Highlights
- •
A novel hybrid ceramic showed a higher loading capacity than feldspar ceramic cemented on one-piece zirconia implants.
- •
The compressive strength of dental resin composite cements correlates with the fracture load of hybrid and feldspar crowns.
- •
Etching of the intaglio surface of hybrid and feldspar ceramic crowns does not increase the fracture load of the restoration.
Abstract
Objective
Recently a polymer infiltrated hybrid ceramic was developed, which is characterized by a low elastic modulus and therefore may be considered as potential material for implant supported single crowns. The purpose of the study was to evaluate the loading capacity of hybrid ceramic single crowns on one-piece zirconia implants with respect to the cement type.
Methods
Fracture load tests were performed on standardized molar crowns milled from hybrid ceramic or feldspar ceramic, cemented to zirconia implants with either machined or etched intaglio surface using four different resin composite cements. Flexure strength, elastic modulus, indirect tensile strength and compressive strength of the cements were measured. Statistical analysis was performed using two-way ANOVA ( p = 0.05).
Results
The hybrid ceramic exhibited statistically significant higher fracture load values than the feldspar ceramic. Fracture load values and compressive strength values of the respective cements were correlated. Highest fracture load values were achieved with an adhesive cement (1253 ± 148 N). Etching of the intaglio surface did not improve the fracture load.
Significance
Loading capacity of hybrid ceramic single crowns on one-piece zirconia implants is superior to that of feldspar ceramic. To achieve maximal loading capacity for permanent cementation of full-ceramic restorations on zirconia implants, self-adhesive or adhesive cements with a high compressive strength should be used.
1
Introduction
As an alternative to the well-established dental implants made from titanium, zirconium dioxide has been introduced. Zirconia is an inert, non-resorbable and biocompatible metal oxide, which facilitates osseointegration in the form of 3–5 mol% yttria stabilized polycrystalline tetragonal zirconia (Y-TZP) . Esthetic considerations or potential allergies indicate the use of zirconium dioxide instead of titanium, and clinical short-term success rates are promising . However, due to the lack of long-term data the use of zirconia implants in routine clinical practice is not yet recommended .
Implant and suprastructure consist of different materials functioning together as a complex system in withstanding strong intraoral bite forces. Maximum biting forces are reported to be in the range of 286–847 N in the anterior and molar region, respectively . Due to its excellent characteristics such as esthetical properties, chemical stability, biocompatibility, and a coefficient of thermal expansion similar to the natural tooth ceramic might be the material of choice for implant restorations. Unfortunately, under tensile stress ceramic is susceptible to fracture as a result of its brittleness, surface and bulk defects and crack propagation under oral function .
In order to improve the reliability of ceramics a novel polymer infiltrated ceramic was developed . For this material a fracture toughness of 1.21 MPa m 1/2 is reported, which is higher than the one of a typical dental feldspar ceramic (0.92–1.12 MPa m 1/2 ) . In parallel the hybrid ceramic showed a three times lower hardness value (2.92 ± 1.92 GPa) compared to the feldspar ceramic (10.64 ± 0.46 GPa) . In a three point bending test a flexure strength of 144.44 ± 9.61 MPa was measured . Due to its low modulus of elasticity of 31.72 ± 1.43 GPa the hybrid material may work as a buffer area to counterbalance the stiffness of zirconia implants, which is owed to the high elastic modulus of zirconia in the range of 200 GPa and the ankylotic connection to the bone as a result of osseointegration.
When investigating the performance of hybrid ceramic restorations the influence of the cement as an intermediate layer has to be considered. The impact of the cement type on fracture load values of tooth supported restorations has been analyzed in several investigations . The use of a conventional zinc phosphate cement resulted in lower fracture load values for feldspar and resin composite crowns than a cementation with adhesive cement . Glass-infiltrated alumina as well as lithium disilicate and leucite reinforced ceramic crowns luted with a resin composite cement showed higher fracture load values than those luted with a resin modified glass ionomer cement . On a steel analogue of a prepared upper canine, fracture load values of zirconia, lithium disilicate or ceramic fused to metal crowns were not influenced by the type of cement . This was explained by the fact that the intrinsic strength of these materials was so high that cementing with an adhesive cement could not contribute to the fracture strength. In contrast the fracture load of leucite reinforced glass-ceramic crowns significantly increased by the use of adhesive cement when being compared to glass ionomer cement .
