Abstract
Objective
Monomer development for a reduced shrinkage of composite materials still challenges the modern research. The purpose of this study was to analyse the shrinkage behavior of an innovative composite material for dental restorations based on a resin system that is claimed to control polymerization kinetics having incorporated a photoactive group within the resin.
Methods
Shrinkage stress development within the first 300 s after photoinitiation, gel point as well as micro-mechanical properties (Vickers hardness HV, modulus of elasticity E , creep Cr and elastic–plastic indentation work W e / W tot ) were evaluated ( n = 10). The experimental flowable resin-based composite (RBC) was measured in comparison to regular methacrylate-based micro- (Esthet X Flow) and nano-hybrid flowable RBCs (Filtek Supreme Plus Flow). Additionally, the high viscosity counterparts of the two regular flowable methacryate-based composites (Esthet X Plus and Filtek Supreme Plus) as well as a low shrinkage silorane-based micro-hybrid composite (Filtek Silorane) were considered. The curing time was 20 s (LED unit Freelight2, 3M-ESPE, 1226 mW/cm 2 ).
Results
The experimental material achieved the significantly lowest contraction stress (1.1 ± .01 MPa) followed by the silorane-based composite (3.6 ± .03 MPa), whereas the highest stress values were induced in the regular methacrylate-based flowable composites EsthetX Flow (5.3 ± .3 MPa) and Filtek Supreme Flow (6.5 ± .3 MPa). In view of gel point, the best values were obtained for the experimental flowable composite (3.1 ± .1s) and Filtek Silorane (3.2 ± .3 s), which did not differ significant from each others, whereas EsthetX Plus and Filtek Supreme Plus did also not differ significantly, inducing the shortest gel point. The experimental flowable material achieved also the lowest shrinkage-rate (maximum at 0.1 MPa/s). For all analysed materials, no significant difference in the micro-mechanical properties between top and bottom were found when measured on 2 mm thick increments 24 h after polymerization. The categories of flowable materials performed in the measured micro-mechanical properties significantly inferior when compared to the hybrid-composites, showing lower HV and E and predominantly higher creep and plastic deformation. Within the flowable RBCs, the experimental material achieved the lowest Vickers hardness, the highest modulus of elasticity, the highest creep and showed the significantly lowest elastic deformation.
Significance
The experimental flowable composite revealed the lowest shrinkage stress and shrinkage-rate values in comparison to regular methacrylate composites but intermediate micro-mechanical properties. Being at the same time more rigid (higher modulus of elasticity) and more plastic (low W e / W tot and high creep values) as the regular flowable materials, its effect on interfacial stress build-up cannot be easily predicted.
1
Introduction
Several negative effects in resin-based composite (RBCs) restorations, like marginal discrepancies , marginal staining, white lines around the restoration, cusps fractures , microleakage , debonding , recurrent caries, post-operative sensitivity or pain, are frequently connected to polymerization shrinkage stress. Even if these assertions are nowadays broadly accepted, only little clinical evidence exists to support a clear relationship between this negative outcomes and polymerization shrinkage stress . Nevertheless, new materials having reduced internal stress as a result of low polymerization shrinkage are predicted to dominate the market in the future, even before a complete understanding of the clinical effect of shrinkage was achieved .
As a consequence, extensive efforts have been invested over years to develop low shrinkage dental restorative materials. Beside changes in filler amount, shape or surface treatment, changes in monomer structure or chemistry and modification of dynamics of the polymerization reaction are the most promising approaches.
The changes in monomer chemistry were at first directed to improve the already clinical successful methacrylate-based systems, by modifying the Bowen monomer (Bis-GMA: 2,2-bis[4-(2-hydroxy-3-methacrylyloxypropoxy)phenyl]propane) to create monomers with lower viscosity like hydroxyl free Bis-GMA , aliphatic urethane dimethacrylates (UEDMA: 1,6-bis[methacrylyloxy-2-ethoxycarbonylamino]-2,4,4-trimethylhexane) , partially aromatic urethane dimethacrylate or highly branched methacrylates . But also ring-opening systems for non- or minimally shrinking dental composites like spiro-orthocarbonates as additives to dimethacrylates and epoxy-base resins like the siloranes as well as ormocers (organically modified ceramics) were introduced on the market for the same purpose.
