Graphical abstract
Mineral/Matrix ratio (MMR) calculated at the different Raman spectra after principal components analysis. ADH: adhesive; HL: hybrid layer; BHL: bottom hybrid layer; DEN: dentine; PA: phosphoric acid; SB: single-bond; EDTA: ethylenediaminetetraacetic acid; SEB: Clearfil SE bond; ctr: control; CL: cycling.
Abstract
Objectives
The purpose of this study was to evaluate if mechanical loading promotes bioactivity at the resin interface after bonding with three different adhesive approaches.
Methods
Dentin surfaces were subjected to three different treatments: demineralisation by (1) 37% phosphoric acid (PA) followed by application of an etch-and-rinse dentin adhesive Single Bond (SB) (PA + SB), (2) by 0.5 M ethylenediaminetetraacetic acid (EDTA) followed by SB (EDTA + SB), (3) application of a self-etch dentin adhesive: Clearfil SE Bond (SEB). Bonded interfaces were stored in simulated body fluid during 24 h or 3 w. One half of each tooth was submitted to mechanical loading. Remineralisation of the bonded interfaces was assessed by AFM imaging/nano-indentation, Raman spectroscopy/cluster analysis, dye assisted confocal microscopy evaluation (CLSM) and Masson’s trichrome staining.
Results
Loading cycling for 3 w promoted an increase of mechanical properties at the resin–dentin interface. Cluster analysis demonstrated an augmentation of the mineral–matrix ratio in SB-loaded specimens. CLSM showed an absent micropermeability and nanoleakage after loading EDTA + SB and SEB specimens. Trichrome staining reflected a narrow demineralised dentin matrix after loading, almost not observable in EDTA + SB and SEB.
Significance
In vitro mechanical loading promoted mineralization in the resin–dentin interfaces, at 24 h and 3 w of storage.
1
Introduction
Restoring teeth with minimal sacrifice to sound tooth structure following the concept of “minimal invasive dentistry” forms the basis for today’s restorative practice . The major shortcoming of contemporary adhesive restoratives is their limited durability in the mouth , due to the loss of retention and marginal adaptation , specially to dentin.
Two main strategies are used to create dentin bonding: (a) ‘Etch-and-rinse’ adhesives involve a separate etch-and-rinse phase. In their most common configuration, an acid (phosphoric acid/PA or ethylenediaminetetraacetic acid/EDTA) is applied, to demineralize the underlying dentin , and then, rinsed off. This conditioning step is followed by a priming step and application of the adhesive resin; simplified two-step etch-and-rinse adhesives combine the primer and adhesive resin into one application. At dentin, the primary bonding mechanism of etch-and-rinse adhesives is primarily diffusion-based and depends upon the formation of the hybrid layer (HL) or micro-mechanical interlocking of resin within the exposed collagen fibril scaffold . It has been demonstrated a stronger dentin-demineralisation effect of PA compared with EDTA, that will lead, consequently, to a higher volume of demineralised/unprotected collagen, at the bottom of the hybrid layer (BHL). (b) An alternative approach is based on the use of non-rinse acidic monomers that simultaneously condition and prime dentin, the so-called ‘self-etch’ adhesives; the rinsing phase is eliminated, which not only lessens the clinical application time, but also significantly reduces the technique-sensitivity or the risk of making errors during application. Adhesion is consequently obtained micro-mechanically through shallow hybridization and by additional chemical interaction of specific carboxyl/phosphate groups of functional monomers with residual hydroxyapatite . However, a high quality and durable HL can be only achieved if the demineralised dentin collagen matrix is fully resin-infiltrated . Even when the self-etching approach seems to reduce the non-resin covered collagen layer, with both strategies, this unprotected collagen may become the sites for collagen hydrolysis by host-derived matrix metalloproteinase (MMP) enzymes .
Host-derived matrix-metalloproteinases (MMPs 2, 8, 9, 20), a family of structurally related zinc-dependent endopeptidases that are activated during acid-etching procedures, contribute to the degradation of dentin collagen matrix. They also play an important role in dentin matrix modulation during caries progression and in the collagen degradation of the HL, jeopardizing the longevity of bonded restorations . What is needed is a suitable strategy to increase the longevity of adhesive restorations, as can be the inhibition of MMPs activity within the demineralised collagen matrix and to promote additional collagen protection by tissue engineering strategies at the resin–dentin interface. However, achieving remineralisation of dentin remains one of the most difficult tasks in dentistry .
