Introduction to Orofacial Pain
Key Points |
![]() |
![]() |
![]() |
![]() |
![]() |
![]() |
Orofacial pain refers to pain associated with the hard and soft tissues of the head, face, and neck. These tissues, whether skin, blood vessels, teeth, glands, or muscles, can send impulses through the trigeminal nerve to be interpreted as pain by brain circuits that are primarily responsible for the processing that controls complex behavior.1 Headaches; neurogenic, musculoskeletal, and psychophysiologic pathology; and cancer, infection, autoimmune phenomena, and tissue trauma represent the diagnostic range for the complaint of orofacial pain. Because of the diverse potential for pain arising from trigeminal receptive fields, evaluation and management of orofacial pain require collaboration among all fields of medicine.
The quest to better manage pain problems involving the trigeminal system, such as TMDs and headaches, has led to the establishment of orofacial pain as a discipline in the field of dentistry. There are residency training programs in orofacial pain, board certification processes, and increasing cooperation among advocacy groups, universities, professional organizations, and federal agencies. A huge step in the recognition of orofacial pain as a discipline in dentistry occurred in 2009 when the CoDA approved orofacial pain as an area of advanced education.2 Since 2011, multiple programs in the United States have received accreditation by CoDA. Furthermore, the International Association for the Study of Pain acknowledged the area of orofacial pain as a component of professional education by developing a core curriculum on this subject for all health care professionals.3
This revised edition is a collaborative effort derived from reviews of refereed literature spanning the spectrum of conditions at the root of orofacial pain. It is intended for health care professionals who evaluate and treat patients with orofacial pain and face the daunting task of “keeping up with the literature” in the rapidly emerging arena of pain management in clinical practice.
The Health Care Professional’s Responsibility in Orofacial Pain
The capacity to remain unbiased during evaluation and differential diagnosis is every clinician’s responsibility. Because of the diverse, complex physiologic interrelationships involved in orofacial pain complaints, all clinicians must be able to judge when their diagnostic acumen requires consultation; otherwise, treatment may not target the appropriate source.
The clinician’s responsibility is threefold. First, the clinician must combine a current working knowledge of the basic and clinical science of orofacial pain with an ability to solicit a relevant history. Appropriate questions must be asked, answers must be analyzed, and findings must be synthesized into an initial differential diagnosis.
Second, the clinician must perform a thorough clinical assessment, including a physical examination and indicated laboratory testing, imaging studies, neurologic testing, and consultations. Accurate diagnosis may require insight from other health care professionals.
Third, the clinician must be able to explain all findings to the patient as well as the details of the treatment plan, which must be consistent with standards of care based on current scientific literature. When the scope of care falls beyond individual expertise, an interdisciplinary team approach may be developed. The clinician should discuss appropriate referral options with the patient.
Epidemiology of Orofacial Pain
The 1986 Nuprin Pain Study reported that most Americans experience an average of three or four different kinds of pain every year.4 Crook et al5 reported that 16% of a general population had experienced pain within the past 2 weeks. More than 81% of the general population report at least one significant pain experience in their lifetime.6 Brattberg et al7 reported that 66% of 827 randomly selected individuals from a general population group reported pain or discomfort in different parts of their bodies, with approximately 10% describing pain in the head, face, or neck. Von Korff et al8 surveyed 1,016 members of a large health maintenance organization (HMO) and found that, while 12% reported facial pain in the previous 6 months, rates for other types of pain were 41% for back pain, 26% for headaches, 17% for abdominal pain, and 12% for chest pain. In addition, 40% of individuals reported missing one or more workdays because of pain.
Lipton et al9 surveyed 45,711 American households and reported that nearly 22% of the general population experienced at least one of five types of orofacial pain in the past 6 months. The most common type of orofacial pain was toothache, reported by 12.2% of the population. Temporomandibular joint (TMJ) pain was reported by 5.3%, with face or cheek pain being reported by 1.4%.
Orofacial pain seldom appears to be an isolated complaint. More than 81% of patients reporting to an orofacial pain center had pain sources beyond the trigeminal system,10 but few patients mention these other pain sources.11 Conditions such as fibromyalgia, chronic fatigue syndrome, headache, panic disorder, gastroesophageal reflux disorder, irritable bowel syndrome, multiple chemical sensitivity, and posttraumatic stress disorder seem to coexist with TMDs.12 Symptoms for such comorbid conditions differentiate orofacial pain patients from subjects seeking routine dental care.13 If the true pain sources are not revealed during the evaluation, prognosis may be adversely affected by the continued barrage of brain circuits as the result of chronic nociception.
