In this review, the authors discuss the use of laser photothermolysis and laser resurfacing in the management of hypertrophic burn scars. They provide details regarding preoperative selection, intraoperative decision making, and postoperative care.
Key points
- •
Lasers can be used safely to treat hypertrophic burn scars.
- •
Pulsed-dye lasers improve the vascularity of hypertrophic burn scars and reduce pruritis, redness, and sometimes neuropathic pain.
- •
Fractional CO 2 laser resurfacing decreases the thickness and improves the pliability of hypertrophic burn scars, allowing for improved range of motion across joints.
Lasers in medicine
Records for the use of light for medical purposes can be found dating back to 4000 bc . In 1917, Albert Einstein described the essential formulas and theoretic concepts of laser light; however, it was not until 1960 that the first laser was successfully operated. The word LASER is an acronym for L ight A mplification by S timulated E mission of R adiation. Laser light has three unique properties which are different from conventional light: (1) it is monochromatic because the wavelength of laser light approaches unity, (2) it is coherent because the light waves are spatially and temporally in phase, and (3) it is collimated because the light waves are parallel and not divergent.
To achieve a precise biologic effect and minimize collateral damage, laser light must be absorbed by a specific component of skin, which is usually a function of the wavelength and pulse duration. The 3 main chromophores of skin-absorbing laser light are melanin, hemoglobin, and intracellular or extracellular water, which are specifically targeted by different laser devices. Fig. 1 illustrates how different wavelengths pass different depths and affect different structures in the body. The depth of penetration for any laser can usually be altered by increasing the power output of the laser.
Since Castro and colleagues in 1983 described for the first time that laser treatment may be a useful treatment modality for hypertrophic and keloid scars, techniques have evolved dramatically. Demonstrated improvement in erythematous and hypertrophic scars was reported in 1994 using 585-nm flashlamp-pumped pulsed dye laser. However, with the introduction of fractional photothermolysis in 2004, there has been a significant breakthrough in clinical laser science. Fractional lasers use technology that splits the laser beam into a pixelated pattern of microbeams, creating hundreds of small thermal injuries to the skin, and leaving the surrounding areas uninjured. These tiny thermal microscopic wounds that reach deep dermal depth allow a rapid healing response, and the islands of untreated skin serve as a reservoir of viable tissue to promote neocollagenesis and tissue remodeling.
This method of fractional skin resurfacing has led to clinical efficacy in aesthetic and scar treatments with high physician and patient approval. Fractional lasers have a superior safety profile when compared with traditional resurfacing techniques.
Fractional laser devices may be either nonablative or ablative. Nonablative fractional photothermolysis heats up the dermis from 50°C to 70°C, which induces collagen to undergo irreversible coagulation of proteins. Ablative fractional photothermolysis heats up the dermis to greater than 100°C, causing vaporization of tissue with the immediate area surrounded by a thermal coagulation zone ( Fig. 2 ).
Nonablative fractional resurfacing (NAFR) devices were introduced in 2004 with increased safety profile but decreased efficacy versus traditional resurfacing devices. NAFR has become a popular worldwide procedure because of its effective epidermal and dermal rejuvenation, increased safety profile, decreased postoperative downtime, use on all anatomic areas, and wide spectrum of medical and aesthetic indications. NAFR can be used for mild to moderate rhytides, leukoderma, atrophic and hypertrophic scars, and other indications such as melasma and striae. Despite the success of nonablative fractional lasers, the need for more aggressive tissue ablation for the purposes of rejuvenation of severely photodamaged skin, deeper rhytides, and severe scars remained.
Ablative fractional resurfacing (AFR) devices entered the market in 2007 with improved efficacy for rhytides, photodamage, and scars. AFR have increased efficacy over NAFR and provide a better safety profile than traditional resurfacing, because a more vigorous remodeling response is triggered, thereby being highly effective for the treatment of hypertrophic scars. There are many fractional devices presently on the market. Many of these devices have distinct technological characteristics that maximize safety and efficacy. This article reviews and discusses fractional devices and fractional treatment pearls in particular.