Teeth are continuously subjected to stresses during mastication, swallowing and parafunctional habits . Maximum biting force, recorded on first molars, is approximately 40–90 kgf. Although masticatory loads recorded on a single molar are lower ( ca. 11–27 kgf), they may still represent a challenge to the long-term durability of resin–dentin bonds . Load cycling produced an increase of collagen degradation when PA-demineralised dentin and EDTA-treated dentin were infiltrated with resin . The clinical significance of this outcome has been related with the lowest MMP activity revealed when specimens were loaded after partially demineralised/treated dentin. It might be speculated a relationship between those findings and some process of a partial dentin remineralisation, where mineral crystallites remaining within the collagen after partial demineralisation might act as seed sites for apatite growth . Additionally, it has been demonstrated that in vitro load cycling promoted mineralization at the resin-infiltrated dentin and mineral depleted areas of the resin–dentin interface, at 24 of storage in deionized water.
A main interest has arisen considering whether this hybrid layer protection can be maintained over time or whether it disappears after resin–dentin interfaces storage. The purpose of this study was to evaluate the ability of two dentin adhesives (etch-and-rinse vs. self-etch) to induce remineralisation on the bonded dentin interface created by using different demineralisation procedures of the dentin surface and in vitro mechanical loading application, at three weeks of simulated body fluid (SBF) storage. This study tested the null hypothesis that remineralisation is not produced at the resin–dentin interface after in vitro load cycling, at 21 days of SBF storage.
2
Materials and methods
2.1
Specimen preparation, bonding procedures and mechanical loading
Eighteen non-carious human third molars were obtained with informed consent from donors (20–40 year of age), under a protocol approved by the Institution Review Board. Molars were stored at 4 °C in 0.5% chloramine T for up to 1 month before use. A flat mid-coronal dentin surface was exposed using a hard tissue microtome (Accutom-50; Struers, Copenhagem, Denmark) equipped with a slow-speed, water-cooled diamond wafering saw (330-CA RS-70300, Struers, Copenhagen, Denmark). A 180-grit silicon carbide (SiC) abrasive paper mounted on a water-cooled polishing machine (LaboPol-4, Struers, Copenhagem, Denmark) was used to produce a clinically relevant smear layer . The specimens were divided into three main groups ( n = 6) based on the tested adhesive systems and dentin-etching procedure: (i) two-step etch and rinse adhesive system, Adper™ Single Bond Plus (SB, 3 M ESPE, St. Paul, MN, USA) applied on 37% phosphoric acid (PA), 15 s (PA + SB); (ii) SB applied on EDTA-treated dentin, 0.5 M, 60 s (EDTA + SB); (iii) Clearfil SE Primer and SE Bond (SE, Bond, Kuraray Medical Inc., Tokyo, JAPAN) (SEB). A flowable resin composite (Tetric EvoCeram ® – Ivoclar Vivadent, Schaan, Liechtenstein) was placed incrementally in five 1 mm layers and light-cured with a Translux EC halogen unit (Kulzer GmbH, Bereich Dental, Wehrheim, Germany) for 40 s. The detailed composition and application mode is shown in Table 1 . Half of the teeth were stored for 24 h in simulated body fluid solution (SBF), and the other half during 3 w. Before storing, all teeth were longitudinally cut in two halves. Only one half was submitted to mechanical loading (100,000 cycles, 3 Hz, 49 N) (S-MMT-250NB; Shimadzu, Tokyo, Japan). Specimens stored for 3 w were loaded 25,000 cycles every 5 d. To proceed with the mechanical loading, specimens were mounted in plastic rings using a dental stone. The cycling compressive load was applied to the flat resin composite build-ups using a 5-mm diameter spherical stainless steel plunger, while immersed in deionised water . All specimens were further longitudinally sectioned to obtain a 1.5 mm slab from the central part of the specimen. It was polished through SiC abrasive papers from 800 up to 4000-grit with a final polishing procedure performed with diamond pastes (Buehler-MetaDi, Buheler Ltd. Lake Bluff, IL, USA) through 1 μm down to 0.25 μm. The specimens were treated in ultrasonic bath (Model QS3, Ultrawave Ltd., Cardiff, UK) containing deionised water [pH 7.4] for 5 min at each polishing step.