Pain is a common experience that has profound societal effects. Bonica estimated that nearly a third of the population of industrialized nations suffers to some extent from chronic pain.14 Chronic pain costs billions of dollars annually for health care services, loss of work, decreased productivity, and disability compensation.
Of particular relevance to the orofacial pain community, results from a study funded by the National Institute of Dental and Craniofacial Research to identify risk factors involved in the initiation and maintenance of TMDs, as well as for developing treatments for managing TMD-associated pain, were recently published. The major objectives of this longitudinal, multidisciplinary study, titled “Orofacial Pain: Prospective Evaluation and Risk Assessment (OPPERA),” were to determine psychologic and physiologic risk factors, clinical characteristics, and associated genetic/cellular mechanisms that influence the development of TMDs. Those individuals seeking more information are encouraged to visit the Journal of Pain website.15
Pain is defined as “an unpleasant sensory and emotional experience associated with actual or potential tissue damage, or described in terms of such damage.”16Nociceptors are polymodal, high-threshold nerve endings that send tissue damage impulses on faster-conducting Aδ fibers and slower-conducting C fibers to the central nervous system (CNS). Although pain is an interpretation of nociception, many orofacial pain patients lack apparent tissue damage, and anatomical changes (such as TMJ disc displacement without reduction) do not predict continuing pain.17,18
About 25% of free nerve endings in skeletal muscle that transmit impulses to the CNS on Aδ and C fibers are chemo- and mechanoreceptive but not nociceptive.19 Some of these low-threshold receptors, called metaboreceptors, appear to be uniquely stimulated by metabolic products generated during muscle activity,20,21 while others sense the relative distension of postcapillary bed venules.22 These receptors display background activity at rest, accelerate impulse transmission as behavior intensity increases,22–24 and may affect the same central modulatory systems affected by nociception.25 The CNS uses such input to coordinate respiratory and cardiovascular changes during dynamic muscle behavior.20,22–24 Future consideration of the role of these unique receptors in pain etiology may help us better understand pain conditions in which there is no apparent visible tissue damage.
Anatomical and Physiologic Considerations of Orofacial Pain
Orofacial pain may be defined as pain and dysfunction affecting motor and sensory transmission in the trigeminal nerve system.26 From a sensory perspective, the trigeminal system oversees the efficacy and tissue integrity of highly integrative orofacial behaviors that are controlled by cranial nerves and modulated by the autonomic nervous system (ANS) and the greater limbic system.27 Orofacial nerves transmit information about pressure (touch), position (proprioception), temperature, and potential pain to the trigeminal nuclei, which have extensive bidirectional connections throughout the brain.28–30 These trigeminal connections affect the sensory, motor, and autonomic-endocrine changes that occur during orofacial behaviors, and, when these behaviors are impaired, orofacial pain may result. The next sections briefly discuss peripheral and central trigeminal neuroanatomy to elucidate how the trigeminal system affects physiology and pain.
Neuroanatomy of the orofacial structures
Cranial nerves are extensions of the brain that innervate tissues involved with the trigeminal system directly or indirectly.14 The specialized neurons of the olfactory, optic, and vestibulo-cochlear nerves that send smell, sight, sound, and balance information to the CNS do not travel through the trigeminal nuclei. However, nerves associated with the nose, eye, and ear tissues do transmit proprioceptive, pressure, and potential pain impulses into the trigeminal nuclei. These cranial nerves, along with the occulomotor, trochlear, abducens, and the hypoglossal nerves, are not reviewed here. The remaining five cranial nerves and upper cervical nerves are briefly discussed. However, a comprehensive orofacial pain evaluation should include a basic assessment of the function of all cranial nerves (see chapter 2).
Trigeminal nerve
The trigeminal nerve, which provides sensory innervation to most of the head and face, is the primary nerve involved in TMDs, migraine, sinus, pulpal, and periodontal pathology. It is the largest cranial nerve, consisting of three peripheral divisions: the ophthalmic, the maxillary, and the mandibular.31–34 Figure 1-1a shows the regions where these branches receive sensory input that is conveyed by first-order neurons through the trigeminal ganglion, where most neuronal cell bodies are located. Although these neurons enter the ganglion on three branches, they exit in one large sensory root that enters the brainstem at the level of the pons before reaching the trigeminal nuclei.35
Fig 1-1 Basic neuroanatomy. (a) Receptive fields for the ophthalmic (V1), maxillary (V2), and mandibular (V3) branches of the trigeminal nerve and the upper cervical nerves. (b) The “onion peel” facial dermatomes. Impulses are carried on V1, V2, and V3 through the trigeminal ganglion to the bilateral trigeminal nuclear columns in the pons. (c) The trigeminal nuclear columns and converging nerves. Note the subnucleus caudalis and the rostrocaudal arrangement of its lamina (1 to 5), which receive nociceptive impulses from the respectively numbered facial dermatomes, whether they originate on V1, V2, or V3. Also note where cranial nerves VII, IX, X, and C2, C3, and C4 can provide pathways for convergence onto trigeminal nuclei. SN—subnucleus.
