In the area of craniomaxillofacial trauma, neurosensory disturbances are encountered commonly, especially with regard to the trigeminal and facial nerve systems. This article reviews the specific microanatomy of both cranial nerves V and VII, and evaluates contemporary neurosensory testing, current imaging modalities, and available nerve injury classification systems. In addition, the article proposes treatment paradigms for management of trigeminal and facial nerve injuries, specifically with regard to the craniomaxillofacial trauma setting.
Key points
- •
The inferior alveolar nerve, lingual nerve, infraorbital nerve, as well as other branches of the trigeminal nerve are at risk for injury as a result of craniomaxillofacial trauma.
- •
The facial nerve can be categorized into 3 distinct segments: the intracranial, intratemporal, and extratemporal segments.
- •
The Seddon classification, Sunderland classification, and Medical Research Council Scale are all ways to classify sensory nerve injuries.
- •
The House-Brackmann scale, or variants of this scale, is the preferred scale for evaluating and monitoring facial nerve injuries.
Introduction
Injury to the fifth (V, trigeminal) and seventh (VII, facial) cranial nerves may result in significant cosmetic and functional deficits. These deformities have a profound effect on quality of life. The head and neck region is rich in neural and vascular networks, and the density of peripheral nerve receptors in this area makes neurosensory disturbance more noticeable than in other parts of the body (eg, trunk and extremities). The trigeminal and facial nerves are the most common cranial nerves affected in maxillofacial trauma. Ultimately, the structures that they innervate are involved in nearly all cases of facial trauma. Unlike with other mechanisms of injury, diagnosis of cranial nerve injury in maxillofacial trauma can be challenging because of the associated patient-oriented factors and the setting in which they are assessed. However, as in the nontrauma setting, expeditious management is critical to the best chance of neurosensory recovery. Injury to the trigeminal nerve can have a profound effect on speech and swallowing, taste, and mastication. Likewise, injury to the facial nerve may have deleterious effects on function and social interaction because it not only innervates the muscles of mastication but provides parasympathetic stimulation to the lacrimal, sublingual, and submandibular glands. This article reviews the diagnosis and management of trigeminal and facial nerve injuries in the setting of craniomaxillofacial trauma. Standard use of terminology between clinicians is critical when managing peripheral nerve injuries. Commonly used to terms to describe neurosensory disturbances are listed in Table 1 .
Term | Definition |
---|---|
Allodynia | Pain because of a nonpainful stimulus |
Analgesia | Absence of pain from a painful stimulation |
Anesthesia | Absence of any sensation from a painful or nonpainful stimulation |
Dysesthesia | Abnormal sensation, spontaneous or evoked, that is unpleasant |
Hyperalgesia | Abnormal response to a painful stimulus |
Hyperesthesia | Increased sensitivity to stimulation, excludes special senses |
Hyperpathia | Increased reaction to a (repetitive) stimulus |
Hypoalgesia | Diminished pain in response to a painful stimulus |
Hypoesthesia | Decreased sensitivity to stimulation; excludes special senses |
Neuralgia | Pain in the distribution of a nerve or nerves |
Paresthesia | An abnormal sensation, spontaneous or evoked, that is not unpleasant |
Trigeminal nerve
Macroanatomy
The trigeminal nerve is the largest of the cranial nerves. It has 3 divisions: the ophthalmic (V1), maxillary (V2), and mandibular (V3). The trigeminal nerve is unique in that it contains sensory (general afferent) and mixed motor (proprioceptive and special efferent) components. The third division of trigeminal nerve has 2 main branches: the inferior alveolar nerve (IAN) and the lingual nerve. This article does not discuss in detail injuries to the first division of the trigeminal nerve or other less commonly injured nerves, such as the buccal nerve or nerve to the mylohyoid muscle.