The test design has a strong impact on fracture load test results. For instance feldspar ceramic as one of the weaker materials among ceramic systems has been tested with fracture load values of 300–1279 N while using different fracture load test designs . Fracture load values of 833.4 ± 147.5 N and 1272 ± 109 N , respectively were found in two different studies where machined feldspar ceramic crowns were cemented with an adhesive resin composite cement on human teeth or epoxy duplicates of a prepared tooth. These observations indicate that test results cannot easily be matched and a control group is essential in every investigation.
The objective of this study was to compare the fracture load values of a new hybrid ceramic material with a feldspar ceramic on zirconia implants while using different luting cements and to detect any correlation between the fracture load values of the ceramics and mechanical properties of the cements. The hypotheses are that (1) the fracture load values of hybrid ceramic crowns are higher than those of feldspar ceramic crowns and (2) fracture load values of feldspar and hybrid ceramic crowns are influenced by mechanical properties of the cement.
2
Materials and methods
2.1
Implant preparation
Ten one-piece zirconia implants (ceramic implant, VITA Zahnfabrik, Bad Säckingen, Germany) with a diameter of 4.0 mm, a length of 10 mm in the endosseous part and a machined abutment surface ( R a = 0.42 ± 0.06 μm) were used for this study. All implants were embedded according to ISO 14801:2008 in epoxy (RenCast CW 20/Ren HY 49, Huntsman Advanced Materials, Duxford, UK) in order to simulate the elasticity of human bone. The implants were inserted with a 3 mm clearance between implant neck and resin surface as required by the standard.
2.2
Crown preparation and cementation
One implant was scanned with an optical scanner (inEos Blue, Sirona, Bensheim, Germany). A standardized molar crown (46) was designed by CAD-software (inLab SW4.0, Sirona) and milled (inLab MCXL, Sirona) ( Fig. 1 ).

Hundred crowns of a feldspar ceramic (Vitablocs Mark II, VITA) and 100 crowns of a hybrid ceramic (VITA Enamic, VITA) with polished occlusal surfaces were produced following the manufacturer’s recommendations. All crowns were milled with the same design and equipment. Surface polishing was performed manually as it is common practice in a dental laboratory. Trimming and smoothing was done with white polishers, silky luster achieved with pink polishers (porcelain polishers white medium and porcelain polishers pink fine, Hager & Meisinger, Neuss, Germany). All crowns were finally polished with a goat hair buffing wheel and polishing paste (Wetzler Dental, Bielefeld, Deutschland). Prior to cementation crowns and implants were properly cleaned in an ultrasonic bath (TPC-15, Telsonic, Bronschhofen, Switzerland) with 96% ethanol for 5 min. After drying, the crowns were labeled and cemented to the implants with different luting cements under a static load of 20 N for 6 min. Fig. 2 provides the proportions of crown and implant, the cement gap is shown in detail in Fig. 3 . For 50 crowns the intaglio surface was etched for 60 s with hydrofluoric acid prior to cementation (VITA Ceramics Etch, VITA) whereas the other 50 crowns of the respective material were cemented in the machined state. The 200 crowns were cemented to the implants according to the matrix given in Fig. 4 . The materials used are listed in Tables 1 and 2 .
Name | Code | Type | Manufacturers | Lot. no. |
---|---|---|---|---|
VITABLOCS Mark II | VM | Feldspar ceramic | VITA Zahnfabrik, Bad Säckingen, Germany | 27291 |
VITA Enamic | VE | Hybrid ceramic | VITA Zahnfabrik, Bad Säckingen, Germany | 100001 |
Harvard Implant semi-permanent | HIS | Semi-permanent cement, dual curing | Harvard Dental International, Hoppegarten, Germany | 7206489 |
Panavia SA | PSA | Selfadhesive cement, dual curing | Kuraray-Noritake Dental Inc., Kurashiki, Japan | 8V0015 |
Maxcem Elite | MCE | Selfadhesive cement, dual curing | Kerr, Scafaty, Italy | 4870103 |
Multilink Automix | MLA | Adhesive cement, dual curing | Ivoclar Vivadent, Schaan, Liechtenstein | S53599 |
Product name | Composition |
---|---|
Harvard implant semi-permanent | Methacrylates, zinc oxide |
Panavia SA | Bisphenol A diglycidylmethacrylate, sodium fluoride, triethylene glycol dimethacrylate, 10-methacryloyloxydecyl dihydrogen phosphate (MDP), hydrophobic aromatic dimethacrylate, hydrophobic aliphatic dimethacrylate, silanated barium glass filler, silanated colloidal silica di-camphorquinone, initiators, accelerators, catalysts, pigments |
Maxcem Elite | Glyceroldimethacrylate dihydrogen phosphate (GPDM) hydroxyethylmethacrylat (HEMA), 4-methoxyphenol (MEHQ), cumolhydroperoxid (CHPO), methacrylate ester monomers, titanium dioxide, pigments |
Multilink Automix | Bis-GMA, 2-hydroxyethylmethacrylat, 2-dimethylaminoethylmethacrylate, urethandimethacrylate, dibenzoylperoxide, barium glass filler, Ba-Al-fluoro-silicate-glass filler, ytterbiumtrifluoride siliciumdioxidefiller, catalysts, stabilizers, pigments |
The cement excess was eliminated using foam pellets. After the cementation process, the specimens were stored in distilled water for 24 h under permanent temperature control (CTS T-4025, Hechingen, Germany) at 37 °C.
