Considering the integumentary system, it is hypothesized that, once this spectral band is absorbed by the membrane proteins, it can then be absorbed by the aquaporins, for example, thus stimulating its action, which is the transport of transmembrane water molecules (from the dermis to the epidermis). This could promote active epidermal hydration.
The infrared laser in low intensity is more penetrating, but because it interacts through changes in the polarity of the biomembranes, it has been the wavelength of choice for neural and bone repair, and also to promote immediate and temporary analgesia, as it works by altering the potential of these biomembranes.
As with red light, it can also be delivered in the target tissue at the dental clinic, both in a timely manner and in a larger area, when the idea of concentrating the delivered light penetrates a little more and the diffusion in the cluster generates the opposite effect, keeping the greater percentage of the energy absorbed in the more superficial layers.
Several studies with infrared, laser, and/or LED light, associated with exercises and other kinesiotherapies, have demonstrated a great ability to accelerate neuro-muscular rehabilitation, both facially and in the body. This possibility opens a very important window for orofacial rehabilitation procedures, as it would not only be suitable for reconditioning the system to perform its functions, but also to prevent the loss of efficiency that is physiologically occurring with the aging of the stomatognathic system. This approach will be discussed in Sect. 6.2.2 on photokinesiotherapy.
6.2.1.3 Blue Light (455–492 nm)
Blue light is able to accelerate chemical reactions, including cell metabolism. Absorbed by the flavoproteins [15], in the mitochondrial respiratory chain, hemoglobin, porphyrins, and cytochrome c were reduced [16], but also absorbed by cryptochromes [2], in biological tissues, and by most biomaterials because of their spectral range.
Upon absorption by flavoproteins and cryptochromes, the blue light promotes an increase in the influx of some ions, such as calcium, magnesium, and zinc, improving the cellular metabolism.
It can be used as a photoactivator and photoaccelerates polymerization of monomers in restorative materials and to induce the production of singlet oxygen in photodynamic therapies, when the photosensitizer and/or the target bacterium (as for example, the bacterium of acne, Propionibacterium acnes [17] and the bacteria of periodontal disease) absorb blue wavelengths [18, 19].
However, even within photobiomodulation, it can decompose hydrogen peroxide present in cells (metabolism products) [18] and/or biomaterial (dental bleaching agents). The action, when absorbed by the peroxides, results in the breaking of large light-absorbing molecules and of these peroxides, transforming double bonds into simple ones, reducing the absorption of light, and then there will be whitening of the skin and dental tissue (enamel and dentin) and hydration of the tissues [20, 21].
In addition to this mechanism, through controlled thermotherapy, when the source emits with medium intensity, a facilitation occurs in the decomposition of the peroxides and diffusion of the products to the interstitial space, in the soft tissues, inducing swelling and acting as a hydrating agent by wetting, promoting the change in the superficial tension of the skin, with the aesthetic effect of expansion of the tissues.
In addition, once absorbed, blue light may induce the production of collagen and elastin, being able to accelerate cellular metabolism as much as or more than the red wavelength [22].
Another hypothesis on tegument hydration (skin = epidermis, dermis, and cutaneous attachments) is that the blue spectrum accelerates the function of keratin in making the stratum corneum more resistant and impermeable, acting as an occlusion agent for the epidermis, reducing loss (reducing transepidermal water loss).
A more recently discovered function: low doses (3–16 J/cm2) are able to modulate immature and mature Langerhans cells (dendritic cells), modulating proinflammatory cytokines locally and in lymph nodes [23].
6.2.1.4 Amber Light (577–622 nm)
Amber light emits into a spectral range of 577–622 nm. It has the best peak of absorption by cytochrome c oxidase, according to Poyton and Ball [24]. Thus, as well as wavelengths emitting red (600–700 nm), it interferes with mitochondrial respiration by accelerating the rate of endocellular ATP production and promotes a large release of nitric oxide (NO), which is responsible for vasodilation and neurotransmission.
It is therefore recommended to accelerate the repair of soft tissues of the tegument (gingiva, epidermis, and dermis) and the conjunctiva, such as assisting the return of muscle toning in a case of facial paralysis during the neuromuscular rehabilitation process.