Ophthalmic branch (V1). This branch of the trigeminal nerve leaves the skull through the superior orbital fissure and transmits sensory information from the scalp and forehead, upper eyelid, conjunctiva and cornea of the eye, nose (including the tip of the nose), nasal mucosa, frontal sinuses, parts of the meninges (the dura and blood vessels), and deep structures in these regions. It also carries postganglionic parasympathetic motor fibers to the glands and sympathetic fibers to the pupillary dilator muscles.35
Maxillary branch (V2). This branch exits the skull at the foramen rotundum. It has a sensory function for the lower eyelid and cheek; the nares and upper lip; the maxillary teeth and gingiva; the nasal mucosa; the palate and roof of the pharynx; the maxillary, ethmoid, and sphenoid sinuses; and parts of the meninges. Near its origin, it divides to form the middle meningeal nerve, which supplies the middle meningeal artery and part of dura mater. The terminal V2 branches—the anterior and greater palatine nerves and the superior, middle, and anterior alveolar nerves—innervate the soft palate, uvula, hard palate, maxillary gingiva and teeth, and mucous membranes of the cheek.35
Mandibular branch (V3). This branch leaves the skull through the foramen ovale and functions in sensory and motor transmission. V3 carries sensory information from the lower lip, mandibular teeth and gingiva, floor of the mouth, anterior two-thirds of the tongue, chin and jaw (except the angle of the jaw, which is supplied by C2 and C3), parts of the external ear, parts of the meninges, and deep structures. A branch of V3, the auriculotemporal nerve, innervates most of the TMJ.
The motor nuclei use V3 to provide motor fibers to the muscles of mastication (ie, masseter, temporalis, medial pterygoid, lateral pterygoid, anterior digastric, and mylohyoid), the tensor veli palatini involved with eustachian tube function, and the tensor tympani, which attaches to the malleus bone in the eardrum.35
Trigeminal sensory nuclei. The trigeminal sensory nuclei lay in bilateral columns on either side of the brainstem that originate in the midbrain and terminate in the dorsal horn of the cervical spinal cord (Fig 1-1c). They are, in a rostrocaudal orientation, the mesencephalic nucleus, the main sensory nucleus, and the spinal trigeminal nucleus. All touch, position, and temperature sensory input from the face plus potential pain input from the face, head, and neck is sent to the trigeminal nuclei.14
The mesencephalic nucleus, which is more a ganglion than a nucleus, houses the cell bodies of the proprioceptive neurons that convey input from the apical periodontal ligament and the muscle fibers that contract during the jaw-closing reflex. These proprioceptive neurons, and possibly the blink reflex nerves, represent the only peripheral nerves with cell bodies located within the CNS.14,36 The neurons are monosynaptic and pass through the mesencephalic nucleus to synapse in the trigeminal motor nuclei located medially to the much larger main sensory nucleus. The main sensory nucleus receives the facial proprioceptive and pressure input for orofacial behaviors (eg, chewing, kissing, smiling, and light touch) other than the jaw-closing reflex. These neurons have their cell bodies in the trigeminal ganglion and synapse in the main sensory nucleus, where input is conveyed to the motor nuclei by arrays of small interneurons.14
The spinal trigeminal nucleus, sometimes referred to as the medullary dorsal horn because it extends into the spinal cord, consists of three subnuclei: subnucleus oralis, subnucleus interpolaris, and subnucleus caudalis. Subnucleus oralis and subnucleus interpolaris receive some peripheral nociceptive fibers, but mostly they receive temperature information on Aδ fibers and touch impulses on Aβ fibers from the periphery and convey this input via interneurons to the motor nuclei.14 In response to nociceptor activation, neuropeptides and other inflammatory agents are released in the spinal trigeminal nucleus and can cause excitation of neurons and glial cells that promotes development of central sensitization, allodynia, and hyperalgesia, which are physiologic events associated with acute and chronic pain.37,38
The subnucleus caudalis is the main terminus for most slow first-order neurons that convey potential pain from trigeminal receptive fields. Figures 1-1b and 1-1c illustrate the “onion peel” somatotropic organization of the face (areas 1 to 5) and the corresponding laminae (1 to 5) in the subnucleus caudalis, where first-order nociceptive neurons terminate regardless of their division of origin.14 For instance, Aδ and C-fiber neurons from area 5 in the face, whether they start in V1, V2, or V3, all synapse with second-order nociceptive neurons in the most caudal aspect of the subnucleus caudalis, lamina 5. Such convergence means that a dural blood vessel, masseter muscle, or a tooth or tongue nociceptive afferent could excite the same second-order neurons. This convergence, the anatomic basis for referred pain, is not just a facial phenomenon. Cervical spine nociceptive afferents also synapse in the subnucleus caudalis, meaning that trapezius or sternocleidomastoid nociceptive afferents can excite second-order neurons that also receive input from facial tissues29,30,39 (Fig 1-2). In support of this notion, recent findings from the OPPERA Study have provided evidence that muscle pain in the neck and shoulder is highly correlated with both acute and chronic TMDs. Thus, this type of neuronal organization may help to explain the high prevalence of comorbid pain conditions associated with tissues in the head and face (ie, headache and sinusitis, headache and TMDs).
