• Figure 17-1 A, Real-time OCT imaging prototype. B, Real-time OCT imaging with the handpiece probe. (A Courtesy Professor Anderson Zanardi de Freitas; B courtesy Dr. Petra Wilder-Smith.)
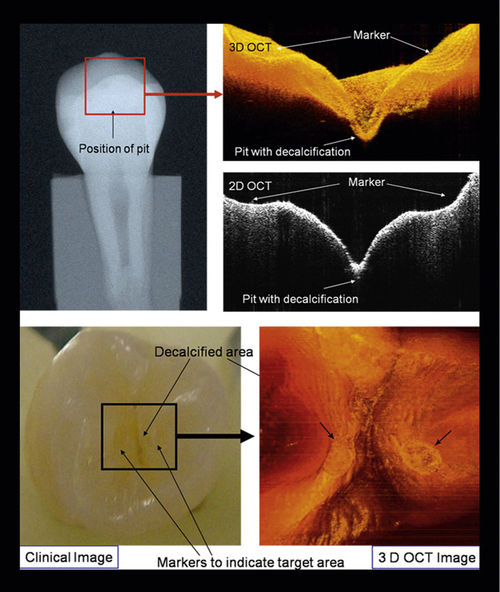
• Figure 17-2 Two-dimensional (2D) and three-dimensional (3D) OCT images with clinical intraoral photographs and x-ray images for comparison. (Courtesy Dr. Petra Wilder-Smith.)
Although OCT is not yet widely clinically available in dentistry, the technique promises fast technological development.8 Applying OCT to other clinically relevant biologic structures has been complicated because of optical scattering problems. Within the oral cavity, relevant biologic tissues typically are close to the surface, so OCT is a promising technique for obtaining images of human dental tissue in vivo and of carious lesions in particular.
Despite the significant gains in reducing its incidence, dental caries remains the principal cause of tooth loss worldwide. New treatment approaches emphasize early detection followed by minimal intervention. The most common approach to dental caries detection consists of dental radiography in conjunction with visual and tactile exploration.7 At present, however, these routine procedures are not precise enough to detect early lesions, especially in occlusal surfaces, or gaps at the tooth-restoration interface, which potentially lead to secondary caries.8 The early detection of restoration failures in the tooth-restoration interface could be the first step in preventing secondary caries formation and progression, as well as hypersensitivity of restored teeth, development of pulpal pathology, marginal staining, and the ultimate breakdown of a restoration.
Also, intraoral radiographs are highly sensitive and specific for the detection of primary caries but are less reliable for identifying recurrent caries around existing restorations.2 OCT offers a potentially more sensitive method for detecting recurrent caries. In 2005, de Araujo et al.8 showed the potential usefulness of OCT in clinical diagnosis compared with x-ray films. The 10-μm longitudinal resolution of the OCT system identified “induced” gaps. Conventional radiographs did not show the failure gaps. According to these authors, OCT has the advantage of showing the restored region as well as the gap, precisely localizing its position.
Amaechi et al.9 quantitatively assessed the mineral changes in a carious lesion based on the effectiveness of preventive measures to remineralize the lesion at an incipient stage. These researchers monitored the changes over time in the mineral status of the caries by using OCT with a system that could collect A-scans (depicting depth versus reflectivity curve), B-scans (longitudinal images), and C-scans (transverse images at constant depth). Bovine teeth were subjected to demineralization in acidic buffer solution for 3 days, with images obtained before demineralization and again after 3 days of demineralization. Whereas the B-scans and C-scans qualitatively described the lesion, the A-scans showed the depth (in mm)–resolved reflectivity (in dB) of the tooth tissue and were used for the quantitative analysis. The results showed that R (dB/mm) decreased with increasing demineralization time, and that the percentage reflectivity loss (R%) in demineralized tissue (amount of mineral loss) increased with increasing demineralization time, showing that OCT could quantitatively monitor the mineral changes in a carious lesion over the long term.
