Highlights
- •
Internal adaptation in a high C-factor cavity was inferior to that in a low C-factor cavity for both conventional and bulk-filled composites.
- •
Internal adaptation, polymerization shrinkage, and stress were different among composite resin materials.
- •
Polymerization stress under the compliance-allowed condition showed significant correlations with internal adaptations in high and low C-factor cavities.
- •
Polymerization stress under the zero-compliance condition did not show a significant correlation with internal adaptation in the low C-factor cavity.
Abstract
Objective
This study compared internal adaptation of composites under different C-factors and examined the relationship between internal adaptation and polymerization shrinkage parameters.
Methods
Cylindrical cavities 3 mm in diameter were prepared in 100 human third molars in two depths: 4 mm high C-factor (H-CF) or 1 mm low C-factor (L-CF). After adhesive application (Clearfil SE One, Kuraray Noritake), the composite was placed in two increments in three subgroups: Filtek Supreme (FS, 3 M ESPE); Charisma Diamond (CD, Heraeus Kulzer); Amelogen Plus (AP, Ultradent); and as a single increment in two subgroups: Tetric EvoCeram Bulk Fill (TB, Ivoclar Vivadent) and Venus Bulk Fill (VB, Heraeus Kulzer). After thermo-mechanical load-cycles, imperfect margin percentage (%IM) was calculated using swept-source optical coherence tomography (SS-OCT) imaging. The relationships between %IM and linear shrinkage (LS) and shrinkage stress, measured under either zero-compliance (PS0) or compliance-allowed (PS) conditions were evaluated.
Results
The %IM was significantly different between H-CF and L-CF groups. The %IM in H-CF turned out to be as groups CD, FS ≤ TB < AP, VB. The %IM in L-CF showed as groups CD, TB ≤ FS, AP < VB. There were significant correlations between shrinkage parameters and %IM, except between PS0 and %IM in L-CF.
Significance
Internal adaptation in a high C-factor cavity was inferior to that in a low C-factor cavity for both conventional and bulk-filled composites. Internal adaptation, polymerization shrinkage, and stress were different among composite materials. Polymerization stress under the compliance-allowed condition showed significant correlations with internal adaptations in high and low C-factor cavities.
1
Introduction
Developments in resin composite technology and dentin bonding systems have improved the physical properties of these materials. However, in spite of the significant increase in physical properties and bonding strength values, the occurrence of microleakage and gap formation remains a frequent issue . This can be due to resin composite shrinkage, which still ranges between 1% and 6% by volume even for the newly developed low-shrinkage products. . Polymerization stress in composite restorations develops as a result of this polymerization shrinkage under confinement created by bonding to cavity walls.
The magnitude of the polymerization shrinkage stress is influenced by numerous factors. These factors can be divided into the cavity configuration and the material properties. The cavity configuration includes elements such as cavity size, ratio of bonded to unbonded surfaces known as C-factor and compliance of the cavity wall. The C-factor defines configuration of cavity walls and is known as an important variable that should be considered when placing composites. In addition, the compliance of the substrate to be bonded affects stress development. As for the material properties, filler content of composite, matrix formulation, polymerization shrinkage, elastic modulus, flow of the resin and adherence of the resin to the wall should be considered . Filler content in composite resin can be one of the key factors; if the composite has high inorganic filler content, it will show low volumetric shrinkage and high stiffness . The amount of polymerization shrinkage and viscoelastic properties can also be variables in shrinkage stress. Braga et al. indicated that volumetric shrinkage prevails over viscoelastic properties in determining contraction stress . The viscoelastic properties include its flow capacity and elastic modulus, both of which can be variable during polymerization . Lower elastic modulus and better viscous flow at the early stage of polymerization can reduce shrinkage stress. In fact, the interplay among these factors determines the polymerization shrinkage stress.