Regarding its penetrability into the biological tissue, it is already known that amber light reaches the connective tissue, i.e., in the skin, it reaches the papillary dermis. Figure shows a measure where this situation is very clear. However, orofacial tissues, because they are very thin, may have the benefit of this light in deeper layers, reaching muscle tissue.
WEISS et al. [25, 26] presented an experience of more than 2 years treating 900 patients with dermatological diseases, using amber LED (±590 nm) as the only instrument or associated with other therapies (Light Intense Pulsed (LIP), RF, ablative lasers, for example). They observed the following clinical effects of this amber light: an anti-inflammatory effect, acceleration in tissue repair, decreased gene expression of collagenase in photodark skins, and thus a suitable light for skin aging management treatments as well as post-treatment of more aggressive surgical procedures [27, 28].
Another study by the same group [25, 26] confirmed with standardized experiments that the photomodulation treatment has the potential to modulate the production of matrix metalloproteinases (MMPs) after thermal lesions. Therefore, combined amber LED treatments with non-ablative thermal techniques, such as ILP or dye laser, may be beneficial.
To McDaniel (2005), the patent was granted to manufacture all the equipment with amber LED, whether alone or associated with the infrared laser, in the continuous (CW), periodic (keyed) or pulsed modes. He presented the methods and how to use them to regulate cell proliferation and gene expression in the production of collagen.
A recently published article by Chen et al. [29] demonstrated that amber light at 585 nm with fluences of 10–20 J/cm2 was able to induce autophagy of melanocytes and decrease melanogenesis, confining their indication to tissue whitening.
6.2.1.5 Violet Light (390–455 nm)
In this emission range, the photons have more energy; therefore, being highly absorbed, the penetrability of this light into the biological tissues is quite superficial, remaining in the epithelial band of the mucous membranes and in the epidermis of the skin. In this way, fluorescence can also be easily observed and seen with the naked eye or with filters; thus, it is a welcome spectral range for both optical diagnostics and surface treatments.
Absorption chromophores consist of derivatives of porphyrins, flavoproteins, and terminal oxidases; oxyhemoglobin and melanin; and long chain molecules with delocalized electrons [30].
In addition, it is important to note that the effects of nitric oxide (NO) and NO molecules on the surface of the lungs have been shown to be associated with the effects of vasoconstriction and decontamination [31], and with the use of a high-performance tooth-whitening agent (Lever et al., 2010).
Some studies have shown that violet light is more efficient than blue light for decontamination of skin, mucosa, and even osseo-integrated implants [32–34], and is very well indicated as a photonic agent for facial peelings [35], promoting excellent clinical results by softening facial expression marks and wrinkles.
However, it is a spectral band that can generate many free radicals that are deleterious to important molecules, such as carotenoids (natural skin antioxidants) and fibroblasts present in the dermis [36]; the dose and frequency of application should be monitored. It is worth remembering here the importance of dosimetry in the clinical success of photobiomodulation.
6.2.1.6 Green Light (492–577 nm)
There are still few studies on green light. According to HAMBLIN [2], green light is absorbed by the opsins. One of the best defined signaling events that occurs after opiate light activation is the opening of light ion channels, such as members of the calcium channel family of transient receptor potential (TRP). TRP channels are now known as pleiotropic cellular sensors that measure the response to a wide range of external stimuli (heat, cold, pressure, taste, smell) and are involved in various cellular processes.
-
Antioxidant effect on photoaged skin [37].
-
Increased angiogenesis [38].
-
Acceleration in the process of repair in burned skin [39].
-
Anti-inflammatory effect on burned skin [40].
-
Acceleration in the cellular metabolism (increase in the concentration of calcium ions) and an increase in the gene expression for osteogenesis, from the stem cells [41].
-
Excessive increase in the entry of calcium ions (oxidation), greatly increasing free radicals and inhibiting the proliferation of adipocytes (from the stem cells) [42].
