Abstract
Objectives
This study was designed to evaluate the effect of an increase of fiber-density on some mechanical properties of higher volume fiber-reinforced composite (FRC).
Methods
Five groups of FRC with increased fiber-density were fabricated and two additional groups were prepared by adding silanated barium-silicate glass fillers (0.7 μm) to the FRC. The unidirectional E-glass fiber rovings were impregnated with light-polymerizable bisGMA-TEGDMA (50–50%) resin. The fibers were pulled through a cylindrical mold with an opening diameter of 4.2 mm, light cured for 40 s and post-cured at elevated temperature. The cylindrical specimens ( n = 12) were conditioned at room temperature for 2 days before testing with the three-point bending test (Lloyd Instruments Ltd.) adapted to ISO 10477. Fiber-density was analyzed by combustion and gravimetric analyzes.
Results
ANOVA analysis revealed that by increasing the vol.% fraction of E-glass fibers from 51.7% to 61.7% there was a change of 27% ( p < 0.05) in the modulus of elasticity, 34% ( p < 0.05) in the toughness, and 15% ( p < 0.05) in the load bearing capacity, while there was only 8% ( p < 0.05) increase in the flexural strength although it was statistically insignificant. The addition of particulate fillers did not improve the mechanical properties.
Significance
This study showed that the properties of FRC could be improved by increasing fibervolume fraction. Modulus of elasticity, toughness, and load bearing capacity seem to follow the law of ratio of quantity of fibers and volume of the polymer matrix more precisely than flexural strength when high fiber-density is used.
1
Introduction
The development of biomaterials has focused on composites with properties that can be tailored for biomedical needs. One such biomaterial, fiber-reinforced composites (FRC), began to be used in the late 1990s. One rationale for the use of FRCs in dentistry was their mechanical properties, which correspond to those for dentin and bone, while others are related to the adhesional characteristics of the FRC. The mechanical properties can be optimized to be equal of those of dentin or bone . The use of FRC has increased in dental applications such as removable prosthodontics, fixed partial dentures (FPD), periodontal splints, and in orthodontic treatment as a retention splint . Recently, attempts have been made to use FRC as implant material in dental, orthopedic, and craniofacial surgeries . Although, there is a limited amount of scientific literature on using FRC material as surgical devices, they show potential for their use in surgery, especially when combined with bioactive modifiers, such as bioactive glass.
The rigidity and strength of prostheses made from FRC are dependent on the polymer matrix of the FRC and the type of fiber reinforcement. The reinforcing capacity of the fibers depends on the orientation, quantity, adhesion of the fibers to the polymer matrix resin, and impregnation of the fibers with the matrix resin . Among different high performance fibers, glass fibers seem to be the fibers of choice in dental applications because of the good adhesion of silanated glass fibers to mono- and dimethacrylates and because of good esthetic properties.
Effective wetting of fibers by the resin matrix, also called resin impregnation, is a prerequisite for their effective use . Optimal reinforcement and transfer of stresses from the polymer matrix to reinforcing fibers may be achieved with good resin impregnation. An improper degree of impregnation causes increasing water sorption through voids, leading to reduced mechanical properties of FRC . Furthermore, voids of poorly impregnated fibers are oxygen reservoirs , which inhibit the radical polymerization of the polymer matrix. This also decreases the strength of the FRC and increases the residual monomer content, which can lead to irritant reactions in the oral mucosa .
In the case of photopolymerization of FRC, the light intensity, exposure time, and polymerization temperature have an effect on flexural properties and monomer conversion .
Numerous articles have demonstrated the relationship between the quantity of fibers in the polymer matrix and the enhancement of the flexural, transverse, and impact strength of fiber-reinforced reconstructions . It has been shown that with increasing fiber content, the flexural strength increases linearly . The fiber quantity in the polymer matrix should be defined in volume not weight percentage. In the case of carbon-, aramid- or ultra-high-modulus polyethylene fibers (UHMPE), which have a lower density than glass fibers, the fiber content can lead to misleading results with regard to the strength of FRC . Vallittu has described a formula for transforming fiber weight percentage into volume percentage .