Fried and co-workers have spent more than 12 years studying and refining OCT, based on use of 1310-nm light for the detection of carious lesions in enamel, including the very problematic occlusal surfaces.10–13 Their early work showed the advantage of using polarization-sensitive OCT (PS-OCT) over nonpolarized OCT.10 PS-OCT gave much better resolution and imaging of early lesions in enamel and produced quantitative imaging as well as qualitative imaging of artificial and natural carious lesions.12,14 Numerous experiments were conducted in vitro that demonstrated quantitative assessment of demineralization and remineralization of enamel carious lesions using PS-OCT.12,15,16 The technique also can be applied to lesions in dentin and tooth roots for the assessment of demineralization and remineralization.17–21 Secondary caries can be imaged with PS-OCT.22 Of importance, quantitative assessment of carious lesions in vivo has recently been reported by this group of investigators, demonstrating that this methodology is very close to widespread use in clinical dentistry.23 At the same time, further refinements of the technique have been achieved, leading to the development of cross-polarization OCT (CP-OCT).24–28 CP-OCT has now been shown to be capable of monitoring carious lesion progress in human mouths.13,29
Prosthetic materials (e.g., metal, composites, ceramic fillings/crowns) also have been imaged with OCT, showing its potential advantage over conventional methods by visualizing structural and marginal restoration defects before significant leakage occurred, thereby minimizing tooth loss and decreasing the need for replacement restorations.2,7 Although accessibility of the probe tip to the area of interest is likely to be the limiting factor in this application, OCT also easily identifies marginal adaptation of the metal coping to the cavosurface margin and visualizes the internal aspects and marginal adaptation of porcelain and composite restorations.
In periodontics, because of visualization of the microstructural detail of the periodontal soft tissues, OCT offers the potential for identifying active periodontal disease before significant alveolar bone loss occurs. Visual recordings of periodontal tissue contour, sulcular depth, and connective tissue attachment are possible. OCT is a powerful method for generating high-resolution, cross-sectional images of oral structures, with in vivo imaging studies showing much more structural detail of dental tissues than obtainable with previous measurements.2,7
In endodontics, the OCT probe can be used to obtain a detailed microscopic image through the surrounding root canal circumferential wall to the outside cementum layer of the root.30 The probe also can image the anatomy and assess the cleanliness of the canal walls and measure the exact thickness of the dentinal wall, which helps prevent canal overpreparation and perforation of the canal walls. OCT also could prove extremely useful in the diagnosis of vertical fractures.31
OCT has potential applications in dentistry as a noninvasive method for imaging dental microstructure. The cross-sectional images exhibit microstructural details that cannot be obtained with other current imaging modalities. Using this new technology, visual recordings of the detection of very early demineralization and remineralization processes, tooth defects and restorative failures, periodontal disease, soft tissue dysplasias, precancerous lesions, and root canal anatomy can be obtained. OCT is a diagnostic aid that could meet the dental challenges of prevention and early intervention.
Widespread availability of OCT for clinical use can be anticipated in the near future. This technique tracks carious lesion progress or remineralization using infrared light and no ionizing radiation, meaning that high-caries patients could be monitored frequently as therapeutic interventions are carried out, without any concerns regarding excess radiation exposure.
Photoactivated Disinfection and Microbial Reduction
Reduction in levels of microorganisms is the main goal of various procedures in daily dental practice, especially in the treatment of root canal and periodontal tissue. Protocols that provide significant microbial reduction have established this modality as a coadjutant to the treatment of dental infections, especially in patients with resistant microorganisms and anatomic complications.
The temperature increase resulting from high-intensity laser irradiation can cause protein denaturation and can destroy microorganisms, with high decontamination indexes.32 Low-level laser therapy (LLLT) is not capable of increasing tissue temperature,33 so the same antimicrobial effects of the high-intensity laser cannot be expected when LLLT is used as the sole clinical modality.34 Despite this limitation, low-intensity lasers have been studied and introduced clinically for microbial reduction. Their antimicrobial effect is achieved using the combination of low-power lasers with extrinsic photosensitizers, which results in highly reactive oxygen species (ROS).35 These cause damage to cell membranes, mitochondria, and DNA,36–38 and microbial destruction is inevitable. This process is called photoactivated disinfection (PAD), also called photodynamic therapy (PDT), photochemotherapy, and lethal photosensitization (see also Chapter 15).