Inferior Alveolar Nerve
The mandibular division (V3) of the trigeminal nerve has 3 branches, with the IAN being the largest ( Fig. 1 ). The third division of the trigeminal nerve exits the skull base through the foramen ovale of the greater wing of the sphenoid bone (see Fig. 1 ). It descends lateral to the medial pterygoid muscle and medial to the mandibular forearm. It enters the mandible through the mandibular foramen and then travels through the body of the mandible and exits the mental foramen. The IAN is most lateral and closest to the buccal cortex at the third molar region of the mandible. It then changes trajectory at the first molar region where it neighbors the lingual plate. At the area of the premolars, the IAN again travels adjacent to the buccal cortex before exiting the mental foramen. Depending on race, the mental foramen may be located between the premolars (white people) or distal to the second molar (black people). The superior extent of the lingual nerve is at the lingula, 100 mm from the sigmoid notch. It reaches its most caudal aspect at the region between the first molar and second premolar. At this landmark, its splits into the incisive and mental nerve branches and it is 7.5 mm (±1 mm) from the inferior border of the mandible. The IAN provides sensory innervation to the mandibular teeth.

The mental nerve is a continuation of the IAN as it exits the mental foramen (see Fig. 1 ) and is frequently at risk for injury with parasymphysis and body fractures of the mandible. It has 2 main branches; however, up to 4 subdivisions can be seen (angular, medial inferior labial, lateral inferior labial, and mental). The orbicularis oris branch, the largest branch, runs just beneath the mucosa and superficial to the orbicularis oris muscle. This branch can easily be injured on a transoral approach to the mandibular body or symphysis. Care should be taken to dissect this nerve during the approach to the mandible (see Fig. 1 ). The labial branch innervates the mucosa of the lip and chin. Another smaller mucosal branch travels posteriorly to innervate the buccinator muscle.
Lingual Nerve
The lingual nerve emanates from the posterior division of the third division of the trigeminal nerve. It travels medial to the lateral pterygoid muscle on a course medial to the IAN (see Fig. 1 A). It then travels between the ramus and medial pterygoid muscle within mesioneural fat. The lingual nerve then enters the oral cavity as it travels over the superior pharyngeal constrictor, styloglossus, and mylohyoid muscles. The nerve then travels from lateral to medial at the level of the third molar position. It provides sensory and special sensory function to the anterior two-thirds of the ipsilateral tongue, floor of mouth, and lingual mandibular gingiva. The lingual nerve merges with the chorda tympani branch of the facial nerve as it travels through the petrotympanic fissure. This nerve provides taste to the anterior two-thirds of the tongue and carries special visceral afferents, including preganglionic parasympathetic fibers to the submandibular and sublingual glands. At the level of the sigmoid notch there are 4 branching patterns of the IAN and lingual nerve : separation above the sigmoid notch and inferior to the otic ganglion (65.6%), separation below the notch and at the superior half of the distance between the notch and ganglia (28%), separation below the notch and at the inferior half of the distance between the notch and lingula (3.1%), and separation in a plexiform pattern (6.3%). The bifurcation occurs at a mean distance of 14.3 mm (7.8–24.1 mm) inferior to the foramen ovale and 16.5 mm superior to the tipoff the hamulus (4.9–24.3 mm). There are collateral branches to the retromolar trigone in 81% of cases owing to the commonly reported failure rate of mandibular anesthesia.
In the area of the third molar, the lingual nerve can be found superior to the lingual crest in 10% of people, and in direct contact with the lingual plate in 25%. At the third molar region, the lingual nerve has a diameter between 2 and 5 mm. The shape varies between being round, oval, kidney shaped, elliptical, or ribbonlike in cross section; however, the predominant shape varies between studies. The exact location of the lingual nerve in the third molar region varies between studies ( Table 2 ). When adjacent to the second molar, the lingual nerve crosses the Wharton duct from lateral to medial traveling below the duct and hypoglossal nerve in the submandibular triangle. In this region, that the lingual nerve gives off preganglionic parasympathetic fibers from the facial nerve to the submandibular ganglion. The lingual nerve may be damaged on excision of the submandibular gland in up to 1.4% to 4.8% of patients. After looping under the Wharton duct, the lingual nerve travels cephalad and enters the genioglossus muscle and tongue substance. One to 2 branches travel to the tip of the tongue. Other branches travel to the lingual mucosa and gingiva and are of small diameter (1–2 mm).