Fig 1-2 Sensory pathways and motor response to referred pain. The first-order neurons from a pain site in facial lamina 5 and from the pain source in the C4 receptive field each converge on lamina 5 of the subnucleus caudalis and excite the same second-order neurons. As these second-order neurons ascend, they arborize with the subnucleus oralis and subnucleus interpolaris (not shown) and many reticular formation structures before synapsing with third-order neurons in the thalamus. The third-order neurons are thalamocorticobasal ganglia-limbic circuits that interpret pain and generate the descending motor and pain modulatory reactions to pain interpretation. The descending motor neurons also arborize with reticular formation locations and connect, via interneurons, to the trigeminal motor nucleus and to all cranial nerve motor nuclei. Note that trigeminal input is never analyzed in isolation, as primary sensory and spinal thalamic tract input is also always being presented to the brain for analysis. RF—reticular formation structure; SN—subnucleus; STT—spinal thalamic tract.
Another construct to consider is that all of the CNS structures affected by trigeminal nociceptive input are also contacted by second-order neurons from the dorsal horn of the spinal cord.16 Therefore, potential pain input from regions outside trigeminal receptive fields may excite CNS structures that communicate with trigeminal nuclei and modulate their functions.
Facial nerve
The seventh cranial nerve is a mixed nerve that has five branches (temporal, zygomatic, buccal, mandibular, and cervical) that course through the parotid gland but do not innervate the gland. Its main function is motor control of most of the muscles of facial expression and the stapedius muscle of the middle ear. The facial nerve supplies parasympathetic fibers to the sublingual and submandibular glands via the chorda tympani and to the lacrimal gland via the pterygopalatine ganglion. In addition, it conveys taste sensations from the anterior two-thirds of the tongue to the solitary tract nucleus and transmits cutaneous sensation from the skin in and around the earlobe via the nervus intermedius.35
Glossopharyngeal nerve
The ninth cranial nerve is a mixed nerve comprising somatic, visceral, and motor fibers. It conveys sensory information from the posterior third of the tongue, tonsils, pharynx, middle ear, and carotid body. Taste sensation from the posterior third of the tongue as well as carotid body baroreceptor and chemoreceptor information is transmitted to the solitary tract nucleus. Nociceptive input from the ear is sent to the spinal trigeminal nucleus. From the inferior salivatory nucleus, the glossopharyngeal nerve delivers parasympathetic control to the parotid and mucous glands throughout the oral cavity. Motor fibers from the nucleus ambiguus project to the stylopharyngeus muscle and upper pharyngeal muscles. An altered gag reflex indicates glossopharyngeal nerve damage.35
Vagus nerve
The tenth cranial nerve originates in the brainstem and extends to the abdomen and innervates virtually all organs from the neck to the transverse colon except the adrenal glands. It supplies visceral afferent fibers to the mucous membranes of the pharynx, larynx, bronchi, lungs, heart, esophagus, stomach, intestines, and kidneys and distributes efferent or parasympathetic fibers to the heart, esophagus, stomach, trachea, bronchi, biliary tract, and most of the intestine. Also, the vagus nerve af-ects motor control of the voluntary muscles of the larynx, pharynx, and palate and carries somatic sensory fibers that terminate in the skin of the posterior surface of the external ear and the external acoustic meatus.35 Through these connections, the vagus affects activities as varied as respiration, cardiac function, sweating, digestion, peristalsis, hearing, and speech.