The antimicrobial capacity of PAD has been used to improve microbial reduction during conventional therapy in periodontics, endodontics, restorative dentistry, and implantology.39–42 Viral inactivation and successful treatment in herpes simplex virus type 1 (HSV-1) infection also have been reported.43
Photoactivated disinfection presents various advantages over traditional antimicrobial agents. PAD promotes faster microbial killing, without the need to maintain higher concentrations of photosensitizer in the infected area, as with antiseptics and antibiotics.44 The main advantage is attributed to its local action; PAD affects microorganisms at the site of photosensitizer deposition exclusively, whereas systemic drugs exert their actions throughout the body.45 Moreover, PAD does not damage or alter adjacent structures, such as periodontal and periapical tissue, even when higher concentrations of photosensitizer and higher energy densities are used.46
For the effective treatment of bacterial infections, it is paramount to have an adequate light source and a photosensitizer capable of binding to the target pathogen, so that photosensitization may occur in either subgingival or superficial oral tissues. The most frequently used light source for photosensitization in dentistry is the low-power laser because it (1) presents a narrow spectral band that enables a more specific interaction with photosensitizers, (2) can be coupled to optical fibers, and (3) does not cause increased tissue temperatures, as observed with polychromatic light sources.47,48 The use of light-emitting diodes (LEDs) for this application also has been reported.49
Several photosensitizers are available for PAD; however, disinfection of oral pathogens generally necessitates use of cationic-charged photosensitizers, such as toluidine blue, methylene blue, and poly-L-lysine-chlorin-(e6) conjugates.50,51 Interaction between photosensitizers and microorganisms occurs within a few minutes, and this period (incubation or preirradiation time) must be respected before laser irradiation begins.35,51
Disadvantages of PAD include lack of standardization and absence of an established protocol. Researchers have only started to evaluate the antimicrobial action of PAD; therefore much remains to be elucidated regarding the ideal light source, the most adequate photosensitizer for each type of bacteria and target tissue, and the proper energy density and power settings. Nevertheless, protocols adopted from in vitro and in vivo studies have presented safe and favorable scientific results that already enable the clinical application of PAD.
Recent studies by Feuerstein and co-workers have reported effects of blue light on biofilm formation. Two major conclusions have come from this laboratory work, and the results are yet to be confirmed in vivo. First, exposure of Streptococccus mutans in biofilm to blue light affected the re-formation of a new biofilm, showing an increase in the amount of dead bacteria.52 This phenomenon suggests that blue light has a delayed antibacterial effect, although it does not interfere with bacterial capability to re-form an initial biofilm. Second, earlier work showed that blue light coupled with hydrogen peroxide has a major antibacterial effect on biofilm.53,54 An antibacterial synergic effect between blue light and hydrogen peroxide was observed. The mechanism of the phototoxic effect on S. mutans was basically a photochemical process involving ROS. Application of such light in combination with hydrogen peroxide to an infected tooth could be an alternative to or serve as an additional minimally invasive antibacterial treatment.
Influence of Pulse Duration on High-Intensity Laser Application
High-intensity lasers frequently are used in the daily practice of dentistry. The variety of wavelengths and their interaction with the different chromophores allow their application for many purposes, in either oral soft or hard tissues. The results depend on numerous parameters, including wavelength, pulse energy, fluence (joules per square centimeter), pulse duration, and repetition rate. Laser ablation of hard tissue and selective removal of dental caries have attracted attention because these are considered safe procedures55 that reduce pain,56,57 without the noise and vibrations of a conventional drill.58
Laser interaction with dental hard tissue may result in efficient and safe removal of compromised dental structure. Achieving this aim will require full awareness of those factors and technical skills involved in limiting lateral thermal and mechanical damage and in optimizing final surface characteristics.