Etch-and-rinse [Single Bond (SB) (3 M ESPE, USA)] |
Composition | |
HEMA | Dentin conditioning | |
Bis-GMA | 37% H 3 PO 4 (15 s) | |
Ethanol | 0.5 M EDTA (60 s) | |
PAM | Adhesive application | |
UDMA | Rinse with water | |
CQ | Air-dry (5 s) | |
ODMAB: | Adhesive application (30 s) | |
Tripheylantimonydicaroxylates | Light activation (15 s) | |
Phosphine | ||
Water | ||
Quartz | ||
Self-etching [Clearfil SE primer and bond (SEB) (Kuraray, Japan)] |
Composition Primer | |
MDP | ||
HEMA | ||
CQ | Adhesive application | |
N,N-diethanol p-toluidine | Rinse with water | |
Water | Air-dry (5 s) | |
Primer application (20 s) | ||
Air-dry (3 s) | ||
Composition Bond | Adhesive application (10 s) | |
Bis–GMA | Light activation (15 s) | |
MDP | ||
HEMA | ||
CQ | ||
N,N-diethanol p-toluidine | ||
Silanated colloidal silica. |
2.2
AFM imaging and nano-indentation
An atomic force microscope (AFM – Nanoscope V, Digital Instruments, Veeco Metrology group, Santa Barbara, CA, USA) equipped with a Triboscope indentor system (Hysitron Inc., Minneapolis, MN, USA) and a Berkovich indenter (tip radius ∼20 nm) was employed for the imaging and indentation processes in a fully hydrated status . For each subgroup, three slabs were tested. On each slab, five indentation lines were executed in five different mesio-distal positions along the interface in a straight line starting from the adhesive layer down to the intertubular dentin. Indentations were performed with a load of 4000 nN and a time function of 10 s. The distance between each indentation was kept constant by adjusting the distance intervals in 5 (±1) μm steps and the load function . Hardness (Hi) and modulus of elasticity (Ei) data were registered. Mean nanohardness and Young modulus values were obtained in GPa. Kolmogorov–Smirnov test was conducted to assess normal data distribution ( P > 0.05) and data were analyzed by ANOVA including analysis of interactions ( P < 0.05). Adhesive procedure, storage time and load cycling were considered as independent variables. Student t test ( P < 0.001) and Student–Newman–Keuls ( P < 0.05) were used for post hoc comparisons.
2.3
Raman spectroscopy and cluster analysis
A dispersive Raman spectrometer/microscope (Horiba Scientific Xplora, Villeneuve d́Ascq, France) was also used to analyze bonded interfaces. A 785-nm diode laser (100 mW sample power) through a X100/0.90 NA air objective was employed. Raman signal was acquired using a 600-lines/mm grating centered between 900 and 1800 cm −1 . Chemical mapping of the interfaces were performed and submitted to K -means cluster analysis using the multivariate analysis tool (ISys® Horiba), which includes statistical pattern to derive the independent clusters. The K -means clustering (KMC) is a method of cluster analysis based on a centroid model which aims to partition n observations into k clusters in which each observation belongs to the cluster with the nearest mean . The natural groups of components (or data) based on some similarity and the centroids of a group of data sets were found by the clustering algorithm once calculated by the software. To determine cluster membership, this algorithm evaluated the distance between a point and the cluster centroids. The output from a clustering algorithm was basically a statistical description of the cluster centroids with the number of components in each cluster. The biochemical content of each cluster was analyzed using the average cluster spectra . Four to five clusters were identified and values for each cluster such as hybrid layer, dentin, and adhesive within the interface were independently obtained. Principal component analysis (PCA) decomposed data set into a bilinear model of linear independent variables, the so-called principal components (PC S ). At this point, the mineral matrix ratio (MMR) (the ratio of integrated areas of the phosphate v 1-{961 cm −1 }, P–O symmetric stretch, contour to the collagen at 1465 cm −1 , CH 2 deformation) was measured for the same cluster in each different group, in order to quantitatively calculate the extent of mineralization along the interface subsequent the mechanical loading.
2.4
Dye assisted confocal microscopy evaluation (CLSM)
Twenty-four further dentin-bonded specimens were prepared as previously described for each group with the primer/bond resins doped with 0.05 wt% Rhodamine-B (Rh-B: Sigma–Aldrich Chemie Gmbh, Riedstr, Germany). Half of the specimens were submitted to mechanical loading (as described in previous section); afterwards, the pulpal chamber was filled with 1 wt% aqueous/ethanol fluorescein (Sigma–Aldrich Chemie Gmbh, Riedstr, Germany) for 3 h . Specimens were copiously rinsed with water and treated in an ultrasonic water bath for 2 min. Then, they were cut in resin–dentin slabs and polished using ascending grit SiC abrasive papers (#1200–#4000) on a water-cooled polishing device (Buehler-MetaDi, Buehler Ltd. Lake Bluff, IL, USA). A final ultrasonic treatment (5 min) concluded the specimen preparation. Analysis of bonded interfaces was performed using a confocal laser scanning microscope (SP5 Leica, Heidelberg, Germany) equipped with a 20×, 40× and 60× oil immersion lenses. The fluorescein was excited at 488-nm, while the ultramorphology evaluation (resin-diffusion) was executed using a 568-nm krypton (rhodamine excitation) laser. CLSM images were obtained with a 1 μm z -step to optically section the specimens to a depth up to 12–10 μm below the surface. The z -axis scans of the interface surface were arbitrarily pseudo-colored by the same operator for better exposure and compiled into single projections using the Leica image-processing software SP2 (Leica, Heidelberg, Germany). Five optical images were randomly captured from each resin–dentin interface. Micrographs representing the most common features of nanoleakage observed along the bonded interfaces were captured .