Spinal accessory nerve
The eleventh cranial nerve innervates the cervical muscles, the sternocleidomastoid and trapezius, which are coactivated during masticatory behaviors. Like the trigeminal motor nucleus, the accessory motor nuclei are rich in norepinephrine receptors, which can facilitate vigilant behaviors.40 Nociceptive afferents from the cervical muscles converge onto the spinal trigeminal nucleus. It is notable that cervical myofascial pain seems to be prominent in patients with orofacial pain.
Upper cervical nerves
Spinal nerves C1 to C4 and possibly C5 are important considerations in orofacial pain because their sensory fibers converge onto the trigeminal subnucleus caudalis.29,30,39 As C1 to C4 leave the spine, they combine to form the cervical plexus, which yields cutaneous, muscular, and mixed branches. C1 forms the suboccipital nerve that supplies motor control to the muscles of the suboccipital triangle. The cutaneous branches are the lesser occipital (C2, C3), the greater auricular (C2, C3), the transverse cervical (C2, C3), and the supraclavicular (C3, C4). These nerves innervate the back of the head and neck, the auricle and external acoustic me-atus, the anterior neck and angle of the mandible and the shoulders, and the upper thoracic region. The muscular branch, the ansa cervicalis, innervates the sternohyoid, sternothyroid, and omohyoid muscles and is composed of a superior root (C1, C2) and an inferior root (C2, C3). The mixed branch is the phrenic nerve (C3, C4, and C5), which innervates the diaphragm.35
Autonomic nervous system
The ANS, which is commonly viewed as a largely involuntary motor system, is composed of three peripheral divisions, the sympathetic, parasympathetic, and enteric, that function to maintain homeostasis.35 The peripheral ANS is controlled by the central ANS, which is composed of cortical, limbic, and reticular formation structures and nuclei.40 Stimuli that activate the central ANS induce increased sympathetic activity initially in the brainstem and then in the periphery.40,41 The sympathetic system is involved in vigilance, energy expenditure, and the “fight or flight” response, while the role of the parasympathetic system is to counterbalance sympathetic arousal with “rest and digest” actions.42 The sympathetic and parasympathetic systems have preganglionic neurons that originate in different parts of the CNS and postganglionic neurons that deliver impulses to target tissues. Preganglionic neurons release acetylcholine at the autonomic ganglia, and postganglionic sympathetic and parasympathetic neurons release norepinephrine and acetylcholine, respectively, at the target sites.
The enteric system provides local sensory and motor fibers to the gastrointestinal tract, the pancreas, and the gallbladder. This system can function autonomously but is regulated by CNS reflexes. Its control of gastrointestinal vascular tone, motility, secretions, and fluid transport plays a vital role in homeostasis. Persistent sympathetic arousal that impairs parasympathetic function and leads to disturbances of the enteric system may be relevant to orofacial pain, because functional disorders of visceral organs controlled by the ANS seem to be common comorbid conditions.12,13,42
Sympathetic input to the orofacial region. Sympathetic preganglionic neurons originate in the spinal cord. Their cell bodies are found in the intermediolateral gray matter at the level of the 12 thoracic and upper 3 lumbar vertebrae. They exit the spinal cord via the ventral horn at the segmental level, where their cell bodies are located, but can synapse with any of the sympathetic ganglia in the bilateral paravertebral chains. The superior portion of the sympathetic chain contains four cervical ganglia. In a rostrocaudal orientation, they are the superior cervical, middle cervical, intermediate cervical, and stellate ganglia. Postganglionic fibers leaving these sympathetic ganglia transmit motor input to the blood vessels in the head and neck, various glands, and the eyes. The skin of the face and scalp receive sympathetic innervation from the superior cervical ganglia via plexuses extending along the branches of the external carotid artery.35,42
Parasympathetic input to the orofacial region. Parasympathetic preganglionic neurons originate in the brainstem nuclei, where their cell bodies are located, or in the lateral gray columns of the sacral spinal cord (S2 to S4). Cranial nerves III, VII, IX, and X and the splanchnic nerve in the pelvic region carry parasympathetic preganglionic neurons, which are considerably longer than the postganglionic fibers because ganglia are generally located close to or embedded in the target organ.
Neurophysiology of Orofacial Pain
Orofacial pain pathways
Nociceptive impulses generated by potential or actual tissue damage are just one type of input that is continuously assessed at different levels of the CNS. The senses (smell, sight, hearing, touch, and taste) alert the brain to stimuli through thalamic-amygdala and thalamic-cortical-amygdala circuits, and those data streams are analyzed and compared with what the brain already knows in order to sequence efficient behavior.43,44 Ongoing proprioceptive, nociceptive, thermoreceptive, baroreceptive, chemoreceptive, and vestibular input informs the brain how effectively its tissues are conducting responses and enables the brain to make ongoing behavioral adjustments aimed at maintaining efficiency. Nociception provides the brain an opportunity to interpret pain and make behavioral adjustments to avoid further, potentially damaging stimuli.