Study | Nerves (N) | Vertical Measurement (mm) | Horizontal Measurement (mm) | Lingual Nerve Above Alveolar Crest (%) | Lingual Nerve in Contact With Lingual Plate (%) |
---|---|---|---|---|---|
Kiesselbach & Chamberlain, 1984 | 34 cadaveric and 256 in vivo nerves | 2.28 ± 1.96 | 0.58 ± 0.9 | 17.6 | 62 |
Pogrel et al, 1995 | 40 cadaveric nerves | 8.32 ± 4.05 | 3.45 ± 1.48 | 15 | 0 |
Behnia et al, 2000 | 669 cadaveric nerves | 3.01 ± 0.42 | 2.06 ± 1.10 | 14.05 | 23.27 |
Holzle & Wollfe, 2011 | 68 cadaveric nerves | 7.83 ± 1.65 | 0.86 ± 1.00 | 8.82 | 57.4 |
Karakas et al, 2007 | 21 cadaveric nerves | 9.56 ± 5.28 | 4.19 ± 1.99 | 4.7 | 0 |
Miloro et al, 1997 | 20 in vivo nerves (evaluated by high-resolution MRI) | 2.75 ± 1.00 | 2.53 ± 0.67 | 10 | 25 |
Infraorbital Nerve
The infraorbital nerve (ION) is a terminal branch of the second division of the trigeminal nerve and becomes extracranial at the foramen rotundum. It emerges through the skeleton onto the face at the infraorbital foramen. The mean distance of the infraorbital foramen from the facial midline and inferior orbital rim is 2.7 cm and 6 to 8 mm respectively. , There is usually 1 canal (90%), with 2 canals (5%) or 3 canals being present (5%) in fewer cases. The shape of the canal may be oval (30%), round (40%), or semilunar (30%). The infraorbital nerve exits the infraorbital foramen to divide into 4 branches, supplying the midface, nose, upper lip, and lower eyelid.
The ION enters the orbit via the inferior orbital fissure and travels posteriorly. The canal may not be complete, and a groove rather than a canal is seen in 50% of cases. The ION is at risk during periorbital approaches and during fractures involving the midface or neighboring structures.
Microanatomy
The microanatomy of all peripheral nerves, sensory or motor, is similar, with a ratio of myelinated to unmyelinated fibers of 1:4. Sensory and motor peripheral nerves differ in the number of Schwann cells surrounding each fiber with nodes of Ranvier that permit saltatory nerve conduction ( Fig. 2 ).The membrane, or basal lamina, created by the Schwann cell wraps around the axon and runs the entire length of the axon. This structure is often called the band of bungner (laminar myelin sheath) and is crucial in the process of nerve regeneration. Although myelin may be destroyed during injury, the Schwann cells often survive. This process plays a supportive role in nerve repair and regeneration. The internode is the length of axon surrounded by a single Schwann cell. The small area (0.3 μm to 2.0 μm) between the internodes where the axon is not myelinated is known as the node of Ranvier. The nodes of Ranvier allow the diffusion of certain ions, causing nerve depolarization and repolarization. This process allows conduction of nerve impulses along the nerve fiber. It is this conduction between nodes of Ranvier (saltatory conduction) that is responsible for the rapid transmission of nerve impulses in myelinated nerves (up to 150 m/s) compared with nonmyelinated nerve fibers (2–2.5 msec).

In general, unmyelinated axons are smaller in diameter than myelinated nerves (0.15–2.0 μm). Collagen creates the surrounding framework of the nerve and contributes to the structural architecture of the nerve. The endoneurium is the collagen covering surrounding each nerve fiber axon ( Fig. 3 ). , Several of endoneurial groups, which are known as fascicles, are grouped together and surrounded by a second layer of collagen cells known as perineurium. The outermost layer supporting the nerve along with some elastic fibers is known as the epineurium (extrafascicular epineurium). The internal epineurium is another layer of epineurium that invests the fascicles. The epineurium consists of 50% of the cross-sectional area of the nerve. It provides a layer of protection again compression and other types of trauma. The final and outermost layer of the nerve, containing connective tissue and continuous with the epineurium and surrounding tissue, is the mesoneurium, or adventitia of the nerve.