2.5
Light microscopy–Masson’s trichrome staining
The resin–dentin bonded slices of each group (PA + SB, EDTA + SB, SEB, PA + SB cycling, EDTA + SB cycling and SEB cycling) after 24 h and 3 w of storage were used for the histomorphological evaluations. The medial aspects of each resin–dentin bonded slice was fixed in a glass holder with a photo curing adhesive (Technovit 7210 VLC, Heraeus Kulzer GmbH Co.,Werheim, Germany) and ground with SiC papers of increasing fine grits (800, 1000, 1200 and 4000) in a polisher (Exakt, Apparatebau D-2000, Norderstedt, Germany) until its thickness was approximately 10 mm. Slices were stained with Masson’s trichrome for differentiation of resin and non-resin encapsulation of the exposed collagen. This dye has a high affinity for cationic elements of normally mineralized type I collagen, resulting in staining collagen green, and when demineralised, resulting in different coloration, generally red; collagen coated with adhesive stains orange and pure adhesive appears beige. Slides with adherent stained sections were dehydrated through ascending ethanol and xylene. The sections were cover slipped and examined by light microscopy (BH-2, Olympus, Tokyo, Japan) at 100× magnifications. Three slices were prepared from each specimen, and images were digitalized in a scanner (Agfa Twin 1200, Agfa-Gevaert NV Mortsel, Belgium). In each specimen, the presence or absence of a red band (that would correspond to demineralised dentin) was observed. A qualitative assessment of the collagen encapsulation was completed by observing color differences within the interfacial zones of resin–dentin interfaces .
2
Materials and methods
2.1
Specimen preparation, bonding procedures and mechanical loading
Eighteen non-carious human third molars were obtained with informed consent from donors (20–40 year of age), under a protocol approved by the Institution Review Board. Molars were stored at 4 °C in 0.5% chloramine T for up to 1 month before use. A flat mid-coronal dentin surface was exposed using a hard tissue microtome (Accutom-50; Struers, Copenhagem, Denmark) equipped with a slow-speed, water-cooled diamond wafering saw (330-CA RS-70300, Struers, Copenhagen, Denmark). A 180-grit silicon carbide (SiC) abrasive paper mounted on a water-cooled polishing machine (LaboPol-4, Struers, Copenhagem, Denmark) was used to produce a clinically relevant smear layer . The specimens were divided into three main groups ( n = 6) based on the tested adhesive systems and dentin-etching procedure: (i) two-step etch and rinse adhesive system, Adper™ Single Bond Plus (SB, 3 M ESPE, St. Paul, MN, USA) applied on 37% phosphoric acid (PA), 15 s (PA + SB); (ii) SB applied on EDTA-treated dentin, 0.5 M, 60 s (EDTA + SB); (iii) Clearfil SE Primer and SE Bond (SE, Bond, Kuraray Medical Inc., Tokyo, JAPAN) (SEB). A flowable resin composite (Tetric EvoCeram ® – Ivoclar Vivadent, Schaan, Liechtenstein) was placed incrementally in five 1 mm layers and light-cured with a Translux EC halogen unit (Kulzer GmbH, Bereich Dental, Wehrheim, Germany) for 40 s. The detailed composition and application mode is shown in Table 1 . Half of the teeth were stored for 24 h in simulated body fluid solution (SBF), and the other half during 3 w. Before storing, all teeth were longitudinally cut in two halves. Only one half was submitted to mechanical loading (100,000 cycles, 3 Hz, 49 N) (S-MMT-250NB; Shimadzu, Tokyo, Japan). Specimens stored for 3 w were loaded 25,000 cycles every 5 d. To proceed with the mechanical loading, specimens were mounted in plastic rings using a dental stone. The cycling compressive load was applied to the flat resin composite build-ups using a 5-mm diameter spherical stainless steel plunger, while immersed in deionised water . All specimens were further longitudinally sectioned to obtain a 1.5 mm slab from the central part of the specimen. It was polished through SiC abrasive papers from 800 up to 4000-grit with a final polishing procedure performed with diamond pastes (Buehler-MetaDi, Buheler Ltd. Lake Bluff, IL, USA) through 1 μm down to 0.25 μm. The specimens were treated in ultrasonic bath (Model QS3, Ultrawave Ltd., Cardiff, UK) containing deionised water [pH 7.4] for 5 min at each polishing step.