The most frequent method of measuring contraction stress is to use the tensilometer; however, there is some controversy about this method. One of these concerns is the compliance of the testing system. There are two types of measurement systems: zero-compliance (rigid) setup and compliance-allowed (non-rigid) setup. Zero (or near-zero) compliance means that a feedback system exists during the polymerization shrinkage measurement to keep a constant distance between the measurement components, simulating a situation in which the shrinking composite is completely confined by rigid walls. When the test system does not have a feedback system, it indicates that the compliance is allowed, when composite resin can shrink relatively freely. When contraction stress is measured with a non-rigid setup, the shrinkage stress can be dissipated through the components of the system. There are conflicting reports on the relationship of the shrinkage stress to the C-factor with these two settings. When the rigid (zero or near-zero) compliance system was used, there was a direct relationship between the polymerization stress and C-factor value . If the non-rigid system was used, an inverse relationship could be found . These findings raised the questions of why there were opposite results of the relationship between the stress and C-factor and which one of the two measurement systems would be applicable to evaluating microleakage or internal adaptation.
The C-factor concept should be carefully applied to clinical practice. Cavity configurations have a much more complex geometry than the specimens used in an experimental test. When it comes to C-factor and microleakage, Uno et al. reported that there was no relationship between the C-factor and gap dimension in compomer restorations with different C-factors . Another research suggested that microleakage seemed to be related to a restoration’s volume but not to its C-factor . However, in these studies, samples of different C-factors, set by different volumes of composite, were compared. The C-factor seemed to be a valid parameter in comparisons of restorations of identical shapes and volumes.
Microleakage is an inevitable consequence of polymerization shrinkage in composite restoration. Some studies have correlated the results from contraction stress tests and those from microleakage tests . Ferracane et al. investigated the relationship between composite contraction stress and microleakage in Class V restorations . Calheiros et al. studied the polymerization contraction stress of low-shrinkage composite and its correlation with microleakage . They found a direct relationship between contraction stress values and microleakage for the composites. Marginal gap formation is the result of a localized bond failure and it is a concern where the microgap is found in the interfaces of the restorative material and tooth substrate, resulting in a leak. Nevertheless, marginal seal could be different from internal adaptation because localized debonding may produce microgaps that are not always associated with the outside margin and are not readily apparent . For the Class I cavity, it was found that microgaps were dominantly formed on the pulpal cavity floor rather than on the outer enamel margin .
Internal adaptation, which defined how well a restoration adapted internally to the dental substrate, can involve the evaluation of microgaps at the pulpal floor of a restoration. Clinically, it may affect hypersensitivity to cold or pain on mastication and, possibly, mechanical strength and durability of a restoration . For internal adaptation evaluation, the specimen should be cut and examined unless a non-destructive method is used. Physical sectioning of specimens might lead to increased artefacts and interfacial gap values and cannot be evaluated on the same specimen repeatedly . The number of sections per specimen is also a limiting factor, which may not be representative of the whole specimen. Not long ago, optical coherence tomography (OCT) was introduced as a noninvasive, cross-sectional imaging method for biological systems . After a laser source is projected over a restoration, the backscattered light is transformed into a signal intensity that can be shown as an image. OCT is a noninvasive method that can be used for different purposes such as detecting dental caries or cracks . A specific type of OCT is a swept-source optical coherence tomography (SS-OCT), which is known to have a better image resolution and scanning speed. Sadr et al. reported a methodology to quantify internal adaptation of direct dental composites by SS-OCT based on backscatter signal intensity .
A recent development in composite resins is the bulk-fill technology that supposedly allows placement of resin composite in a single increment up to 4 or 5 mm in depth without compromising polymerization efficiency . Bulk-fill composites can be divided into two categories: flowable type and nonflowable type. Manufacturers claim that these recent bulk-fill composites have higher filler contents and increased physical properties. However, it has been questioned whether bulk-fill resins offer any advantage over existing composites in terms of polymerization shrinkage and internal adaptation.
The objectives of this study were to compare the internal adaptations of different resin composites in two cavity configurations (H-CFs and L-CFs) and to find out the relationship between the internal adaptation and polymerization shrinkage or shrinkage stress. The shrinkage stress was evaluated under the zero-compliance and compliance-allowed conditions. In the present study, two cavities of different C-factors were prepared, representing high and low C-factors. The composite restoration of the same shape and volume was built up with different resins as subgroups, and then the internal adaptations were compared in two ways.
The null hypotheses were:
- 1.
There was no difference in internal adaptation between high and low C-factor cavities.
- 2.
There was no difference in internal adaptation among the different composite restorations in each high and low C-factor cavity.
- 3.
There was no relationship between internal adaptation and linear polymerization shrinkage or polymerization stress measured under either zero-compliance or compliance-allowed conditions.