Recent study has focused on the influence of pulse duration on the ablation process. The development of high-technology laser devices now allows the selection of pulse duration in the range of microseconds (1μsec = one millionth [0.000001] of a second), nanoseconds (1 nsec = one billionth [10−9] of a second), and lately, picoseconds (1 psec = one trillionth [10−12] of a second) and femtoseconds (1 fsec = one quadrillionth [10−15] of a second). The equipment includes different wavelengths in ultraviolet, visible, and infrared regions of the electromagnetic spectrum, such as 2940 nm (erbium-doped yttrium-aluminum-garnet [Er:YAG] laser in free-running and Q-switched modes), 9300 nm (TEA [transverse excited atmospheric] flowing gas CO2 laser), 9600 nm (CO2 and TEA CO2 lasers), 10,600 nm (CO2 laser), 308 nm (xenon monochloride [XeCI] laser), 2780 nm (erbium plus chromium–doped yttrium-scandium-gallium-garnet [Er,Cr:YSGG] laser), 1064 nm (neodymium-doped yttrium-aluminum-garnet [Nd:YAG] laser with regenerative amplifier [RGA] system), and 425 nm (low-power visible femtosecond laser).59–63
Considering that the increase in temperature and the peripheral thermal damage may provoke fracture, cracking, structural breakdown, or melting in dental hard tissue64–67 and healing inhibition and necrosis in bone, it is assumed that high-power pulses, with pulse durations shorter than the thermal relaxation time, are necessary to avoid thermal denaturation of the tissues adjacent to the irradiated surfaces.61,68 Longer pulse durations are thought to be responsible for inducing damage and thermomechanical stress to tissue, because they allow thermal energy to accumulate and penetrate deeply.69
Studies on pulse duration in the ablation process indicate that not only does the threshold energy for ablation decrease,69 but that the morphologic shapes of the crater are altered when ultrashort pulses are used.70–72 Although lasers with ultrashort (fsec) pulse duration are being developed, pulses in the microsecond range are already considered to be extremely short.73 The tissue relaxation time for enamel is 100 μsec, and supershort pulse (SSP) durations (50 μsec) are already sufficient for precise ablation.59 Pulses with 100-μsec duration (very short pulses [VSPs]) are considered the standard for routine work, as well as short pulses (SPs), of 300 μsec. Pulse durations of 700 μsec (long pulses) and 1000 μsec (very long pulses) are indicated for soft tissues because the residual thermal energy provides coagulation. Consequent to the reduction in pulse duration to picoseconds and nanoseconds, studies are now using wavelengths not previously used for dental tissue ablation (e.g., Nd:YAG lasers).74–77
Use of shorter pulse duration and higher energy intensity accelerates the ablation process.78 This acceleration results from the quicker vaporization of the water present in the irradiated tissue, which causes the rapid microexplosion of the water molecules and removal of hard tissue structures.79,80 In this case, ablation efficacy is improved; reduced residual thermal damage is induced because of the minimization of heat diffusion; enamel acid resistance to caries is increased81,82; and less vibration is provoked. The result is a reduction in painful stimulus to the pulp and greater patient comfort and treatment acceptance.83
Carbon Dioxide Lasers
Overview
With all CO2 lasers, the active laser medium is a gas mixture that contains CO2, helium (He), nitrogen (N2), and possibly some hydrogen (H2), water vapor, and xenon (Xe). Such lasers are electrically pumped by gas discharge. N2 molecules are excited by the discharge into a metastable vibrational level and transfer their excitation energy to the CO2 molecules when they collide. Helium serves to depopulate the lower laser level and to remove the heat. Hydrogen and water vapor can help to reoxidize carbon monoxide (CO) formed in the discharge to CO2. These lasers typically emit at a wavelength of 10.6 μm (10,600 nm), but they also can emit as several wavelengths in the region of 9 to 11 μm—specifically, 9.3, 9.6, 10.3, and 10.6 μm.