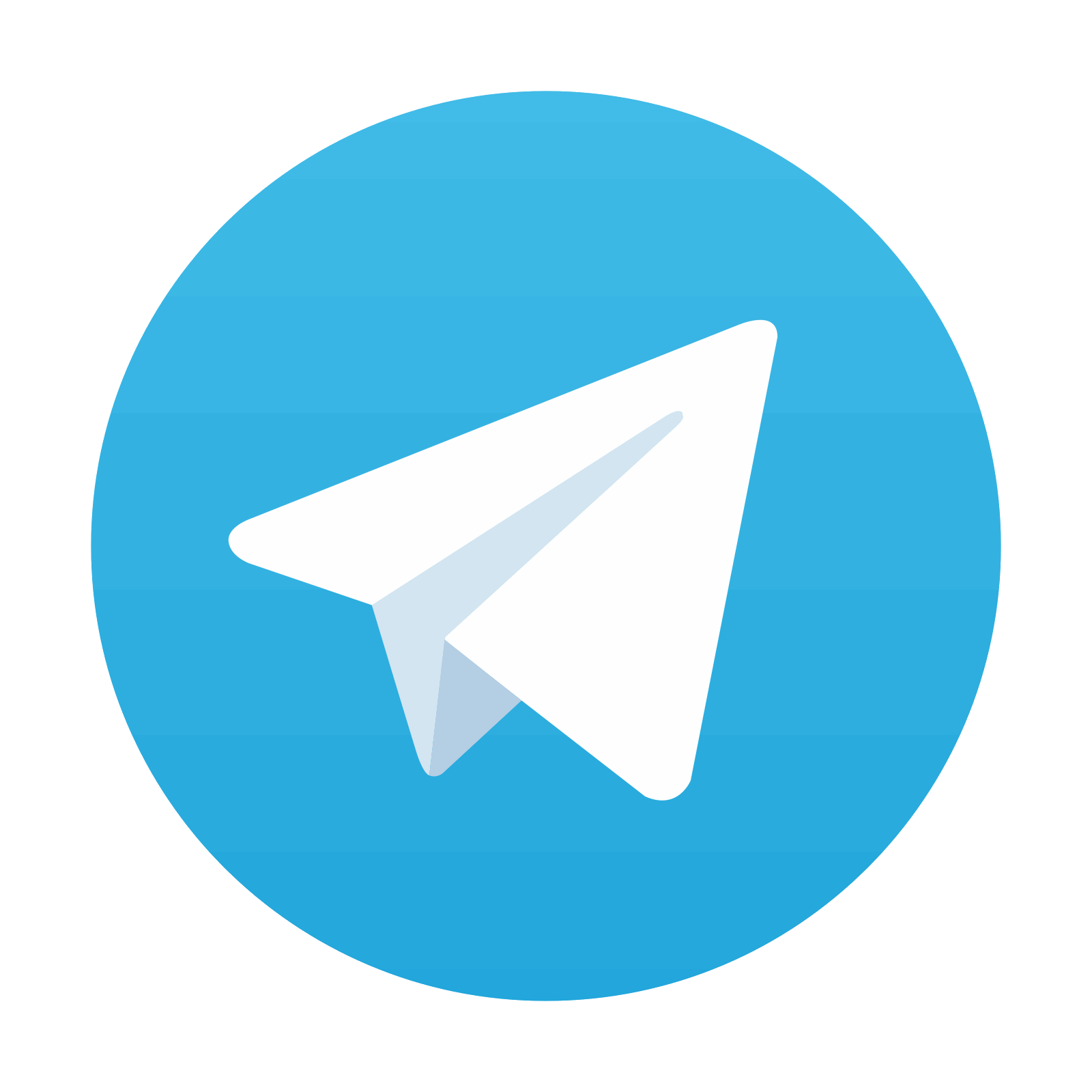
Stay updated, free dental videos. Join our Telegram channel

VIDEdental - Online dental courses
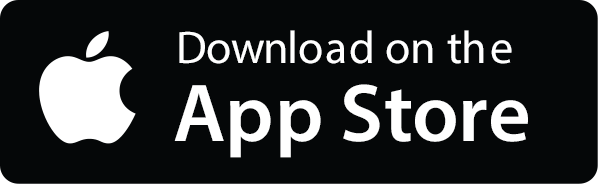
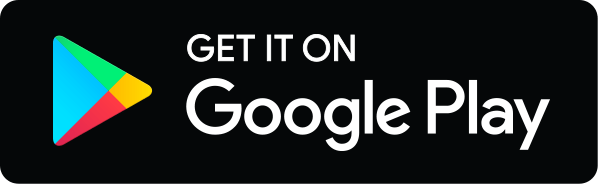