Etch-and-rinse [Single Bond (SB) (3 M ESPE, USA)] |
Composition | |
HEMA | Dentin conditioning | |
Bis-GMA | 37% H 3 PO 4 (15 s) | |
Ethanol | 0.5 M EDTA (60 s) | |
PAM | Adhesive application | |
UDMA | Rinse with water | |
CQ | Air-dry (5 s) | |
ODMAB: | Adhesive application (30 s) | |
Tripheylantimonydicaroxylates | Light activation (15 s) | |
Phosphine | ||
Water | ||
Quartz | ||
Self-etching [Clearfil SE primer and bond (SEB) (Kuraray, Japan)] |
Composition Primer | |
MDP | ||
HEMA | ||
CQ | Adhesive application | |
N,N-diethanol p-toluidine | Rinse with water | |
Water | Air-dry (5 s) | |
Primer application (20 s) | ||
Air-dry (3 s) | ||
Composition Bond | Adhesive application (10 s) | |
Bis–GMA | Light activation (15 s) | |
MDP | ||
HEMA | ||
CQ | ||
N,N-diethanol p-toluidine | ||
Silanated colloidal silica. |
2.2
AFM imaging and nano-indentation
An atomic force microscope (AFM – Nanoscope V, Digital Instruments, Veeco Metrology group, Santa Barbara, CA, USA) equipped with a Triboscope indentor system (Hysitron Inc., Minneapolis, MN, USA) and a Berkovich indenter (tip radius ∼20 nm) was employed for the imaging and indentation processes in a fully hydrated status . For each subgroup, three slabs were tested. On each slab, five indentation lines were executed in five different mesio-distal positions along the interface in a straight line starting from the adhesive layer down to the intertubular dentin. Indentations were performed with a load of 4000 nN and a time function of 10 s. The distance between each indentation was kept constant by adjusting the distance intervals in 5 (±1) μm steps and the load function . Hardness (Hi) and modulus of elasticity (Ei) data were registered. Mean nanohardness and Young modulus values were obtained in GPa. Kolmogorov–Smirnov test was conducted to assess normal data distribution ( P > 0.05) and data were analyzed by ANOVA including analysis of interactions ( P < 0.05). Adhesive procedure, storage time and load cycling were considered as independent variables. Student t test ( P < 0.001) and Student–Newman–Keuls ( P < 0.05) were used for post hoc comparisons.
2.3
Raman spectroscopy and cluster analysis
A dispersive Raman spectrometer/microscope (Horiba Scientific Xplora, Villeneuve d́Ascq, France) was also used to analyze bonded interfaces. A 785-nm diode laser (100 mW sample power) through a X100/0.90 NA air objective was employed. Raman signal was acquired using a 600-lines/mm grating centered between 900 and 1800 cm −1 . Chemical mapping of the interfaces were performed and submitted to K -means cluster analysis using the multivariate analysis tool (ISys® Horiba), which includes statistical pattern to derive the independent clusters. The K -means clustering (KMC) is a method of cluster analysis based on a centroid model which aims to partition n observations into k clusters in which each observation belongs to the cluster with the nearest mean . The natural groups of components (or data) based on some similarity and the centroids of a group of data sets were found by the clustering algorithm once calculated by the software. To determine cluster membership, this algorithm evaluated the distance between a point and the cluster centroids. The output from a clustering algorithm was basically a statistical description of the cluster centroids with the number of components in each cluster. The biochemical content of each cluster was analyzed using the average cluster spectra . Four to five clusters were identified and values for each cluster such as hybrid layer, dentin, and adhesive within the interface were independently obtained. Principal component analysis (PCA) decomposed data set into a bilinear model of linear independent variables, the so-called principal components (PC S ). At this point, the mineral matrix ratio (MMR) (the ratio of integrated areas of the phosphate v 1-{961 cm −1 }, P–O symmetric stretch, contour to the collagen at 1465 cm −1 , CH 2 deformation) was measured for the same cluster in each different group, in order to quantitatively calculate the extent of mineralization along the interface subsequent the mechanical loading.
2.4
Dye assisted confocal microscopy evaluation (CLSM)
Twenty-four further dentin-bonded specimens were prepared as previously described for each group with the primer/bond resins doped with 0.05 wt% Rhodamine-B (Rh-B: Sigma–Aldrich Chemie Gmbh, Riedstr, Germany). Half of the specimens were submitted to mechanical loading (as described in previous section); afterwards, the pulpal chamber was filled with 1 wt% aqueous/ethanol fluorescein (Sigma–Aldrich Chemie Gmbh, Riedstr, Germany) for 3 h . Specimens were copiously rinsed with water and treated in an ultrasonic water bath for 2 min. Then, they were cut in resin–dentin slabs and polished using ascending grit SiC abrasive papers (#1200–#4000) on a water-cooled polishing device (Buehler-MetaDi, Buehler Ltd. Lake Bluff, IL, USA). A final ultrasonic treatment (5 min) concluded the specimen preparation. Analysis of bonded interfaces was performed using a confocal laser scanning microscope (SP5 Leica, Heidelberg, Germany) equipped with a 20×, 40× and 60× oil immersion lenses. The fluorescein was excited at 488-nm, while the ultramorphology evaluation (resin-diffusion) was executed using a 568-nm krypton (rhodamine excitation) laser. CLSM images were obtained with a 1 μm z -step to optically section the specimens to a depth up to 12–10 μm below the surface. The z -axis scans of the interface surface were arbitrarily pseudo-colored by the same operator for better exposure and compiled into single projections using the Leica image-processing software SP2 (Leica, Heidelberg, Germany). Five optical images were randomly captured from each resin–dentin interface. Micrographs representing the most common features of nanoleakage observed along the bonded interfaces were captured .