Lasers in medicine
Records for the use of light for medical purposes can be found dating back to 4000 bc . In 1917, Albert Einstein described the essential formulas and theoretic concepts of laser light; however, it was not until 1960 that the first laser was successfully operated. The word LASER is an acronym for L ight A mplification by S timulated E mission of R adiation. Laser light has three unique properties which are different from conventional light: (1) it is monochromatic because the wavelength of laser light approaches unity, (2) it is coherent because the light waves are spatially and temporally in phase, and (3) it is collimated because the light waves are parallel and not divergent.
To achieve a precise biologic effect and minimize collateral damage, laser light must be absorbed by a specific component of skin, which is usually a function of the wavelength and pulse duration. The 3 main chromophores of skin-absorbing laser light are melanin, hemoglobin, and intracellular or extracellular water, which are specifically targeted by different laser devices. Fig. 1 illustrates how different wavelengths pass different depths and affect different structures in the body. The depth of penetration for any laser can usually be altered by increasing the power output of the laser.
Since Castro and colleagues in 1983 described for the first time that laser treatment may be a useful treatment modality for hypertrophic and keloid scars, techniques have evolved dramatically. Demonstrated improvement in erythematous and hypertrophic scars was reported in 1994 using 585-nm flashlamp-pumped pulsed dye laser. However, with the introduction of fractional photothermolysis in 2004, there has been a significant breakthrough in clinical laser science. Fractional lasers use technology that splits the laser beam into a pixelated pattern of microbeams, creating hundreds of small thermal injuries to the skin, and leaving the surrounding areas uninjured. These tiny thermal microscopic wounds that reach deep dermal depth allow a rapid healing response, and the islands of untreated skin serve as a reservoir of viable tissue to promote neocollagenesis and tissue remodeling.
This method of fractional skin resurfacing has led to clinical efficacy in aesthetic and scar treatments with high physician and patient approval. Fractional lasers have a superior safety profile when compared with traditional resurfacing techniques.
Fractional laser devices may be either nonablative or ablative. Nonablative fractional photothermolysis heats up the dermis from 50°C to 70°C, which induces collagen to undergo irreversible coagulation of proteins. Ablative fractional photothermolysis heats up the dermis to greater than 100°C, causing vaporization of tissue with the immediate area surrounded by a thermal coagulation zone ( Fig. 2 ).
Nonablative fractional resurfacing (NAFR) devices were introduced in 2004 with increased safety profile but decreased efficacy versus traditional resurfacing devices. NAFR has become a popular worldwide procedure because of its effective epidermal and dermal rejuvenation, increased safety profile, decreased postoperative downtime, use on all anatomic areas, and wide spectrum of medical and aesthetic indications. NAFR can be used for mild to moderate rhytides, leukoderma, atrophic and hypertrophic scars, and other indications such as melasma and striae. Despite the success of nonablative fractional lasers, the need for more aggressive tissue ablation for the purposes of rejuvenation of severely photodamaged skin, deeper rhytides, and severe scars remained.
Ablative fractional resurfacing (AFR) devices entered the market in 2007 with improved efficacy for rhytides, photodamage, and scars. AFR have increased efficacy over NAFR and provide a better safety profile than traditional resurfacing, because a more vigorous remodeling response is triggered, thereby being highly effective for the treatment of hypertrophic scars. There are many fractional devices presently on the market. Many of these devices have distinct technological characteristics that maximize safety and efficacy. This article reviews and discusses fractional devices and fractional treatment pearls in particular.
Principles of laser application in burn scar management
When approaching burn patients, it is important to recall what they are confronted with, once their wounds are closed and what then dictates their quality of life. Burn scars can create significant morbidity and remain as a permanent reminder of the damage that has occurred. Diligent, professional wound care in the acute setting followed by time and assiduous scar management with conventional modalities such as physical therapy, intralesional steroids, and surgical revision can mitigate some of the effects of these scars. However, despite skilled care and aside from the obvious stigma, burn patients often suffer from severe pruritus, neuropathic pain, and contractures limiting range of motion. These factors may all have detrimental physical, aesthetic, and social consequences and are associated with substantial costs for modern health care systems. Advances in medical care have led to unprecedented survival rates after extensive injuries, such as from burns and battlefield injuries, further widening the gap in treatment of severely scarred patients.