First-order nociceptive nerves, whether they synapse in the spinal trigeminal nucleus or in the dorsal horn, excite the same type of second-order neurons that are responsive to nociceptive signals or respond to a variety of sensory stimuli and hence are referred to as wide-dynamic range neurons. These neurons conduct nociception and/or other sensations through the brainstem and display varying degrees of arborization with structures throughout the reticular formation, where baseline physiologic processes are controlled, before reaching the third-order neurons in the thalamus14,45–47 (see Fig 1-2). Second-order neurons, stimulated by the faster-conducting Aδ fibers that release glutamate, arborize less than those receiving impulses from the slower-conducting C fibers that release a wide variety of neurotransmitters.14,48,49 Thus, information from Aδ fibers allows for a much faster nocifensive response (ie, reflex response) than that elicited by C-fiber input, which is important in maintaining persistent pain and coordinating reparative and behavioral responses.
With sufficient temporal and/or spatial summation, third-order circuits, which start in the thalamus and connect the sensory cortex with the basal ganglia and the limbic system, interpret nociceptive input, and pain is perceived.1,14 While pain can often be felt, it is sometimes difficult to locate the actual source. Sites of cutaneous stimuli are easier to recognize than stimuli from the muscles and visceral organs because the dermis has more nociceptive free nerve endings than are found in deep tissues to assess integument integrity.14
In response to pain interpretation, multilevel behavioral responses are coordinated, and descending motor commands are created. Whether nociception is delivered to the CNS through the spinothalamic tract or the trigeminal thalamic tract, pain perception evokes ANS-modulated cranial nerve responses.14,50,51 Because tissues under cranial nerve control will continue to excite trigeminal nociceptive pathways, an orofacial pain prognosis may be poor if ongoing pain sources beyond trigeminal receptive fields cannot be controlled.
Nociception and pain modulation
Organisms need to be able to recognize and avoid pathologic pain to prevent potential tissue damage. However, normal daily activities should not be significantly altered by transient physiologic pain. Therefore, nociception has a biphasic effect in the CNS. Initially, low-intensity nociceptive impulses are facilitated through the CNS and then by stimulation of the cortex and a variety of brainstem regions, while inhibition may be facilitated via activation of the rostral ventral medulla (RVM) and the periaqueductal gray regions (PAG).52,53 If nociception is relatively minor, inhibitory mechanisms will minimize the impact of transient nociceptive barrages in the CNS that affect cognitive function and task performance. Simultaneously, low-intensity nociception via second-order neuron arborization stimulates reticular formation structures to coordinate adjustments in motor and vascular behavior.51 Such adjustments, because of net inhibition, can occur almost below the level of consciousness, and we continue to behave efficiently. In addition, data from human and animal studies support a role for diffuse noxious inhibitory controls (DNIC) in modulating response to painful stimuli.54,55 This occurs at the level of the spinal cord and is mediated when some neurons are strongly inhibited in response to a nociceptive stimulus applied to any part of the body, distinct from their excitatory receptive fields. For example, stimulation in other, more remote areas of the body is reported to induce inhibitory reflex movements in the jaw and tongue in response to noxious craniofacial stimulation.56,57 Thus, the inhibitory effects of DNIC are observed in nociceptive neurons and wide-dynamic range neurons in the spinal trigeminal nucleus as well as in sensorimotor behavioral responses involving the spinal trigeminal nucleus.58–61 Because the term DNIC, although still widely used, describes a specific inhibitory mechanism at the lower brainstem level, a group of clinicians and basic scientists has proposed a new term that could be used for psychophysical testing in humans. This new term, conditioned pain modulation, can be used to describe a neuronal mechanism by which pain inhibits pain at all levels in the CNS.62,63 Importantly, dysfunction of these neuronal inhibitory control mechanisms is implicated in promoting and maintaining chronic orofacial pain. Of clinical relevance, dysfunction in DNIC may make those individuals more likely to progress to a chronic pain state following tissue injury or infection in the orofacial region.