Recent trials to modify the dynamics of the polymerization reaction by incorporating a photoactive group in a urethane-based methacrylate resin showed a 60–70% reduction in shrinkage stress in the unfilled resin when compared to conventional methacrylate-based resins . The activated resin demonstrated a relatively slow radical polymerization rate, suggesting that the photoinitiator incorporated into the resin is affecting the radical polymerization process. This lower curing stress was shown to be retained also in filled compositions, particularly in cases of low filler-loading . These resins are patent-registered as being based on the SDR™ technology (=stress decreasing resin).
To additionally minimize shrinkage stress at the interface tooth–composite for a given composite material, several practical steps are proposed, most of them being related to the chosen cavity configuration ( C -factor) , the method of cavity reconstruction , the selection of the curing method , or the introduction of stress absorbing intermediate layers . Positive effects are reported by using flowable composites as stress absorbing intermediate layers. Used in Class II restorations together with packable (Filtek P60) or nanofilled composites (Filtek Supreme XT Universal Restorative), less microleakage was measured . As additionally positive effects, flowable liners are considered to decrease sensitivity and to wet the cavity better than restorative composites due to their flowability, to have thus a better adaptation to the dentinal surface, with fewer voids at the interface of the restoration and tooth structure, when compared with bonding agent and resin composites alone . Reducing shrinkage during polymerization in flowable composite liners will be an additional positive effect.
It was claimed that resin systems based on the SDR™ technology with a polymerization modulator being chemically embedded in the polymerizable resin backbone controlling thus polymerization kinetics will induce lower polymerization shrinkage in the flowable composite based on it .
The aim of this study was therefore to analyse the shrinkage behavior and the micro-mechanical properties of an experimental flowable composite based on this technology, developed to serve as a flowable liner, in comparison to regular methacryate-based micro- and nano-hybrid flowable composites. Additionally, the high viscosity counterparts of the two regular flowable methacryate-based composites as well as a low shrinkage silorane-based micro-hybrid composite were considered.
The null hypotheses tested were:
- 1.
The shrinkage behavior of the experimental flowable material during polymerization do not differ from the regular flowable materials.
- 2.
A reduced final shrinkage stress will not lower the micro-mechanical properties.
2
Materials and methods
An experimental flowable composite material (Exp-Flow, Dentsply) was analysed in comparison to four commercially available methacrylate-based micro- and nano-hybrid composites, two of them being flowable composites, as well as with an silorane-based micro-hybrid composite ( Table 1 ). The material’s behavior was assessed by evaluating the shrinkage stress development during curing, shrinkage stress values yielded thereof and gel point as well as the micro-mechanical properties – Vickers hardness, modulus of elasticity, creep and elastic–plastic deformation – after polymerizing the materials for 20 s with a LED-curing unit (Freelight 2, 3M-ESPE, Germany). The spectral distributions and irradiance of the tested curing unit were determined by means of a calibrated fibre optic spectrally resolving radiometer equipped with an integrating sphere (S2000, Ocean Optics, USA). The total irradiance was obtained by integrating the irradiance as a function of the wavelength over the entire wavelength range, divided by the effective area of the curing unit tip. The diameter of the tip was measured with a digital micrometer, whereby as effective area was defined the area of the tip without cladding.