2
Materials and methods
The setup of this study is schematically illustrated in Fig. 1 .
2.1
Specimen preparation
One hundred non-carious human third molars were used in this study. They were stored in 0.5% chloramine solution for up to 2 months after extraction. The occlusal surface of each tooth was flattened with a trimmer and 320-grit sandpaper. All the teeth were randomly divided in two groups: a high C-factor cylindrical cavity group and a low C-factor cylindrical cavity group. For the high C-factor cylindrical cavity, round cylinder-shaped Class I cavities (3 mm in diameter, 4 mm in depth) were made on the occlusal surface of each tooth, using a high-speed air-turbine hand-piece with a flat-end tapered diamond bur (959 KR 018, Komet, Germany) under water coolant. Then the irregularities and cavity dimension were finely tuned using a low-speed hand-piece with a cylinder-shaped stone point (040 Dura green stone, Shofu, Japan) and hand instruments. For the low C-factor cylindrical cavity, all the teeth were reduced 3 mm in height to the same depth of dentin as the high C-factor cavity before tooth preparation. The cavity bottom dentin in all the specimens was mid-coronal to make sure that the effects of regional variability would be negligible. Then round cylinder-shaped Class I cavities (3 mm in diameter, 1 mm in depth) were made on the occlusal surface of each tooth by the same methods as in the high C-factor cavity.
2.2
Resin composite filling
The high C-factor cavity group (H-CF) was divided into five subgroups ( n = 10) according to the composite used. A one-step, self-etch adhesive (Clearfil SE One, Kuraray Noritake Dental, Tokyo, Japan) was used for dentin bonding. Following the manufacturer’s instruction, the cavities were treated and light-cured. Composite filling materials included three kinds of hybrid composites and two bulk-fill types ( Table 1 ). The five subgroups of the high C-factor group were the following: Group H-CF-FS, which was filled with Filtek Supreme (3M, St. Paul, MN, USA); Group H-CF-CD with Charisma Diamond (Heraeus Kulzer, Dormagen, Germany); Group H-CF-AP with Amelogen Plus (Ultradent, South Jordan, UT, USA); Group H-CF-TB with Tetric Evoceram Bulk Fill (Ivoclar Vivadent, Schaan, Liechtenstein); and Group H-CF-VB with Venus Bulk Fill (Heraeus Kulzer, Dormagen, Germany). These cavities were filled either in bulk (H-CF-VB and H-CH-TB) or with two equal increments of 2 mm as horizontal layers (H-CF-FS, H-CF-CD and H-CF-AP). For the bulk-filling groups, light-curing was done for 40 s with an LED curing device (Bluephase, Ivoclar Vivadent, Schaan, Liechtenstein; 800 mW/cm 2 ). For the incremental filling groups, light-curing was done twice for 20 s after each placement of hybrid composite. The resulting C-factor of the high C-factor cavity group was 6.33. The restorations were stored in distilled water at room temperature.
Code | Product | Manufacturer | Base resin | Filler (wt/vol.%) |
---|---|---|---|---|
FS | Filtek Supreme | 3M ESPE, St Paul, MN, USA | Bis-GMA/EMA, UDMA | 78.5/59.5% |
CD | Charisma Diamond | Heraeus Kulzer, Dormagen, Germany | Bis-GMA | N.A/58% |
AP | Amelogen Plus | Ultradent, South Jordan, UT, USA | Bis-GMA, TEGDMA | 76/61% |
TB | Tetric N-ceram bulkfill | Ivoclar Vivadent, Schaan, Liechtenstein | Bis-GMA, UDMAdimethacrylate co-monomers | 78/55% (including prepolymer) |
VB | Venus Bulk Fill | Heraeus Kulzer, Dormagen, Germany | UDMA, EBPDMA | 65/38% |
The low C-factor cavity group (L-CF) was also divided into five subgroups ( n = 10). The same adhesive was applied to each specimen, dried, and light-cured. To make the specimens of equal volume, a custom silicone mould was used. The silicone mould has a hollow cylinder inside of which the internal dimension is 3 mm in diameter and 3 mm in height. After the mould was placed on the top of the occlusal surface, the same five subgroups as Group high C-factor were made. The low C-factor group cavities were filled with composite either in bulk (L-CF-VB and L-CF-TB) or with two equal increments of 2-mm horizontal layers (L-CF-FS, L-CF-CD and L-CF-AP). For the bulk-filling groups, light-curing was done for 40 s with the same LED curing device as in the high C-factor group. For incremental filling groups, light-curing was done twice for 20 s each after each placement of composite. After light-curing, the same dimension (3 mm in diameter, 4 mm in height cylinder) of restoration was obtained as that of the high C-factor cavity group. The resulting C-factor of the low C-factor cavity group was 0.467. The restorations were stored in distilled water at room temperature.