2.3
Fracture load test
All specimens underwent fracture load testing which was performed at a cross-head speed of 1 mm/min. The specimens were placed consecutively in a universal testing machine (Z020, Zwick/Roell, Ulm, Germany) and loaded axially to the implant until fracture ( Fig. 5 ). A steel ball (4.75 mm diameter) was adjusted in the center of the occlusal surface with a 0.2 mm thick tin foil (Dentaurum, Pforzheim, Germany) placed between the ball and the occlusal surface to attain a homogenous stress distribution. The fracture load values were recorded (testXpert V 2.2, Zwick/Roell). After the fracture load test the 10 zirconia implants were carefully cleaned and re-used for the subsequent test series. Remaining cement on the implant abutment was completely removed using a blow-dryer at 200 °C (MZ 6 HL, miolectric, Switzerland). After the removal of the cement the implants were cleaned in an ultrasonic bath (TPC-15, Telsonic) with 96% ethanol for 5 min.
2.4
Fracture analyses
Two specimens of each group were randomly selected for a fracture analysis with a stereomicroscope (Wild M7A, Leica, Heerbrugg, Switzerland) and a scanning electron microscope (Philips XL30 FEG ESEM, Philips Electron Optics, Eindhoven, Netherlands).
2.5
Elastic modulus and flexure strength of the cement materials
For the flexure strength and static elastic modulus measurement, bending bars ( n = 5) with dimensions of 25 mm × 2 mm × 2 mm were prepared in a Teflon mold (Distrelec, Nänikon, CH) according to ISO 4049:2009. The bars were loaded until fracture in a universal testing machine (Z010, Zwick/Roell) in three-point flexure with a crosshead speed of 0.75 mm/min. The fracture stress, σ f , was calculated by the formula (according to ISO 4049:2009):
σ f = 3 F l 2 w h 2
F is the fracture load, l is the roller span (here 20 mm), w is the width and h is the height of the bar.
The static elastic modulus was calculated from the three-point bending results by the formula:
E f = F l 3 4 w h 3 d
d is the deflection corresponding to load F .
2.6
Indirect tensile strength and compressive strength
Indirect tensile strength and compressive strength of the cements were measured with cylindrical test specimens 3 mm in height and diameter. A Teflon mold (Distrelec) was used to produce the test specimens. The cement was filled into the respective cavities of the Teflon mold and kept in place with a plastic foil and a glass plate on each side. After 1 h storage in distilled water at 37 °C the specimens were carefully removed from the mold and stored in water at 37 °C for another 24 h. Specimens with visible bubbles or surface defects were eliminated. Specimens of each cement were produced and loaded until fracture (Z020, Zwick/Roell). Ten specimens were produced to measure the indirect tensile strength and loaded perpendicular whereas another 10 specimens of the respective material were loaded parallel to the cylinder axis to determine compressive strength. Cross-head speed was set to 1 mm/min.