Composite | Manufacturer | Type | Resin matrix | Filler |
---|---|---|---|---|
EsthetX plus LOT:0705114 | Dentsply | Micro-hybrid | Bis-GMA adduct | Ba–F–Al–B–Si–glass nanofiller silica 77 wt%, 60 vol% |
Bis-EMA adduct | ||||
TEGDMA | ||||
EsthetX Flow LOT:070215 | Dentsply | Flowable Micro-hybrid | Bis-GMA adduct | Ba–F–Al–B–Si–glass nanofiller silica 61 wt%, 53 vol% |
Bis-EMA adduct | ||||
TEGDMA | ||||
Exp-Flow LOT:QD2-161 | Dentsply | Flowable | Modified UDMA | Ba–Al–F–B–Si–glass, St–Al–F–Si–glass 68 wt%, 44 vol% |
EBPADMA | ||||
TEGDMA | ||||
Filtek Supreme Plus LOT:A2B 20070809 | 3M-ESPE | Nano | BIS-GMA | Silica nanofiller, zirconia/silica nanocluster, 78.5 wt%, 59.5 vol% |
BIS-EMA | ||||
UDMA | ||||
TEGDMA | ||||
Filtek Supreme Plus Flow LOT:A2B20070716 | 3M-ESPE | Flowable Nano | BIS-GMA | Silica nanofiller, zirconia nanofiller zirconia/silica nanocluster, 65 wt%, 55 vol% |
BIS-EMA | ||||
TEGDMA | ||||
Filtek Silorane LOT:20070827 | 3M-ESPE | Micro-hybrid | 3,4-Epoxycyclohexylethylcyclo-polymethylsiloxane | Silanized quartz yttrium fluoride 76 wt%, 55 vol% |
Bis-3,4-epoxycyclohexylethyl-phenylmethylsilane |
2.1
Shrinkage stress measurement device
Shrinkage stress and time until gelation (gel point) of the tested materials were investigated with a stress–strain analyzer (SSA T80, Engineering Consultancy Peter Dullin Jr, Munich) . With due compensation for machine compliance, this device measured the contraction stress generated by polymerizing the restorative material with the light exposure mentioned above ( n = 10).
2.1.1
Experimental setup
Composite specimens were placed between two aluminum attachments with a distance of 4 mm and a height of 2 mm. One attachment was connected to a load sensor, and the other to a piezo actuator. A PTFE coated plastic tray was mounted on the testing machine, fitting exactly between the two attachments. The two opposing attachments together with the plastic mould simulate a 4 mm × 4 mm × 2 mm cavity with a C -factor of 0.3 corresponding clinically to a premolar restoration with an increment of 2 mm. The functional surfaces of the two attachments were cleaned with Rocatec Pre (ESPE), coated with Rocate Plus (ESPE), followed by applying a silane coupling agent (ESPE-Sil, ESPE). Experimental composite was then applied in one 2 mm increment.
2.1.2
Measurement procedure
Contraction force generated due to composite polymerization was continuously measured and recorded for 300 s after photoinitiation. Each experiment was conducted at room temperature and repeated ten times for each material. Contraction stress was calculated as contraction force divided by the contact area (=8 mm 2 ). Maximum contraction stress during recording time and time needed to exceed a force threshold of 0.5 N (arbitrarily defined as the time until gelation or gel point) were compared using one-way ANOVA and Tukey’s HSD post hoc test ( α = 0.05) (SPSS 17.0).
2.2
Micro-mechanical properties
To analyse the mechanical properties of the materials, measurements were made with a micro-hardness indenter (Fischerscope H100C, Fischer, Sindelfingen, Germany) according to DIN 50359-1:1997-10 . Prior to testing, the samples were polished with a diamond suspension (mean grain size: 1 μm). Measurements were done at the top and bottom of the materials after storing the samples made for the shrinkage-stress measurements (4 mm × 4 mm × 2 mm) for 24 h in distilled water ( n = 10, with 5 measurement per sample).
The test procedure was carried out force controlled; the test load increased and decreased with constant speed between 0.4 mN and 500 mN. The load and the penetration depth of the indenter were continuously measured during the load–unload-hysteresis. The Universal hardness is defined as the test force divided by the apparent area of the indentation under the applied test force. From a multiplicity of measurements, a conversion factor between Universal hardness and Vickers hardness was calculated and implemented in the software, so that the measurement results were indicated in the more familiar Vickers hardness units. The indentation modulus was calculated from the slope of the tangent of indentation depth-curve at maximum force and is comparable with the modulus of elasticity of the material. By measuring the change in indentation depth with constant test force, a relative change in the indentation depth can be calculated. This is a value for the creep of the materials. The mechanical work W tot indicated during the indentation procedure is only partly consumed as plastic deformation work W plast . During the removal of the test force the remaining part is set free as work of the elastic reverse deformation W elast . According to the definition of the mechanical work as W = ∫ F dh , W tot = W elast + W plast . The parameter W e / W tot [%] representing the percentage of elastic work reported to the total mechanical is indicated in the results data.