2.3
Thermo-mechanical load cycling
After 24-h water storage, the specimens were mechanically loaded using a chewing simulator CS-4.8 (SD Mechatronik, Feldkirchen-Westerham, Germany). They were thermo-cycled under thermodynamic condition (5 °C–55 °C), with a dwell-time of 60 s and a transfer time of 24 s and mechanical load of 5 kgf (49 N) for 120,000 times simultaneously. The conical-shaped opposing plunger, which was made of nickel-chromium, was initially positioned at the buccal surface. No direct contact was made to the resin restoration. A 5-kgf load was applied to press down on the buccal surface of the tooth. The rod moved 6 mm vertically and 0.3 mm horizontally. The rising speed was 55 mm/s; the descending speed was 30 mm/s. After the thermo-mechanical loading, the samples were stored in distilled water at room temperature.
2.4
SS-OCT imaging
The SS-OCT used in this study was the Santec OCT-2000 (Santec Co., Komaki, Japan). It is a frequency domain OCT system, integrating a high-speed frequency sweeping external cavity. The laser probe power is less than 20 mW. The light source in the system sweeps the wavelengths from 1260 nm to 1360 nm at a scan rate of 20 kHz. The system analyses the frequency components of backscattered light from the internal structure of an object, thus creating real-time 2D images. The photo-detected signal is amplified and sent to the computer. The axial resolution of the OCT system is 11 μm in air, which is equivalent to 8 μm in soft tissue and 7 μm in oral hard tissue and resin composite, assuming a refractive index of about n = 1.5. The lateral resolution is about 17 μm and the output is an image of 512 × 1024 pixels .
The imaging depth of the SS-OCT laser through the majority of dental composites is known to be less than 3 mm. Before taking SS-OCT images, the upper 2-mm part of the tooth or composite material was cut off of all the specimens. All the specimens were cut at the top 2 mm of the restoration with a diamond disc ( Fig. 1 ). The handheld scanning probe connected to the SS-OCT was placed over the top of the restorations and was oriented at a right angle to the occlusal surface of the restoration. The light beam was projected onto the resin restoration and scanned across the area. The first SS-OCT image of the restoration was taken parallel to the bucco-lingual plane of the cavity at the centre of the restoration. After the image was taken, the platform holding the specimen was rotated 15° clockwise, then the next image was taken. Twelve images were taken of each sample ( Fig. 1 , right end).
2.5
Measuring internal adaptation on SS-OCT image
The SS-OCT images were imported into the ImageJ program. It is known that when there is a microgap between two media of different refractive indices, the reflections of light at the interface will be dissimilar . The presence of air, which means a microgap between the tooth material and restorative material, is visualized as a bright spot on the SS-OCT image. The high signal intensity at the resin-dentin interface created bright spots or clusters ( Figs. 2 and 3 ). To measure the imperfect margins of the cavity floor, the length of the bright spots on the cavity floor was measured. The cavity floor part where signal intensity of the plot profile in ImageJ was above the threshold was thought to be imperfect margins . The percentage of imperfect margin (%IM) was defined as the percentage from dividing the sum of portions where signal intensities were above the threshold by the entire length of a floor. If %IM increases, it means that composite restoration has poor internal adaptation. GapAnalyzer, which is a plug-in software for ImageJ, was used to verify the imperfect margin and was the same method used by Bista et al. . It can show a spot or cluster where the signal intensity was above the threshold as white dots (right lower insets in Figs. 2 and 3 ).