Peripheral nerves are often classified based on their fascicular pattern, including monofascicular, oligofascicular, and polyfascicular. Monofascicular nerves, such as the chorda tympani, have only 1 large fascicle. Oligofascicular nerves, such as the labial branch of the mental nerve, contain 2 to 10 fascicles. Polyfascicular nerves, such as inferior alveolar or lingual nerves, contain more than 10 fascicles. As cranial nerves travel from proximal to distal, in general the number of fascicles and cross-sectional area decrease. Table 3 reports the number of fascicles and diameter of common branches of the trigeminal nerve.
Nerve | No. of Fascicles (Mean) | Mean Cross-sectional Area (mm 2 ) |
---|---|---|
IAN | 9.4–21 ± 7.05 (third molar region) | 1.64 ± 0.27 (third molar region) |
LN | 8.5–10 ± 4.0 (third molar region) | 1.87 ± 0.38 (third molar region) |
MN | 10.9–12 ± 3.58 | 1.18 ± 0.27 |
ION | 5–6 (in the IOC) | 2 (in the IOC) 0.4 (after it exits the IOF) |
The blood supply of a nerve is usually provided by an associated artery and vein. Nerve fascicles have their own independent blood supplies, which is similar to individual nerve fibers ( Fig. 4 ). , The vasculature passes between the layers of connective tissue within the intrafascicular layers. The vessels travel to the extrafascicular epineurium via the vasa nervosum, running in a longitudinally oriented plexus.

Epidemiology
Inevitably, all structures innervated by cranial nerves V and VII are involved in patients with oral and maxillofacial trauma. The structures that develop from the first and second branchial arches receive their innervation via the second and third divisions of the trigeminal nerve and facial nerve respectively. Therefore, the second and, more commonly, third divisions of the trigeminal nerve and any branch of the facial nerve may be damaged when trauma involves nearby structures. The facial nerve is affected in 48% of ballistic injuries to the temporal bone. Transection of the facial nerve may occur when tethered in the temporal bone or by tearing or crushing the nerve on exiting the temporal bone.
Diagnosis
Trigeminal nerve imaging
Radiologic examination of the trigeminal nerve for injury should be guided by clinical examination. Imaging of cranial nerves, especially that their distal aspects, has not made the same progress in recent decades as imaging of other bodily structures. Imaging of the trigeminal nerve should be categorized with regard to timing (more specifically, the preinjury, postinjury, and postrepair phases). A fourth phase of intraoperative monitoring may be used as well (ie, witnessing a nerve injury or monitoring nerve conduction). The postinjury phase can be divided into the primary phase (immediately after injury) and secondary phase (ie, after nerve injury and repair).
Preoperative phase
In the trauma setting, the preoperative assessment of the trigeminal nerve can only be done by obtaining imaging taken before nerve injury. Often this is not available. However, the surgeon should make attempts to acquire imaging (plain films, computed tomography [CT], MRI, and so forth). Evaluation of the preinjury status in the trigeminal nerve can include a panoramic radiograph, and plain films or CT scans of the head, face, and neck. Any disruption seen in the postinjury phase, not present on the preinjury panoramic radiograph, can help guide the surgeon to an area of needed exploration and possible repair.
CT bone windows are useful for assessing disruption of the cortical pathway of any neural canal, including the IAN canal. However, bone windows of CT are poor at visualizing neurovascular strictures and therefore cannot give any information about the structural integrity of the nerve itself. Soft tissue windows also give poor resolution for viewing neurovascular structures. In addition, dental artifacts, often present on CT scans of the head and neck, can make visualization of soft tissue structures challenging. Cone beam CT (CBCT) technology is an alternative to traditional CT for imaging of maxillofacial structures, but does not offer any additional detail compared with traditional CT, and it may offer less reliable data for neurovascular structures. Although both CBCT and CT can offer excellent visualization of the inferior alveolar canal or infraorbital foramen, both entities are poor at visualizing nerves contained within soft tissues, such as the lingual nerve or buccal nerve. If possible, CBCT should be used as an alternative to CT because it follows the rule: as low as reasonably achievable.