2.5
Light microscopy–Masson’s trichrome staining
The resin–dentin bonded slices of each group (PA + SB, EDTA + SB, SEB, PA + SB cycling, EDTA + SB cycling and SEB cycling) after 24 h and 3 w of storage were used for the histomorphological evaluations. The medial aspects of each resin–dentin bonded slice was fixed in a glass holder with a photo curing adhesive (Technovit 7210 VLC, Heraeus Kulzer GmbH Co.,Werheim, Germany) and ground with SiC papers of increasing fine grits (800, 1000, 1200 and 4000) in a polisher (Exakt, Apparatebau D-2000, Norderstedt, Germany) until its thickness was approximately 10 mm. Slices were stained with Masson’s trichrome for differentiation of resin and non-resin encapsulation of the exposed collagen. This dye has a high affinity for cationic elements of normally mineralized type I collagen, resulting in staining collagen green, and when demineralised, resulting in different coloration, generally red; collagen coated with adhesive stains orange and pure adhesive appears beige. Slides with adherent stained sections were dehydrated through ascending ethanol and xylene. The sections were cover slipped and examined by light microscopy (BH-2, Olympus, Tokyo, Japan) at 100× magnifications. Three slices were prepared from each specimen, and images were digitalized in a scanner (Agfa Twin 1200, Agfa-Gevaert NV Mortsel, Belgium). In each specimen, the presence or absence of a red band (that would correspond to demineralised dentin) was observed. A qualitative assessment of the collagen encapsulation was completed by observing color differences within the interfacial zones of resin–dentin interfaces .
3
Results
3.1
AFM imaging and nano-indentation
The nanomechanical properties (Hi-nanohardness and Ei-Young modulus) of the resin bonded interfaces at the second (HL) and the third (BHL) indentations were influenced by the main effects ( P < 0.001), concretely: adhesive procedure ( P < 0.001), load cycling ( P < 0.001 in nanohardness-HL, nanohardness-BHL, Young modulus-BHL; P = 0.014 in nanohardness-HL) and time of storage ( P = 0.009 in nanohardness-HL, P < 0.001 in nanohardness-BHL, P = 0.007 in Young modulus-HL and P = 0.013 in Young modulus-BHL ( Table 2 ). Interactions between factors were also significant ( P < 0.001), except in Ei at HL ( P = 0.10) ( Table 2 ). At 24 h storage, both Hi and Ei, increased in SEB, at the second indentation (HL); groups obtained with SB (PA or EDTA) performed similar. Nanohardness-BHL was lower in PA + SB than in EDTA + SB and SEB, which performed similar. Young modulus-BHL in PA + SB and SEB were lower than in EDTA + SB. At 3 w of storage, Hi attained the following trend at HL: EDTA + SB > SEB > PA + SB; at BHL, PA + SB showed the lowest values and EDTA + SB and SEB performed similar. Ei at both HL and BHL was lower in PA + SB than in EDTA + SB and SEB, which performed similar. When specimens were load cycled, both EDTA + SB and SEB performed similar and attained higher Hi than PA + SB in both HL and BHL, at 24 h storage. On the contrary, Ei followed similar trend, except in SEB at BHL, which was superior to the rest of groups, which performed similar. At 3 w storage, in Hi, groups reflected the following trend at both HL and BHL: SEB > EDTA + SB = PA + SB; but in Young modulus-HL, PA + SB attained the lowest values, and EDTA + SB the highest at BHL, after 3 w storage. At 24 h storage, within the adhesive procedure, load cycling increased Hi in all groups at HL and BHL, except at HL in PA + SB. On the contrary, load cycling did not affect Ei at HL in PA + SB and at BHL in EDTA + SB. At 3 w storage, cycling increased Hi and Hi in all groups at both HL and BHL, except in EDTA + SB at HL. At the resin–dentin interface, Hi increased after 3 w storage at HL in EDTA + SB unloaded, and in SEB at both HL and BHL when loading. Three weeks storage incremented Ei in all groups, except in EDTA + SB and SEB at HL.