2.3
Statistical analysis
Results were statistically compared using one-way ANOVA and Tukey HSD post hoc-test ( α = 0.05) as well as a Pearson correlation analysis. A multivariate analysis (general linear model with partial eta-squared statistics) tested the influence of the parameters filler volume and weight as well as top-bottom indicating the measurement location on the considered properties (SPSS 18.0).
2
Materials and methods
An experimental flowable composite material (Exp-Flow, Dentsply) was analysed in comparison to four commercially available methacrylate-based micro- and nano-hybrid composites, two of them being flowable composites, as well as with an silorane-based micro-hybrid composite ( Table 1 ). The material’s behavior was assessed by evaluating the shrinkage stress development during curing, shrinkage stress values yielded thereof and gel point as well as the micro-mechanical properties – Vickers hardness, modulus of elasticity, creep and elastic–plastic deformation – after polymerizing the materials for 20 s with a LED-curing unit (Freelight 2, 3M-ESPE, Germany). The spectral distributions and irradiance of the tested curing unit were determined by means of a calibrated fibre optic spectrally resolving radiometer equipped with an integrating sphere (S2000, Ocean Optics, USA). The total irradiance was obtained by integrating the irradiance as a function of the wavelength over the entire wavelength range, divided by the effective area of the curing unit tip. The diameter of the tip was measured with a digital micrometer, whereby as effective area was defined the area of the tip without cladding.