MRI is the optimal imaging for visualizing the nerve itself. Each segment of the nerve and the surrounding soft tissue structures around the nerve can be visualized in detail with MRI. Various settings, including coil selection, in-plane resolution, and use of other techniques, can be specified for visualization of the cranial nerves. Contrast-enhanced T1-weighted (T1W) or T1W three-dimensional fast filled echo imaging can be used to trace the third division of the trigeminal nerve as it exits the foramen ovale. It can then be traced further as it divides into the inferior alveolar and lingual nerves. MRI is rarely available to identify cranial nerves in the preoperative setting. Mostly it is used to identify disorder or injury. Ultrasonography is mainly used to assess peripheral nerve lesions. Although the advantage of ultrasonography is that it is readily available and inexpensive, because of the conspicuous nature, its use to assess the trigeminal nerve is limited.
Postinjury phase
Panoramic radiograph is critical in assessing the postinjury phase in injuries to the lingual nerve and IAN. Cortical disruption and radiopaque foreign bodies can often be seen. Gross disruption of the inferior alveolar canal in the setting of mandible fracture may show an area of disruption and predict neurosensory changes.
Postinjury CT or CBCT may provide a more precise location of injury than panoramic radiography. Disruption along any aspect of the bony inferior alveolar nerve canal can be seen and indicates IAN injury. Disruption of the lingual cortex of the mandible in the third molar region caused by traumatic bony encroachment, or the presence of foreign bodies, along the perceived path of the lingual nerve may indicate injury.
Magnetic source imaging (MSI) is an entity that combines magnetoencephalography (MEG) and high-resolution MRI (HR-MRI). MEG works similarly to somatosensory evoked potentials, where a peripheral stimulus is applied and a central signal is recorded. This technique allows the measurement of both latency and amplitude of response. This information is then combined with HR-MRI to produce a functional and structural source image (MSI) of a region of the brain. MSI has been shown to differentiate different grades of IAN injury.
HR-MRI is still a technology in its infancy. HR-MRI can possibly be used to identify prognosis of neurosensory return in patients with nerve injury caused by trauma. HR-MRI also provides a more precise view of change in nerve position, including retraction of the nerve and neuroma formation in the area of injury. HR-MRI may also be able to show a change in nerve shape, which can lead to the diagnosis of neuroma formation. HR-MRI may also be useful in monitoring the progression of anatomic neurosensory recovery following nerve injury and/or microneurosurgical repair, and this is an area of further study.
Perhaps the most exciting imaging modality to assess nerve injury is magnetic resonance neurography (MRN). Similar to magnetic resonance angiography, which focuses the application of MRI technology to the blood vessels, MRN is direct imaging of neural structures. MRN images are obtained using axial, coronal, and longitudinal T 1 and T 2 image acquisition with customized phased array coils and imaging protocols. MRN is unique in that it can differentiate between surrounding soft tissue structures such as blood vessels, adipose tissue, and lymph nodes. Most research on MRN has focused on large peripheral nerves. , MRN can detect increased diameter of injured nerves, increase signal intensity, and longitudinal variations associated with nerve injury and recovery. Hyperintensity on MRN has not been correlated with degree of nerve injury. Signal hyperintensity has been shown after neurorrhaphy. MRN can depict fascicular architecture based on a difference in fluid composition of the neural elements. The advantage of MRN compared with other imaging techniques is that it is able to correlate imaging findings with histologic characteristics of different grades of nerve injuries as classified by Seddon and Sunderland.
Classification of trigeminal injuries
The 2 most common nerve injury classification schemes are the Seddon and Sunderland. The Seddon classification is based on time between injury and recovery and degree of recovery. Sunderland emphasizes specific histologic degree or damaged neural structures.
Seddon Classification
The Seddon classification is a simple system that classifies nerve injuries into 3 levels of injury ( Fig. 5 ): neurapraxia, axonotmesis, and neurotmesis. The integrity of the axon is maintained in neuropraxic injuries. In these types of injuries, there is a local conduction block from a transient anoxic event caused by acute disruption of the epineurial or endoneurial vasculature. Neuropraxia is often a result of minor nerve manipulation, compression, or traction injury. This condition is characterized by a reversible conduction block with a favorable outcome with rapid and complete recovery within a few weeks of onset. There is no axonal degeneration in neuropraxic injuries, with damaged confined to the endoneurium only. However, axonotmesis is characterized by axonal damage and corresponds to Sunderland II to IV injuries. There is a variable degree of demyelination and axonal injury, which is why spontaneous recovery varies by category of injury. Neurotmesis is near-compete or complete transection with epineural discontinuity. Spontaneous recovery is unlikely and neuroma formation may occur.