2.6
Measuring the linear polymerization shrinkage percentage of resin composite (LS)
Polymerization shrinkage strain was measured using a custom-made Linometer (R&B Inc., Daejon, Korea). Glycerin gel was applied to a metallic disc and a piece of glass slide to prevent adhesion to the resin. Then, 18.85 mm 2 of resin composite was placed on the thin metallic disc, covered with a glass slide and positioned in place under constant pressure using a screw. An LED-type light-curing unit (Bluephase, Ivoclar Vivadent, Schaan, Liechtenstein) with a power density of 800 mW/cm 2 was placed 1 mm above the glass slide, and the material was light-cured for 30 s. As the resin composite under the glass slide was cured, it moved the aluminum disk downward. The amount of disk displacement was measured using an eddy current sensor every 0.5 s for a period of 180 s. This measurement was repeated five times for each material and the average was calculated.
2.7
Measurement of the polymerization shrinkage stress (PS0 and PS)
The polymerization shrinkage stress of the resin composite was measured using a custom-made device ( Fig. 4 ) and software (R&B Inc., Daejon, Korea). The instrument was driven by a motor and was devised to move a metal bar up and down. The polymerization shrinkage stress applied to the acrylic tension rod was measured by a load cell (100 kgf) connected to the bar. The light to polymerize the resin composite sample was projected from beneath the transparent disc. The entire process was controlled by the software made by R&B Inc.
Before connecting the acrylic rod, its surface was roughened with sandpaper (180 grit), treated with adhesive resin (bonding agent, Clearfil SE bond, Kuraray Noritake Dental, Okayama, Japan) and light-cured. For the measurement of the polymerization stress under a zero-compliance condition (PS0), 7.07 mm 2 of resin composite was placed onto an acrylic disc, and the upper acrylic rod was positioned to ensure that the thickness of the specimen was 1 mm and its diameter was 3 mm ( Fig. 4 (a)). Thus, the C-factor ( d /2 h ; d : diameter, h : height) was 1.5. The stress measurement between the tension rod and the resin composite was set to zero before light curing. Then, the resin composite was light-cured with a light-curing unit (Bluephase, Ivoclar Vivadent, Schaan, Liechtenstein, 800 mW/cm 2 ) for 20 s through the transparent disc. While shrinkage stress developed, the displacement of the acrylic tension rod from resin polymerization was restricted for the zero-compliance measurement. In this mode, as soon as the sensor detected the linear displacement of more than 1 μm during the polymerization process, the program in the machine made the opposing rod move backward to keep the same distance that had been set at the very beginning ( Fig. 4 (b)). Along with the load-cell signal, the polymerization stress was continuously recorded to the computer every 0.1 s for a period of 180 s. This measurement was repeated five times for each material and calculated for average value.
The polymerization stress under the compliance-allowed condition (PS), using the same amount of resin composite, was also gauged. Before measurements, the setup was switched to compliance-allowed mode, in which the acrylic rod could be followed according to the polymerization shrinkage ( Fig. 4 (c)). The compliance of the system was 0.3 μm/N when the acrylic road was used. After 7.07 mm 2 of resin composite was placed onto an acrylic disc, the upper acrylic rod was positioned to ensure that the thickness of the specimen was 1 mm and its diameter was 3 mm. During the light-curing, the shrinkage stress caused displacement of the acrylic tension rod, which was connected to the load cell. Along with the load-cell signal, the displacement and stress was continuously recorded to the computer every 0.1 s for a period of 180 s. This measurement was repeated five times for each material and calculated for average value.
2.8
Statistical analysis
To compare the %IM results between high C-factor and low C-factor groups, an unpaired t -test was used. For the comparison of subgroups within each high and low C-factor group, one-way ANOVA with Scheffe analysis was applied. For the relationships between polymerization parameters and %IM, a Pearson correlation test was done among the variables: LS, PS0, PS, %IM of H-CF and %IM of L-CF. The statistical analysis was conducted by PASW statistics 18 software (SPSS for Windows: SPSS Inc., Chicago, IL, USA).