Burn Scars Are a Complex Tissue Requiring a Holistic Approach
Burn scars have multiple characteristics, such as altered vascularization, dyschromia, structural changes, tension, and contour abnormalities. To achieve an optimal outcome, all aspects of a burn scar should be addressed by a holistic treatment approach.
Abnormal scarring occurs by a loss of control mechanisms that regulate the balance of tissue repair and regeneration. Burn wounds in particular are prone to hypertrophic scarring because of the inflammatory nature of the wound and the prolonged course of wound healing. An imbalance in biosynthesis and tissue degradation during the wound-healing process initiates an excess of collagen production and fibroblast proliferation in hypertrophic and keloid scars, so that they become firm and raised with an irregular surface and tension. Fibroblasts are a highly mechanoresponsive cell type known to play a key role in contractures and hypertrophic scarring. In vitro studies suggest that mechanical strain upregulates matrix remodeling genes and downregulates normal cellular apoptosis, resulting in more cells, each of which produces more matrix. This “double burden” may underlie the pathophysiology of hypertrophic scars. Added to that, wounds, grafts, and scar tissue can contract, which result in tension, contour abnormalities, and contractures. The presence of tension in the wound healing environment, except in the case of a keloid, is clinically associated with hypertrophic scarring in virtually every case unless the tension is eventually relieved by scar relaxation or surgical correction. Decreasing tension during the wound healing process has been shown to improve scar formation in both large animal and phase 1 studies.
Alteration in skin color is another major component to hypertrophic burn scars. Pigment alterations in burns scars derive from 2 main components: melanin (brown hues) produced by the melanocytes in the epidermis, resulting in hypopigmentation and/or hyperpigmentation, and oxyhemoglobin (red hues, erythema) by proliferating, dilated microvessels.
A plethora of nonsurgical therapies ranging from compression, silicone products, physical therapy, intralesional injections of corticosteroids, or 5-fluorouracil, and various laser treatments is part of the daily practice for scar rehabilitation, for which the evidence base has been more historical than scientific, but is widely accepted as standard care. Surgery remains the main reconstructive approach for contracted scars to release tension and increase the range of motion if it is limited. Nevertheless, although very effective in restoring range of motion, surgery is associated with high recurrence rates and the efficacy is often limited to the surgical site. Furthermore, a scar remains, which is often painful, pruritic, hypertrophic, atrophic, hyperpigmented, depigmented, erythematous with or without telangiectasia, abnormally textured, or all of the above in various areas of the same scar. Advances in laser therapy, especially the introduction of AFR, are a crucial part to fill the void between occupational therapy and surgical interventions. In some cases, AFR can even replace surgery, or be used before surgery to soften scars thus potentially improving surgical outcomes.
Laser Light for Burn Scar Modulation
Early evidence suggests that laser therapy can significantly improve the symptoms that are associated with hypertrophic scarring. Improvements in scar pigmentation, texture (ie, pliability and height), pain, and pruritus are reported benefits following laser therapy. Although many lasers have been applied for the treatment of hypertrophic scars, pulsed dye laser therapy (PDL) and AFR seem to offer burn patients the greatest benefit.
PDL is frequently used to correct erythema and pruritus and is thought to reduce scar redness by selective photothermolysis of blood vessels, thereby decreasing hypervascularity. Furthermore, targeted vascular destruction results in tissue hypoxia, collagen fiber heating, and catabolism, subsequently preventing excessive collagen deposition and leading to collagen realignment and remodeling. Because the PDL has limited penetration, it is less effective on thicker (>1.0 cm) hypertrophic scars, particularly if tension is present. Tension relieving, minimally morbid surgery, can facilitate the PDL’s effectiveness and may eliminate the need for scar excision ( Fig. 3 A–C). The exact mechanism by which PDL affects burn scar pruritus is not entirely clear, but changes in scar regulatory cytokines following laser treatment have been suggested.
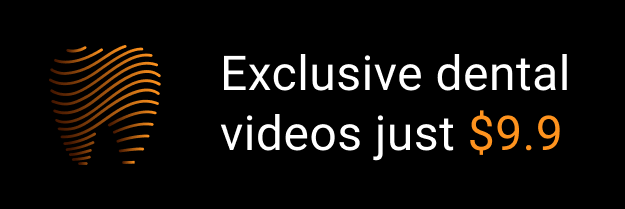