When nociception persists to excite third-order neurons and pain is realized, the brain’s inhibitory capacity, called stimulation-produced analgesia (SPA), must work harder to counteract facilitation. SPA, by both noradrenergic and serotonergic pathways, inhibits nociceptive transmission at many sites but initially where first- and second-order neurons synapse in the spinal trigeminal nucleus or in the dorsal horn.14 This descending inhibition is mediated by endogenous opioids, gamma-aminobutyric acid (GABA), and various inhibitory amino acids that are located in the PAG. These same inhibitory compounds are released when stressors induce anxiety, fear, or depression.64 Brain circuits that interpret pain and direct descending inhibition also send signals to direct alterations in motor behavior and ANS functions. These descending commands reach structures throughout the reticular formation and, by vast pools of interneurons, affect all cranial nerve motor nuclei and alter behavior in response to pain65–67 (see Fig 1-2). Alternative motor pathways are recruited, and protective changes in respiration and cardiovascular mechanisms are engaged.68 In the case of more trigeminal motor activity, premotor interneurons deliver messages to the main sensory nucleus, the subnucleus oralis, and the subnucleus interpolaris, which, through interneurons, alter motor neuron sequencing in the motor nuclei. These same nuclei mediate the minor motor adjustments45–47 when net inhibition minimizes minor nociceptive volleys from intruding on circuits where pain is perceived.
Sensitization. With persistent nociception, facilitation can exceed inhibitory capacity, and a spectrum of neuroplastic changes occurs, first peripherally and then centrally. These changes are referred to as peripheral and central sensitization. The following changes are characteristic of neuronal sensitization: Nerve thresholds are lowered, receptive fields are enlarged, synaptic contacts are rearranged and become more efficient, gene expression is altered, and pain is persistent and evoked by nonpainful stimuli.48–50
Importantly, in the transition from acute to chronic pain, nociceptive neurons can change the type and level of expression of receptors and ion channels leading to the development of a primed state.69 In the primed state, lower levels of inflammatory mediators are required to generate nociception, and sensitizing agents can become stimulatory agents. The transformation of nociceptors to the primed state is implicated in persistent pain conditions. In normal situations, high-threshold peripheral nociceptors do not fire unless exposed to noxious stimuli. However, repeated stimulation can quite rapidly reduce firing thresholds by the actions of a variety of inflammatory molecules acting on various receptors. The antidromic release of neurogenic inflammatory compounds by perivascular afferents at the location of the pain also enhances peripheral nociceptor sensitization. This increase in the transmission frequency of noxious action potentials to second-order neurons is called long-term potentiation and, if persistent, leads to central sensitization.48,49
The development of sensitization is a time-and intensity-dependent progression. Initially, low-intensity nociceptive volleys carried on Aδ fibers release glutamate and activate postsyn-aptic alpha-amino-3-hydroxy-5-methyl-4-isox-azolepropionic acid (AMPA) receptors in the spinal trigeminal nucleus or dorsal horn. Higher-intensity stimuli induce C fibers to release neuropeptides and other inflammatory mediators that cause changes in the expression and activity of neuronal receptors and ion channels that result in lower activation thresholds in second-order neurons.70,71
In nonpainful states, Aβ fibers release only glutamate and deliver tactile sensations, which are important for coordination of motor behaviors, to the subnucleus oralis and subnucleus interpolaris or dorsal horn lamina 3 and 4. As central sensitization develops, thresholds where second-order neurons arborize to the subnucleus oralis and subnucleus interpolaris are lowered,37,38 and Aβ fibers can begin to sprout axons into the adjacent nociceptive lamina.55,72 Thus, nonpainful stimuli that converge onto a sensitized CNS will be interpreted as painful because of this structural reorganization in the adult central nervous system73 (Fig 1-3). Reduction of inhibition or reorganization of synaptic connectivity are other mechanisms by which Aβ fibers may be recruited to mediate pain. Patients thus suffer allodynia (pain induced by stimuli that normally would not be perceived as painful), pain exacerbations, and hyperalgesia (an exaggerated pain response to painful stimuli).14
Fig 1-3 Sensitization. First-order nociceptive neurons from facial lamina 5 transmitted via V1 and C4 converge onto lamina 5 of the subnucleus caudalis. The pain sources are not controlled, summation exceeds descending inhibition, and progressive levels of central sensitization occur, first at the subnucleus caudalis, then at the ipsilateral subnucleus oralis, where Aβ fibers are carried on V3 synapse. With continued summation, sensitization occurs at higher brain sites and at the contralateral subnucleus oralis. Nonpainful thermal and tactile inputs are experienced as painful (allodynia) or a more intense pain is felt (hyperalgesia) because of the effects of central sensitization. RF—reticular formation structure; SN—subnucleus.