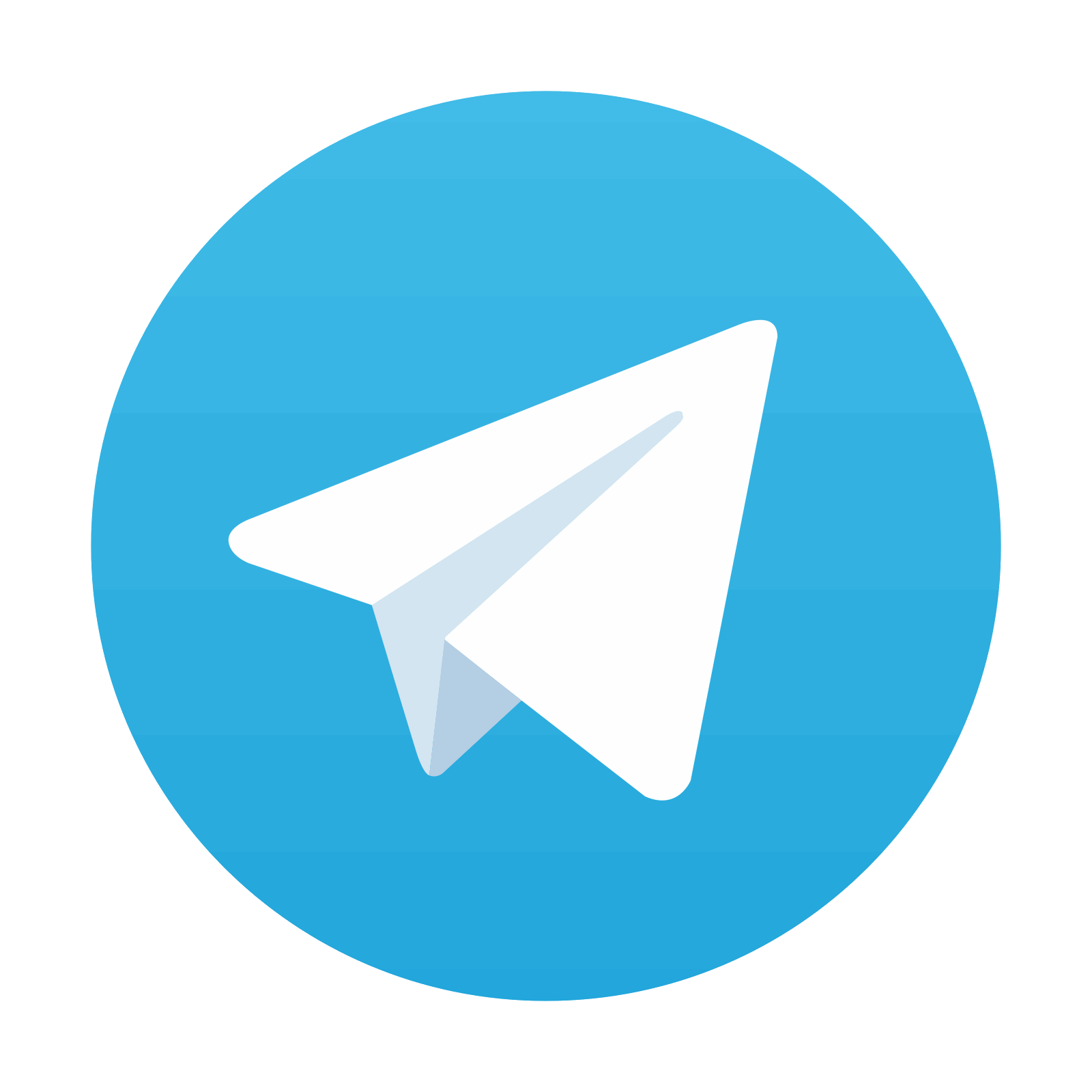
Stay updated, free dental videos. Join our Telegram channel

VIDEdental - Online dental courses
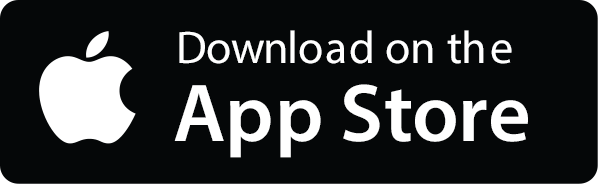
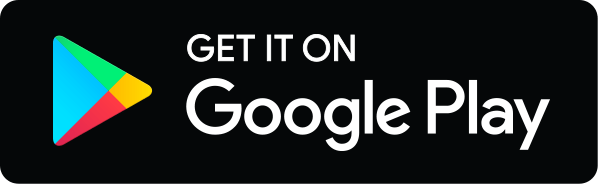