The indirect tensile strength was calculated using the following equation:
σ t = 2 F π d h
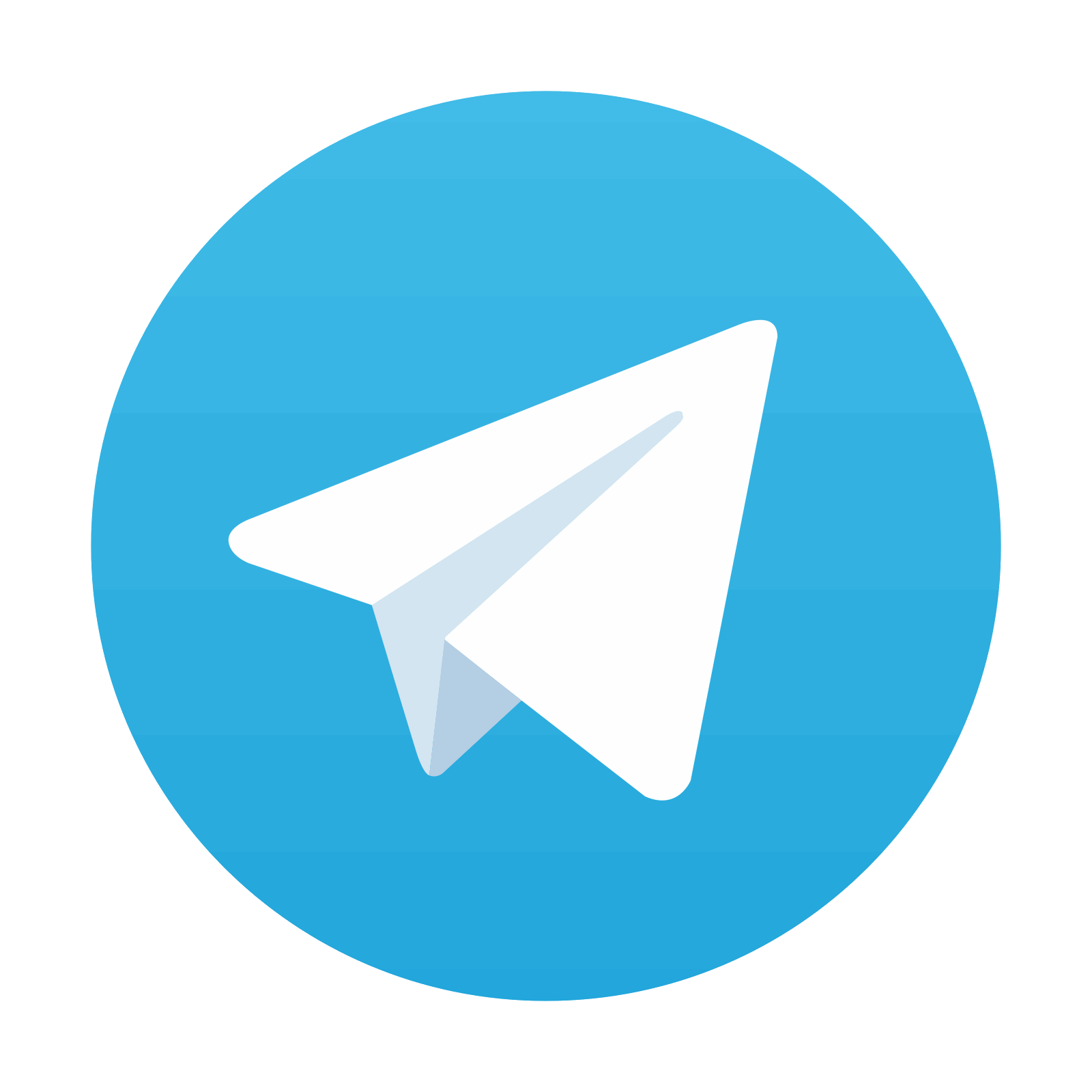
Stay updated, free dental videos. Join our Telegram channel

VIDEdental - Online dental courses
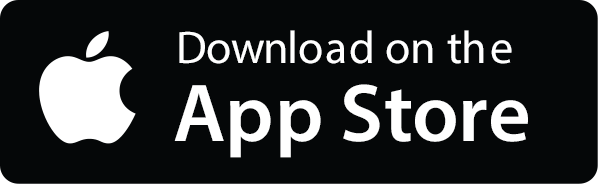
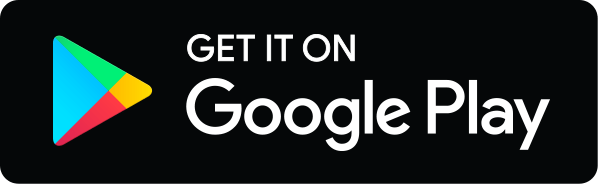