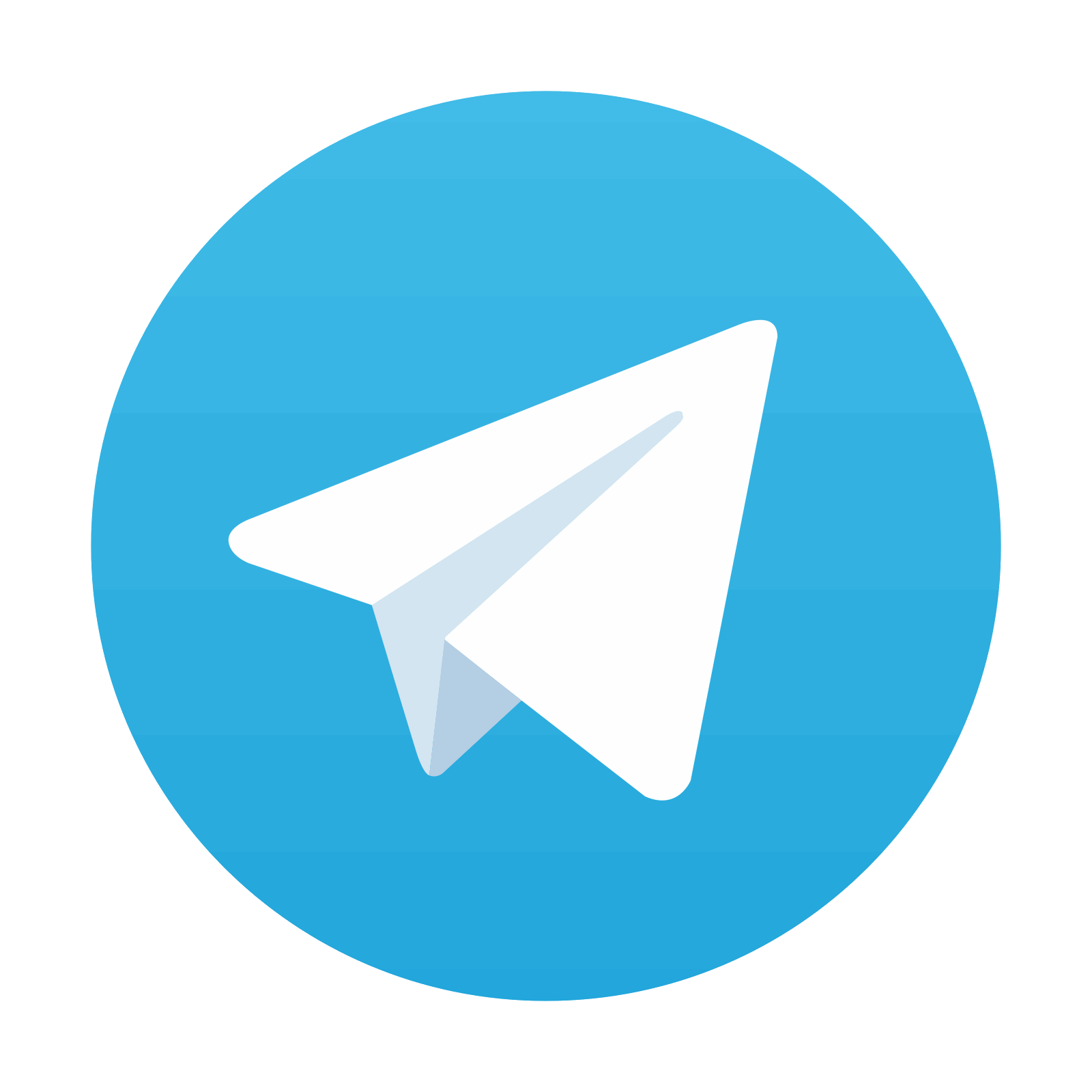
Stay updated, free dental videos. Join our Telegram channel

VIDEdental - Online dental courses
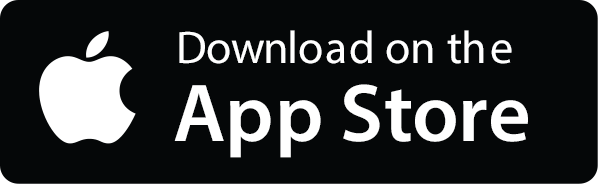
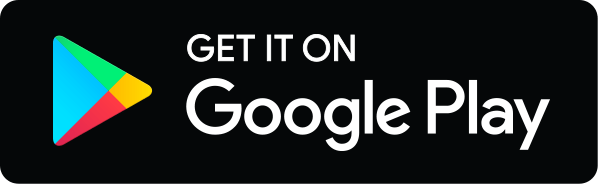