Sunderland Classification
In 1951, Sunderland revised and further subclassified nerve injuries into a 5-tiered system ( Table 4 ). This system was based on histologic findings and degree of nerve involvement ( Fig. 6 ). A first-degree Sunderland injury is similar to Seddon neuropraxia. This classification was further divided by Sunderland into 3 types based on the severity and recovery time. A first-degree type 1 injury is the result of nerve manipulation that causes transient blood supply interruption. Once this is restored, it allows full sensory recovery after only a few hours. A first-degree type II injury involves intrafascicular edema formation from exudate or transudate. This condition usually results from moderate traction or compression injury to the nerve. Neurosensory recovery occurs after the edema resolves in the first few days. First-degree type III is characterized by intrafascicular edema formation resulting from transudate and exudate and results from moderate traction, or compression on the nerve. Recovery of sensation is usually correlated with resolution of edema (several days after injury). First-degree type III injury results from even more aggressive nerve manipulation. Segmental demyelination may be present and recovery often takes a few days to weeks. Second-degree injuries involve axonal and myelin interruption but intact endoneurium, epineurium, and perineurium, which allows axonal regeneration. Recovery may be slow and takes up to 1 year for complete return of normal sensation. Intact supporting and connective tissue structures may play an important role. Second-degree injuries are caused by traction or compression of the nerve. Third-degree injuries involve the endoneurium, whereas the epineurium and perineurium remain intact. Cause is similar to first-degree and second-degree injury, and spontaneous recovery is expected. However, complete recovery is not likely. Surgical exploration should be considered. Fourth-degree injuries result from traction, compression, needle injection, or chemical injury and can lead to disruption of the endoneurium and perineurium. The epineurium remains intact. Fourth-degree injuries have a poor potential or spontaneous recovery and a high probability of neuroma formation and intraneural fibrosis. Surgical intervention is required for these injuries. A fifth-degree Sunderland injury corresponds to Seddon’s neurotmesis (nerve transection, avulsion, or laceration) and disruption of all components of the nerve. There is very little chance of spontaneous recovery. Extensive fibrous changes, neuroma formation, and neuropathic changes are seen. Spontaneous recovery is very rare, and microsurgical repair is indicated when able. In the case of witnessed nerve transection, immediate surgical intervention is advocated.
Injury Type | Histologic Findings | Response | Recovery Pattern | Recovery Rate | Treatment |
---|---|---|---|---|---|
First degree (Seddon neuropraxia) | Transient ischemia, anoxia, ± segmental demyelination, intrafascicular edema | Conduction block | Complete | Fast (hours to weeks) | None indicated |
Second degree (Seddon axonotmesis) | Axon and myelin interruption, (intact endoneurium, perineurium, and epineurium) | Wallerian degeneration distal to injury | Complete | Slow (weeks) | None indicated |
Third degree | Injury involves endoneurium (intact perineurium and epineurium) | Wallerian degeneration and some degree of neuron cell death | Variable | Slow (weeks to months) | Nerve exploration may be considered |
Fourth degree | Injury involves endoneurium and perineurium | Wallerian degeneration, neuron cell death, neuroma formation, intrafascicular fibrosis | None | Spontaneous recovery not likely | Microneurosurgery |
Fifth degree (Seddon neurotmesis) | Complete nerve transection, continuity disruption | Wallerian degeneration, cell death, neuroma, fibrosis | None | No spontaneous recovery possible | Microneurosurgery |
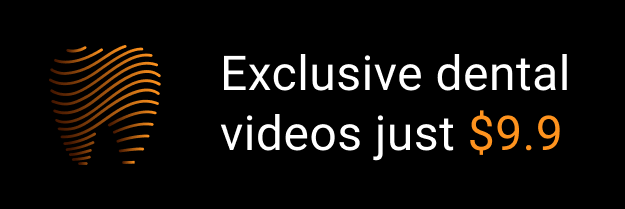