Furthermore, fibers are mechanically more effective in achieving a durable and stiff composite than particulate fillers, and with the aid of fibers, the load-bearing capacity of the material can be increased. Unidirectional fiber-reinforced composite has relative strength and stiffness comparable to metal when loading along the fibers, but with much less weight. Because of the anisotropic nature of unidirectional FRC, the material has different physical properties in different directions, thus designing the FRC device should be done carefully. Unidirectional fibers can most effectively reinforce the composite when positioned on the tension side . When the fiber content in the composite increases, the strength and modulus of elasticity of the composite also increase .
A subtle and under-reported attribute of FRC is fracture failure. A specimen may catastrophically fail instantaneously while others may simply bend under increasing load. In 1975, Craig and Courtney described three modes of failure that can occur when characterizing FRC in a tension type test . The three failure modes are described as instantaneous, statistical, and stepwise. Instantaneous failure occurs after a load causes a strain concentration in a narrow region sufficient to break the composite structure. A strain concentration distributed to a wide region may require further load or elongation for continued fracture, thus leading to what is denoted as stepwise (more bending type) or statistical failure (a series of small intense fractures which recover before complete failure and require more load to progress). Analysis of the mode of failure can give insight on how and why failure occurs. Accurate reporting should, therefore, include information on when the fracture begins (initial fracture), how it progresses, and when it finishes (final fracture) .
The mechanical properties of unidirectional FRC follow the law of ratio of quantity of fibers and volume of the polymer matrix. However, there is a lack of information on the mechanical behavior of FRC at higher fiber densities than used in present FRCs. This study was designed to evaluate the effect of an increase of fiber-density on some mechanical properties of high volume FRC.
2
Materials and methods
The materials used for the fabrication of the specimens for this study are listed in Table 1 . Five FRC groups with different fractions of E-glass fiber vol.% were prepared; two additional groups were prepared by the addition of silanated barium-silicate glass particulate fillers (size 0.7 μm) with two different percentages 5% and 10%, respectively. Cylindrical specimens ( n = 12) were fabricated for each group, by pulling the fiber bundles through a special cylindrical mold with an opening diameter of 4.2 mm ( Fig. 1 ), each bundle is consisted of 4000 continuous unidirectional silanated E-glass fibers (diameter ca. 15 μm). The groups used in the investigation are listed in Table 2 . The unidirectional E-glass fiber rovings were resin impregnated with light-polymerizable bisGMA-TEGDMA (50-50%) resin and incubated in an incubator at 37 °C for 48 h (D 06062, Modell 600, Memmert GmbH + Co. KG, Schwabach, Deutschland). The composition of E-glass fibers was 55% SiO 2 , 15% Al 2 O 3 , 22% CaO, 6% B 2 O 3 , and 0.5% MgO, >1.0% Fe + Na + K. The material was cured with a quartz tungsten halogen light-curing unit (Optilux VCL 501; Demetron/Kerr, Danbury, CT, USA) for 40 s. The irradiance was 460 mW/cm 2 , as measured with a curing radiometer. Subsequently, the specimens were post-cured and tempered in a Targis Power unit (Ivoclar, Schann, Liechtenstein) for 25 min at 95 °C. After polymerization, the cylindrical specimens were ground from the endings using wet silicon carbide grinding paper (FEPA #1200). The average length of the FRC specimen was 66.0 mm, and the average diameter was 4.0 mm. After polymerization, the size of each specimen was confirmed by measuring its dimensions from three different areas. The dimensions of specimens were selected for their suitability to be used as dental implants, i.e. their load-bearing capacity should exceed average maximum occlusal forces within the physiological strain limit of bone . The specimens were conditioned at room temperature for 2 days before mechanical testing.