Composite | Manufacturer | Type | Resin matrix | Filler |
---|---|---|---|---|
EsthetX plus LOT:0705114 | Dentsply | Micro-hybrid | Bis-GMA adduct | Ba–F–Al–B–Si–glass nanofiller silica 77 wt%, 60 vol% |
Bis-EMA adduct | ||||
TEGDMA | ||||
EsthetX Flow LOT:070215 | Dentsply | Flowable Micro-hybrid | Bis-GMA adduct | Ba–F–Al–B–Si–glass nanofiller silica 61 wt%, 53 vol% |
Bis-EMA adduct | ||||
TEGDMA | ||||
Exp-Flow LOT:QD2-161 | Dentsply | Flowable | Modified UDMA | Ba–Al–F–B–Si–glass, St–Al–F–Si–glass 68 wt%, 44 vol% |
EBPADMA | ||||
TEGDMA | ||||
Filtek Supreme Plus LOT:A2B 20070809 | 3M-ESPE | Nano | BIS-GMA | Silica nanofiller, zirconia/silica nanocluster, 78.5 wt%, 59.5 vol% |
BIS-EMA | ||||
UDMA | ||||
TEGDMA | ||||
Filtek Supreme Plus Flow LOT:A2B20070716 | 3M-ESPE | Flowable Nano | BIS-GMA | Silica nanofiller, zirconia nanofiller zirconia/silica nanocluster, 65 wt%, 55 vol% |
BIS-EMA | ||||
TEGDMA | ||||
Filtek Silorane LOT:20070827 | 3M-ESPE | Micro-hybrid | 3,4-Epoxycyclohexylethylcyclo-polymethylsiloxane | Silanized quartz yttrium fluoride 76 wt%, 55 vol% |
Bis-3,4-epoxycyclohexylethyl-phenylmethylsilane |
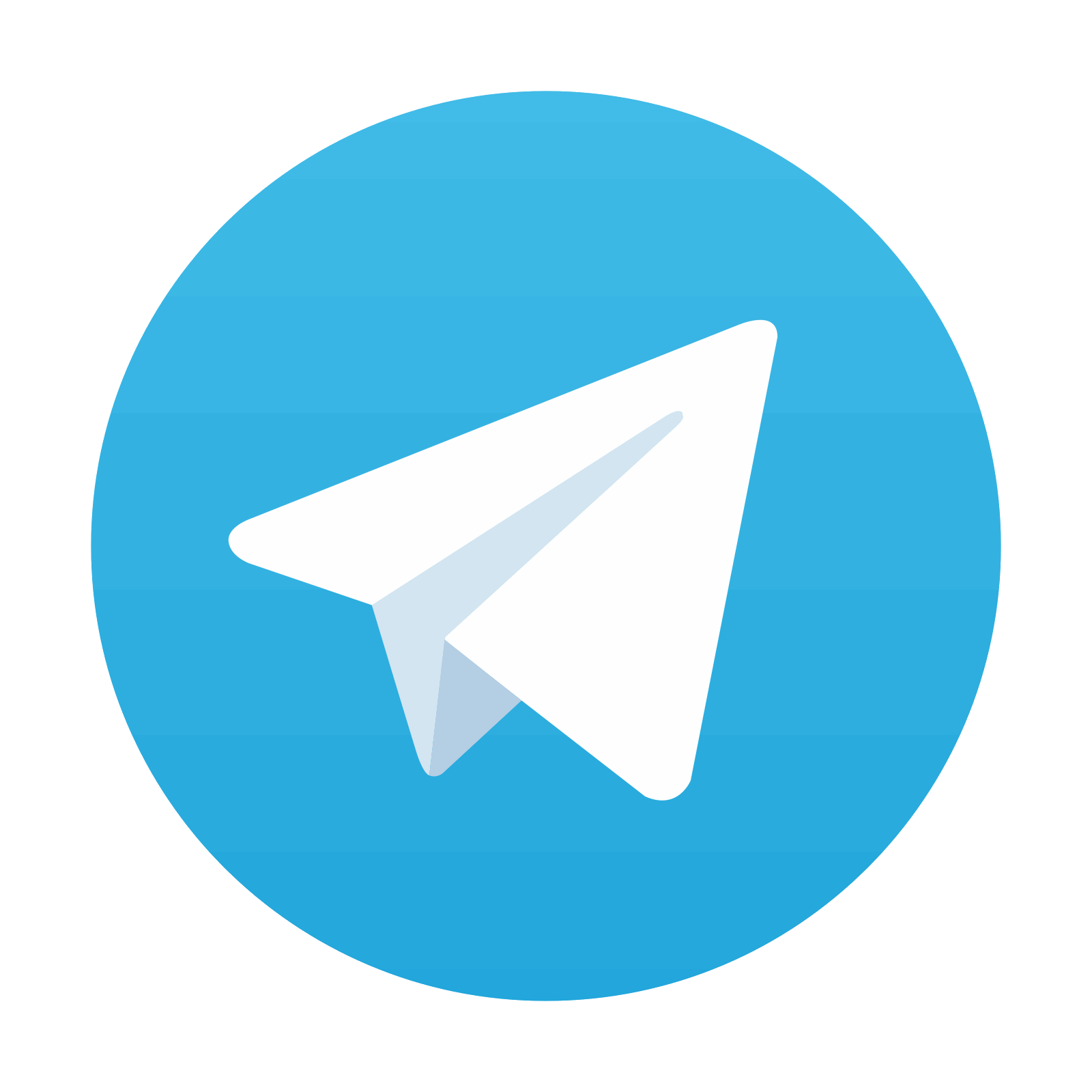
Stay updated, free dental videos. Join our Telegram channel

VIDEdental - Online dental courses
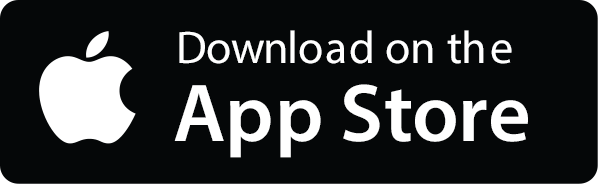
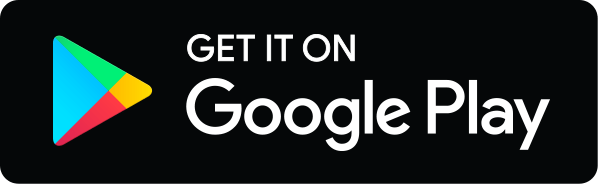
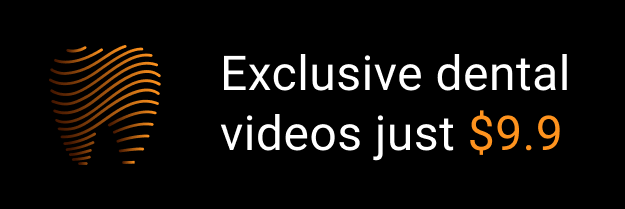