Indentation zone | Anova results for nanohardness | Anova results for young modulus |
---|---|---|
1st Indentation Adhesive layer |
Main effects F = 12.38 P < 0.001 | Main effects F = 18.26 P < 0.001 |
Adhesive F = 7.63 P = 0.001 | Adhesive F = 29.59 P < 0.001 | |
Load F = 3.86 P = 0.04 | Load F = 6.00 P = 0.018 | |
Time F = 31.24 P < 0.001 | Time F = 8.61 P = 0.005 | |
Interactions F = 3.25 P = 0.013 | Interactions F = 10.99 P < 0.001 | |
Time-adhesive F = 4.46 P = 0.017 | Time-adhesive F = 9.71 P < 0.001 | |
Time-load F = 1.07 P = 0.30 | Time-load F = 2.827 P = 0.1 | |
Adhesive-load F = 2.69 P = 0.04 | Adhesive-load F = 15.77 P < 0.001 | |
R = 0.66 | R = 0.65 | |
2nd Indentation Hybrid layer |
Main effects F = 25.52 P < 0.001 | Main effects F = 18.78 P < 0.001 |
Adhesive F = 27.57 P < 0.001 | Adhesive F = 24.72 P < 0.001 | |
Load cycling F = 25.57 P < 0.001 | Load cycling F = 6.50 P = 0.014 | |
Time F = 7.59 P = 0.009 | Time F = 8.07 P = 0.007 | |
Interactions F = 8.38 P < 0.001 | Interactions F = 2.09 P = 0.10 | |
Time-adhesive F = 6.60 P = 0.003 | Time-adhesive F = 3.20 P = 0.08 | |
Time-load F = 5.42 P = 0.025 | Time-load F = 3.61 P = 0.08 | |
Adhesive-load F = 9.30 P < 0.001 | Adhesive-load F = 0.19 P = 0.82 | |
R = 0.74 | R = 0.78 | |
3rd Indentation Bottom hybrid layer |
Main effects F = 46.47 P < 0.001 | Main effects F = 55.59 P < 0.001 |
Adhesive F = 28.86 P < 0.001 | Adhesive F = 30.34 P < 0.001 | |
Load cycling F = 106.67 P < 0.001 | Load cycling F = 161.02 P < 0.001 | |
Time F = 17.99 P < 0.001 | Time F = 6.62 P = 0.013 | |
Interactions F = 7.63 P < 0.001 | Interactions F = 5.94 P < 0.001 | |
