div epub:type=”chapter” role=”doc-chapter”>
S. Stübinger et al. (eds.)Lasers in Oral and Maxillofacial Surgeryhttps://doi.org/10.1007/978-3-030-29604-9_3
3. Laser–Tissue Interaction
Abstract
Since their invention, lasers have been successfully employed in many applications. The basic principle of the interaction of laser with biological tissue is explained, and how many factors may influence the results of the interaction are also discussed. The tissue optical properties, the primary factors of laser interactions, including absorption and scattering, are defined. Other factors, i.e., photochemical, photothermal, photoablation, plasma-induced ablation, and photodisruption, are also discussed.
LasersTissue optical propertiesAbsorptionScatteringPhotochemicalPhotothermalPhotoablationPlasma-induced ablationPhotodisruption
3.1 Introduction
Many different interactions might happen when a laser is impinging onto biological tissues. The laser parameters as well as tissue characteristics play a critical role in this diversity. In this chapter, we discuss the tissue optical properties which are essential for laser–tissue interaction. Tissue thermal properties—such as heat conduction and heat capacity—are also discussed in this chapter. On the contrary, the laser parameters, such as wavelength, exposure time, applied energy, focal spot size, energy density, and power density, are also discussed. As we will find later on in this chapter, the exposure time is a critical parameter when choosing the type of interactions. There is an unlimited number of possible combinations for the experimental parameters. However, mainly five categories of interaction types are classified today. These are photochemical interactions, thermal interactions, photoablation, plasma-induced ablation, and photodisruption. In this chapter, we thoroughly discuss each of these interaction mechanisms.

Laser–tissue interaction map. Colored circles are rough estimation of the associated laser parameters. (Modified from [1])
3.2 Optical Properties of Tissue
In laser–tissue interaction, it is important to know about the absorbing and scattering properties of tissues. The purpose is to have better prediction of successful treatment. When we apply laser onto a highly reflecting materials, the index of refraction might be of interest. In general, we do not assume any tissue optical properties unless specified in tables or graphs. We emphasize more in the general physical interaction which mostly apply to solid and liquid. In reality, there are limitations given by the inhomogeneity of biological tissue to predict the optical properties.
3.2.1 Absorption
The intensity of light is attenuated during absorption by the biological tissue. The absorbance of tissue is defined as the ratio of absorbed and incident light intensities. A partial conversion of light energy into heat motion or certain vibrations of molecules of the absorbing material governs the process of absorption. A perfectly transparent medium which has no absorption will transmit the total radiant energy entering into such medium. In visible range of light, the cornea and lens can be considered as transparent media. In contrast, when the media absorb all the incident radiation, it is called opaque .
The terms “transparent” and “opaque” are very wavelength-dependent. This term depends on the main absorber inside the biological tissue. The cornea and lens, for instance, mainly consist of water which is highly absorbing in the infrared region, will appear opaque in the infrared region but transparent in the visible region. There is no medium known to be either transparent or opaque to all wavelengths of light.
General absorption is being considered if the substance reduces the intensity of all wavelengths by a similar fraction. If we considered the visible region, this substance would appear gray to our eyes. Colors actually originate from selective absorption. Basically, we can divide color as surface and body colors. Surface color is originated from surface reflection. Body color is originated from backscattering light that experiences multiple absorption and scattering inside subsurface of the substance.

where z is the sample optical thickness, I(z) is the intensity at a distance z, I0 is the incident intensity, and μa is the absorption coefficient of the medium.
In biological tissues, absorption is mainly caused by either water molecules or macromolecules such as proteins and pigments, whereas absorption in the IR region of the spectrum can be primarily attributed to water molecules, proteins, and pigments mainly absorb in the UV and visible range of the spectrum. Proteins, in particular, have an absorption peak at approximately 280 nm [1].

Absorption spectra of melanin in the skin and hemoglobin (HbO2) in blood. Relative absorption peaks of hemoglobin are at 420, 540, and 580 nm. (Data according to [2])
As already previously stated, hemoglobin is predominant in vascularized tissue. Krypton ion lasers at 531 nm and 568 nm, respectively, have almost perfectly matched wavelength with the absorption peaks of hemoglobin. Thus, these lasers can be used to coagulate blood and blood vessels. Dye lasers may also be a choice for laser treatment since their tunability can be advantageously used to match particular absorption bands of specific proteins and pigments. In some applications, special dyes and inks are used to provide enhanced absorption. Thus, we can increase specific tissue absorption which leads to better laser treatment. Moreover, we will have less damage to the adjacent tissue due to this enhanced absorption.
3.2.2 Scattering

where z is the sample optical thickness, I(z) is the intensity at a distance z, I0 is the incident intensity, and μs is the scattering coefficient of the medium.
The exact origins of scattering in tissue are not well known. Biological tissue is acomplex and highly heterogeneous material. There are a number of hypotheses identifying contributions to tissue scattering from various biological and biochemical microstructures, both extracellular such as collagen fibers [4, 5] and intracellular such as mitochondria [6], cell nuclei [7], and possibly a large variety of other structures such as cell membranes [8].
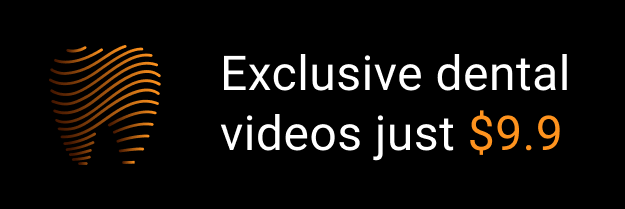