Abstract
Objectives
Bonding of all-ceramic restorations has become routine practice mainly due to results from clinical studies. Sandblasting has been advocated for surface treatment of many ceramics, based on results from numerous in vitro studies. This present work examines the influence of bonding on fracture loads of a veneered alumina ceramic using both chemical and sandblasting treatments.
Methods
Veneered tabs (In-Ceram alumina) were cemented (50 μm; ZnPO 4 or Panavia 21) to dentin-analog bases designed to allow water penetration to the cement/dentin interface. 60 specimens were fabricated for 2 weeks storage and another 80 specimens for 6 months storage (water, 37 °C). Half of each group was tested “as-finished” and the other half “as-sandblasted”, each divided between two cements. Specimens were loaded cyclically (20 Hz, 500,000) cycles in water beneath a 3 mm diameter piston. A staircase sensitivity protocol was followed, using a step size of 25 N. Specimens were examined for cracking by transillumination (10×).
Results
Mean failure loads differed significantly between (1) as-finished Panavia versus zinc phosphate at 2 weeks; (2) as-finished versus sandblasted at 2 weeks; and (3) as-finished at 6 months versus 2 weeks (ANOVA, 95% post hoc test). Sandblasted groups did not differ among themselves at either time period or between 2 weeks and 6 months.
Conclusion
Sandblasting was important for maintaining failure loads after 6 months of water storage. Zinc phosphate was equally protective as Panavia for sandblasted groups. Adhesion testing may not replicate clinical stresses important for investigating protective cement effects.
1
Introduction
Clinical evidence exists supporting the importance of adhesive cementation for the long-term success of all-ceramic restorations and prostheses. Sixteen years of clinical data by Malament and Socransky demonstrated that restorations cemented following acid etching exhibited a more favorable survivor function than those not acid etched . Previously, in vivo study of ceramic inlays strongly suggested better clinical survival for restorations cemented with resin cement versus glass ionomer .
Many in vitro studies have suggested that sandblasting of the interior surface of all-ceramic crowns enables adhesive bonding . In particular, chemical adhesion alone did not provide a sufficiently durable bond following 150 days of water storage, sandblasting was required to maintain the bond to this non-etchable ceramic . Presumably, sandblasting the cementation surface creates considerable roughness enabling a strong micro-mechanical bond with resin cements. Some also suggest that resin luting agents providing durable resin bonds significantly strengthen ceramic materials by “healing” minor surface defects caused by air abrasion . Air-particle abrasion (typically with 50 μm alumina) is routinely used by laboratories to remove investment during the fabrication of ceramic restorations. Sandblasting is used to remove excess glass from glass-infiltrated core materials and is also recommended to create a micro-retentive surface and increase the adhesion of the cement . On the other hand sandblasting can introduce surface flaws or micro-cracks that can compromise the strength of the ceramic . Thus, the role of sandblasting on dental ceramics seems to be controversial.
It has apparently been widely accepted that “bond strength” testing, involving shear loading, provide a surrogate measure predictive of phenomena involved in “bonding protection” provided clinically by cements. However, many basic concepts of “shear testing” have been shown to be incorrect, the most basic being that shear stresses calculated by dividing the failure load by the bonded area are incorrect (vastly underestimating true stresses), true shear stresses are distributed in a highly complex pattern and not easily calculated, and tensile stresses are ignored (and can be higher than shear stresses) . Another aspect of “shear bond” testing is that failure might actually initiate from tensile stresses in one of the bonded materials away from the interface . Additionally, complex crack behavior due to mixed stress intensity modes ( K I peel and K II shear) , changes in the lever arm during failure, influence of residual stresses and elastic/plastic properties across the interface may all cause the crack to follow or turn away from the interface for reasons unrelated to the “bond” – leading to meaningless attributions of percent “adhesive” or “cohesive” failure. The clinical message from all the above is reflected in the nearly complete lack of correlation between bond strength data and class V restoration retention rates . This message is further strengthened by the observation that glass ionomer cement clinically out-performs any other dentin bonding material in class V restoration retention, despite its lack of measurable “bond strength” .
In evaluating the bonding protection of cements and surface treatments, a stress state should be used that mimics that seen in clinical failure. Kelly et al., Thompson et al., Quinn et al., and Scherrer et al. analyzed clinically failed ceramic restorations, concluding that failure often originated from the inner surface of cemented crowns by cracks starting from the cement–ceramic interface . In most cases it appears that the stress state at initial crack pop-in results from compressive loads on the occlusal surface causing bending of the ceramic, creating tensile stresses in the ceramic surface at its interface with the less-stiff cement and dentin. The magnitude of the tensile stress is related to both the applied load and the ratio of the elastic moduli of the supporting materials (dentin and cement) and the ceramic . It has been hypothesized that a radial crack initiates and potentially arrests with catastrophic clinical failure involving repeated mastication or off-axis loads . This supposition is consistent with the report from most patients of chewing something soft when their crown actually failed. Thus a meaningful test of ceramic bonding/protection and cements should involve mastication loading, high numbers of low load chewing cycles and in the presence of water . This present research examined the “bonding” effect of two dental cements (one considered “non-bonding”) and two surface conditions under mastication loading following water storage of either 2 weeks or 6 months.