Laser systems such as the CO2 permit very-high-energy radiation to be focused on a tiny spot and have found applications in many dental specialties. For dental applications, all CO2 lasers are used in a noncontact technique and can be operated in continuous-wave or pulsed-beam mode.84
Laser Types and Applications in Dentistry
The three main CO2 laser wavelengths that have been researched and/or used in dental procedures are 9.3, 9.6, and 10.6 μm (9300, 9600, and 10,600 nm). Even with such similar wavelengths, the absorption by biologic tissues is different, so the clinical applications may vary. Dental mineral tissues (enamel and dentin) have weak absorption in the visible (400 to 700 nm) and near-infrared (1064 nm) spectrum.85,86 CO2 lasers are well absorbed by biologic tissues because of their strong affinity for water and, in the case of the 9.3- and 9.6-μm wavelengths, interact strongly with apatite absorption bands, mainly with phosphate and carbonate groups. Therefore the 9.3- and 9.6-μm CO2 laser wavelengths can be used for procedures involving both hard and soft tissues. The absorption coefficients in dental enamel for 9.3, 9.6, and 10.6 μm are 5500, 8000 and 825 cm−1, respectively,87 compared with the Nd:YAG wavelength (1.06 μm), which has an absorption coefficient in enamel of approximately 1 cm−1. These data mean that the 9.3- and 9.6-μm wavelengths are extremely well absorbed in dental mineral, causing very efficient localized heating88 as compared with the conventional 10.6-μm laser that is absorbed at one tenth of the efficiency.
9.3- and 9.6-μm Carbon Dioxide Lasers
Results of using the 9.3-μm and 9.6-μm CO2 laser wavelengths on hard tissues (enamel and dentin) show promising clinical applications for this wavelength.89,90
Many studies have been published over the past 20 years or so, and only some are highlighted here. Studying the effects of CO2 lasers on dental enamel morphology, McCormack et al.89 demonstrated that surface changes can be produced at low fluences if the hard tissues efficiently absorb the wavelengths used. Exposure included an extensive range of CO2 laser wavelengths (9.3, 9.6, 10.3, and 10.6 μsec), with 5, 25, or 100 pulses at absorbed fluences of 2, 5, 10, or 20 J/cm2 and pulse widths of 50, 100, 200, or 500 μsec. Longer pulses at constant fluence conditions decreased the extent of surface melting and crystal fusion, and the total number of laser pulses delivered to the tissue did not significantly affect surface changes so long as at least 5 to 10 pulses were used. Within the wavelengths of the CO2 laser, differences in the observed surface changes of the enamel were dramatic. On dentin, the effects of CO2 laser at 9.3 μm showed no craters or cracks but included many small, molten and rehardened particles on the surface, suggesting that laser irradiation affected only the dentin surface (<20 μm) and would be less harmful to dental pulp for dentin ablation.91
Both 9.3-μm and 9.6-μm CO2 lasers have been extensively studied for caries prevention. Several studies in the past three decades have demonstrated the potential of laser pretreatment of enamel or tooth roots to inhibit subsequent acid-induced dissolution or artificial caries-like challenges in vitro.92–94 The overall objective is to determine the optimum parameters for CO2 laser irradiation that will effectively inhibit dental caries (primary and secondary) in enamel and dentin. Featherstone et al.92 reported that the rate of CO2 laser inhibition of artificial caries-like lesions in dental enamel ranged from 40% to 85% for all laser conditions tested, comparable to inhibition with daily fluoride dentifrice treatments, with minimal subsurface temperature elevation (<1° C at 2-mm depth).