Product | Description | Manufacture | Lot no. | Composition |
---|---|---|---|---|
E-glass fiber | Unidirectional fiber. | Ahlstrom, Karhula, Finland | 11372313 | E-glass a |
Filler | 0.7 μm filler | Esschem, Linwood, Pennsylvania | 625-29 | Barium–aluminum–boro-silicate glass 5% silane |
Stick Resin | Light curing resin | Stick Tech, Turku, Finland | 54031672 | BisGMA- b TEGDMA c |
Glass fiber groups | Fraction of glass fiber (vol.%) | |
---|---|---|
Gravimetric analysis | Combustion analysis | |
Group A (7 bundles) | 51.7% | 49.0% |
Group B (7.5 bundles) | 53.4% | 52.8% |
Group C (8 bundles) | 56.2% | 55.8% |
Group D (8.5 bundles) | 58.2% | 57.0% |
Group E (9 bundles) | 61.7% | 60.3% |
Group F (8 bundles + 5% fillers) | 53.6% | 52.6% |
Group G (8 bundles + 10% fillers) | 57.4% | 56.5% |
The three-point bending test was used to measure the mechanical properties of the specimens adapted to ISO 10477:92 standards (crosshead speed 5.0 mm/min). All specimens were tested with a material testing machine (model LRX, Lloyd Instruments Ltd., Fareham, England) and the stress–strain curves were recorded with a PC-computer program (Nexygen, Lloyd Instruments Ltd., Fareham, England). Following flexural strength testing, the specimens were visually examined with a light microscope in an attempt to differentiate the origin of the fracture and the failure mode.
The cross-section surfaces of test specimens were ground using wet silicon carbide grinding paper (FEPA #4000) and polishing cloths with 0.1 μm AP-D suspension using a grinding machine (LaboPol-21, Struers A/S, R-dovre, Denmark). SEM-specimens were sputtered with carbon and examined with a scanning electron microscope (SEM), (Cambridge Instrument Stereoscan, LEO Electron Microscopy Ltd. 360, Cambridge, England).
The fiber content of the test specimens containing glass fibers was measured by combustion analysis with a burnout furnace (Radiance Multi-stage msc, Jelrus International, Hicksville, NY, USA). The test specimens were dried in a desiccator for 2 days at room temperature and weighed to an accuracy of 0.001 mg prior to combustion. In order to combust the polymer matrix, the temperature was raised 12 °C per min until the temperature reached 700 °C. Test specimens were maintained at 700 ± 25 °C for 1 h and reweighed. The fiber content as a percentage by volume ( V f ) (vol.%) was assessed in accordance with the following equation :
V f ( % ) = W f / r f W f / r f + W r / r r × 100 ,
where W f is the weight proportion of E-glass, r f (=2.54 g/cm 3 ) is the density of E-glass, W r is the weight proportion of resin, and r r is the density of resin (was calculated from the unreinforced test specimens).