2
Materials and methods
2.1
Specimen preparation for 14 days water storage
Sixty glass-infiltrated alumina core ceramic tabs were made of In-Ceram alumina. Rectangular tabs were cut from partially pre-sintered CEREC blocks (Vita Zahnfabrik, Germany). These tabs were then glass-infiltrated. The final thickness of preliminary core tabs was approximately 0.8 mm to allow final polishing to a thickness of 0.6 mm. Final specimen thickness (0.6 mm) and surface finish (800 grit silicon carbide) were subsequently obtained by expert machining and polishing of both sides of the tabs (BOMAS Machine Specialties, Somerville, MA). Veneering porcelain (VM7 window porcelain, Vita Zahnfabrik, Germany) was added in two firings and a total thickness of 2 mm was achieved by the ceramic machinist. The surface to be veneered was cleaned by ultrasonic cleaning in acetone for 1 min before applying the porcelain veneer. This surface condition was designated “as finished”.
2.2
Specimen preparation for 180 days water storage
Eighty glass-infiltrated alumina core ceramic/veneer specimens were fabricated using the same instruction as preparing specimens for 14 day storage. Technicians at Vita Zahnfabrick, Germany, fabricated all specimens for 180 days water storage.
2.3
Supporting bases
Supporting bases were made from a dentin analog material having contact stress–strain behavior similar to hydrated dentin (woven glass fiber-filled epoxy; NEMA G10, International Paper, Hampton, SC, USA) . Flat G10 bases were 19 mm in diameter, 5 mm thick with five micro-channels for water transport to the intaglio surface ( Fig. 1 ) Micro-channels communicated with a 10 mm × 0.1 mm water reservoir open to the bottom of the base and further served by 4 hemispherical channels in the wall of the base (90° apart) also open to the bottom of the base (0.1 mm radius, 4.5 mm long) to facilitate the flow of the water. These five micro-channels allowed water to access the cementation surface, simulating the condition established by dentinal tubules ( Fig. 1 ). These micro-channels were obdurated with accessory gutta percha points (#40, Henry Schein, Melville, NY) before cementation, with the points being removed prior to water storage.

2.4
Cementation and water storage
Half of the tabs ( n = 70) were sandblasted with 50 μm Al 2 O 3 at 2.5 bar pressure (14 s) at a distance of 10 mm. Tabs with either as-finished or sandblasted surfaces were ultrasonically cleaned in 96% isopropyl alcohol for 3 min before cementing with either zinc phosphate cement (Fleck, Mizzy Inc., Cherry Hill, NJ) or Panavia 21 EX (Kuraray Medical Inc., Okayama, Japan). Fifteen specimens were fabricated for each of four groups for 14 days water storage. Twenty specimens were fabricated for each of four groups for 180 days water storage. Tabs were cemented to bases between the measuring platens of a micrometer adjusted to achieve 50 μm cement thickness. Panavia cement margins were coated with an air blocking gel, as recommended by the manufacturer, and were stored dry at 37 °C for 1 day to enhance polymerization. Following 1 day of dry storage of the 60 2 week specimens from both cement types were stored at 37 °C in distilled water for a minimum of 2 weeks prior to cyclic loading. The 80 specimens from both cement types were stored at 37 °C in distilled water for a minimum of 180 days prior to cyclic loading.