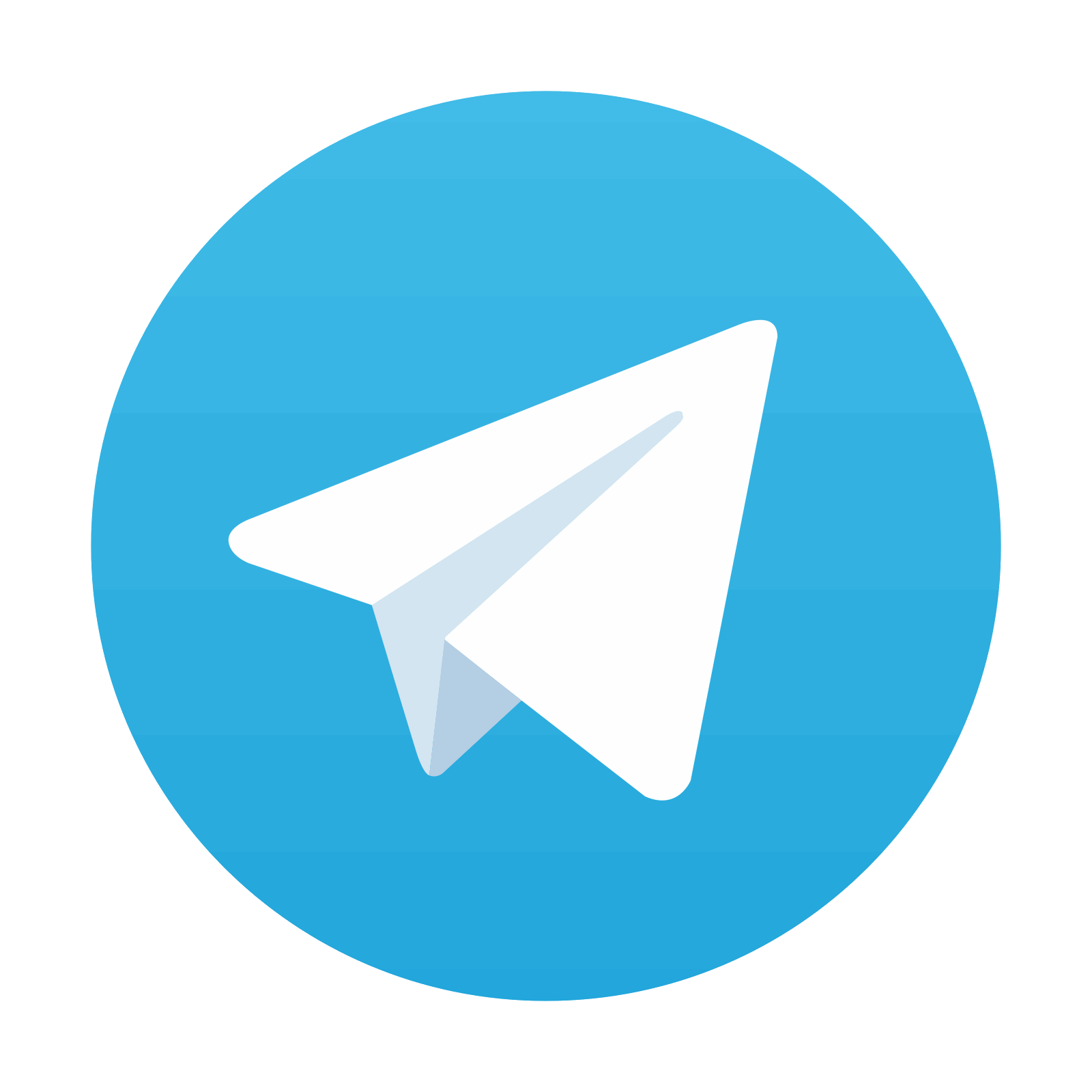
Stay updated, free dental videos. Join our Telegram channel

VIDEdental - Online dental courses
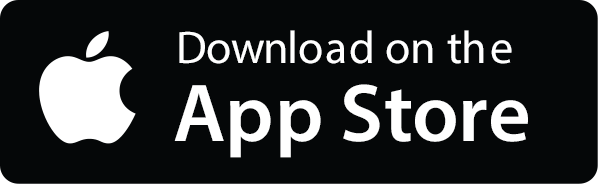
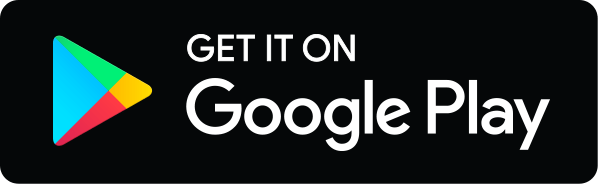