In order to confirm the measurements given by the combustion process, the fiber content was also determined by using the gravimetric analysis based on the Archimedes method in accordance with the following equation:
V f ( % ) = r s − r r r f − r r × 100 ,
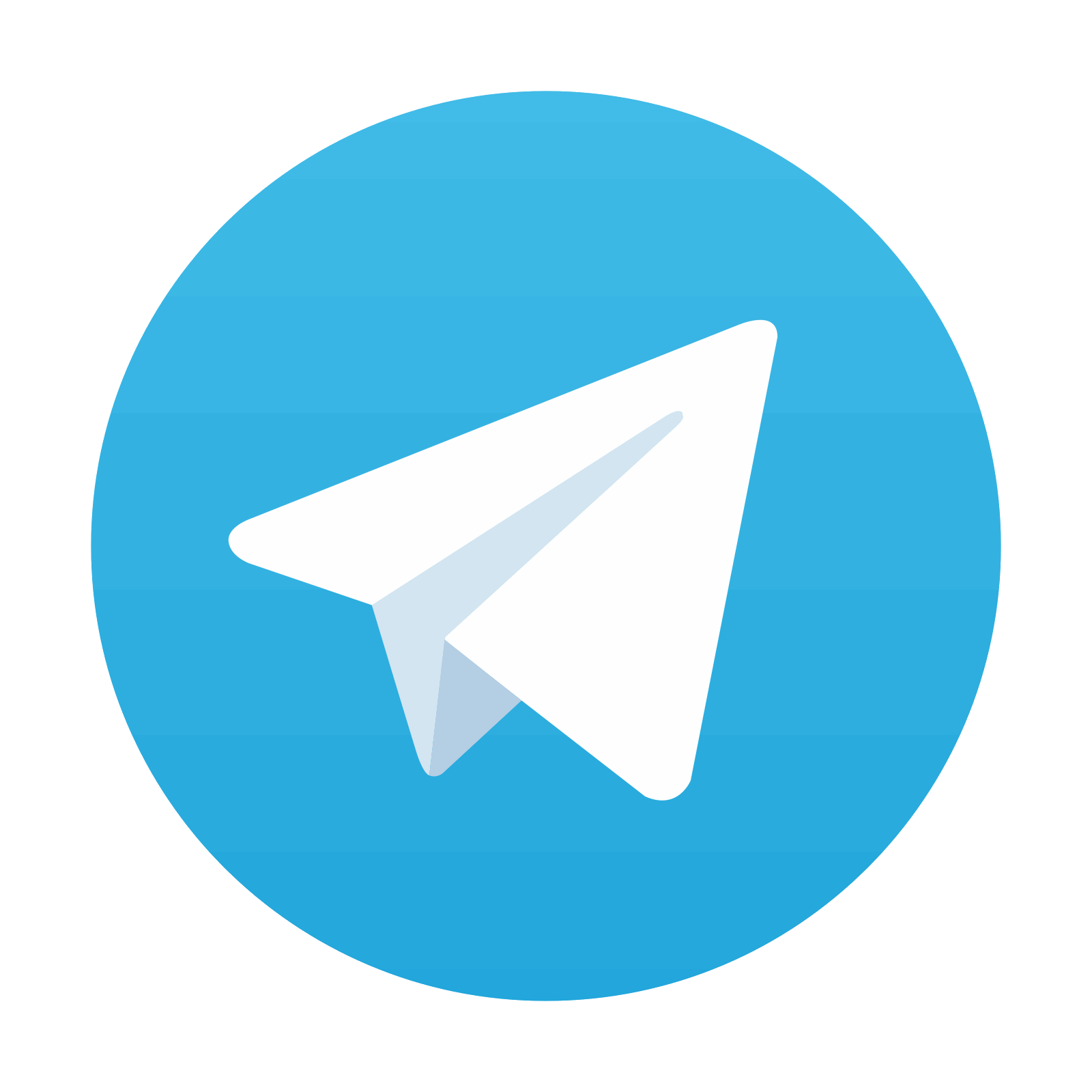
Stay updated, free dental videos. Join our Telegram channel

VIDEdental - Online dental courses
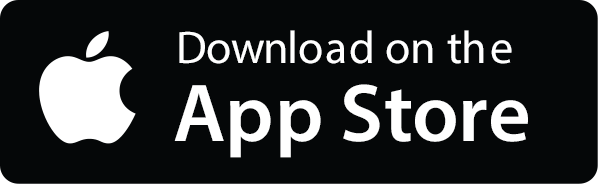
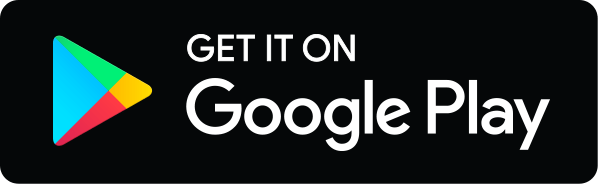