Time-adhesive F = 1.38 P = 0.26 | Time-adhesive F = 9.48 P < 0.001 | |
Time-load F = 10.55 P = 0.002 | Time-load F = 0.05 P = 0.81 | |
Adhesive-load F = 12.15 P < 0.001 | Adhesive-load F = 5.05 P = 0.01 | |
R = 0.83 | R = 0.86 | |
4th Indentation Underlying dentin |
Main effects F = 2.01 P = 0.11 | Main effects F = 19.84 P < 0.001 |
Adhesive F = 3.48 P = 0.04 | Adhesive F = 20.78 P < 0.001 | |
Load cycling F = .59 P = 0.44 | Load cycling F = 7.61 P = 0.009 | |
Time F = .74 P = 0.39 | Time F = 24.19 P < 0.001 | |
Interactions F = 4.92 P = 0.001 | Interactions F = 3.306 P = 0.013 | |
Time-Adhesive F = 1.93 P = 0.16 | Time-Adhesive F = 3.49 P = 0.04 | |
Time-load F = 4.17 P = 0.04 | Time-load F = 8.63 P = 0.005 | |
Adhesive-load F = 8.63 P = 0.001 | Adhesive-load F = 0.59 P = 0.55 | |
R = 0.33 | R = 0.76 | |
5th Indentation Underlying dentin |
Main effects F = 14.044 P < 0.001 | Main effects F = 8.76 P < 0.001 |
Adhesive F = 3.36 P = 0.04 | Adhesive F = 13.96 P < 0.001 | |
Load cycling F = 1.07 P = 0.30 | Load cycling F = 5.65 P = 0.02 | |
Time F = 49.34 P < 0.001 | Time F = 1.17 P = .286 | |
Interactions F = 2.62 P = 0.04 | Interactions F = 8.49 P < 0.001 | |
Time-adhesive F = 4.38 P = 0 .02 | Time-adhesive F = 18.31 P < 0.001 | |
Time-load F = 2.56 P = 0.12 | Time-load F = 1.78 P = 0.19 | |
Adhesive-load F = 0.85 P = 0.43 | Adhesive-load F = 2.11 P = 0.13 | |
R = 0.53 | R = 0.55 |
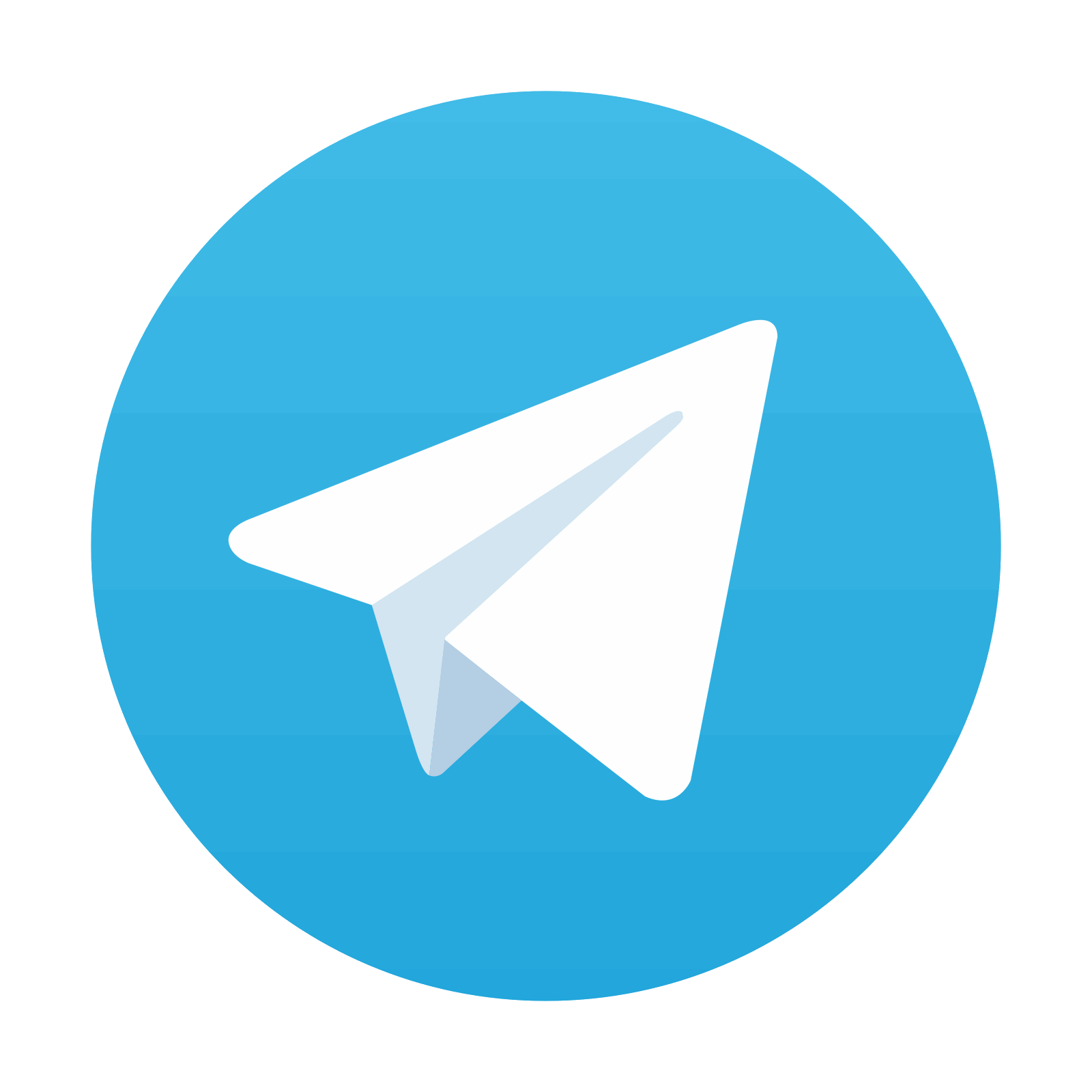
Stay updated, free dental videos. Join our Telegram channel

VIDEdental - Online dental courses
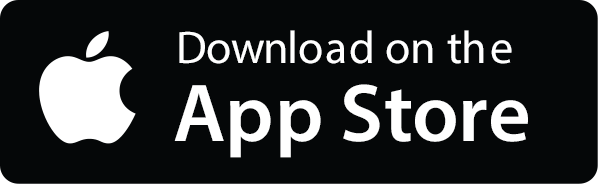
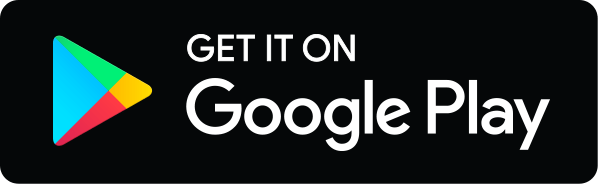
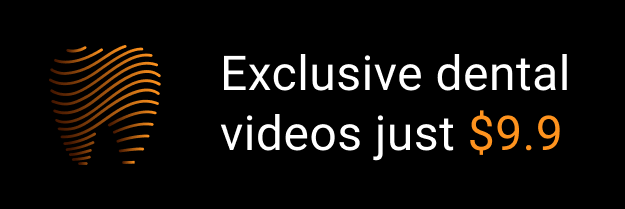