2.5
Fatigue test under cyclic loading
Each cemented specimen was centrally loaded using a 3 mm diameter G10 piston. Cyclic loading was done with a servohydraulic machine (858 Mini BionixII; MTS, Edens Prarie, MN) under load control. Specimens were secured in a base holder at the bottom of a stainless steel well containing distilled water at room temperature. Disks were centrally loaded using the 3 mm diameter piston. A sheet of polyethylene (0.1 mm thick) was placed between the piston and disk to further reduce contact stress concentrations. Sinusoidal cyclic loading was applied to ceramic specimens at a frequency of 20 Hz from 10 N to the target load for 500,000 cycles. Specimens were dried for 2–3 h and then examined for cracking using transilumination. The staircase sensitivity method was chosen for this work . Approximately 4–5 specimens were used to establish the approximate load for 50% probability of fracture. Following this, target loads during testing were determined by the fracture experience of the previous specimen. If the previous specimen survived, the load was increased by one step. Step size was set at 25 N, as determined from previous work to yield a reasonably small standard deviation, as illustrated in Eq. (1) :
SD = 1.62 d ( N − R ) B − A 2 ( N − R ) 2 + 0.029
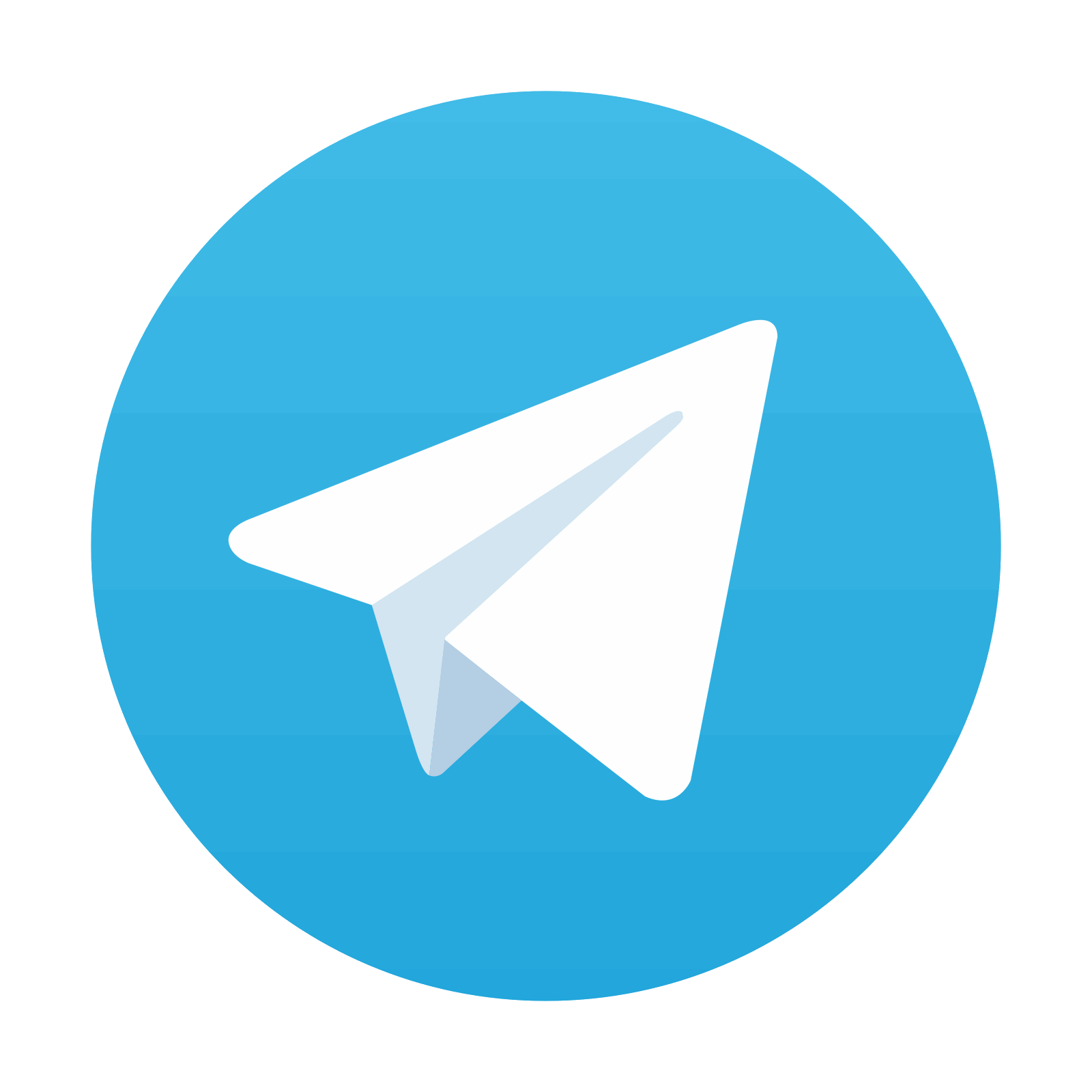
Stay updated, free dental videos. Join our Telegram channel

VIDEdental - Online dental courses
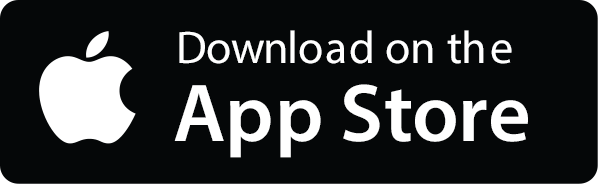
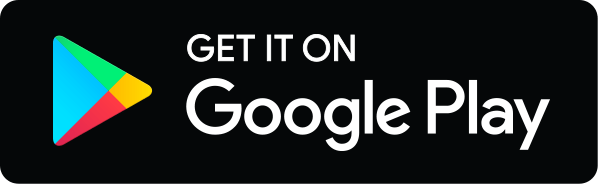