Abstract
Objective
We examined the correlation between clinical wear rates of restorative materials and enamel (TRAC Research Foundation, Provo, USA) and the results of six laboratory test methods (ACTA, Alabama (generalized, localized), Ivoclar (vertical, volumetric), Munich, OHSU (abrasion, attrition), Zurich).
Methods
Individual clinical wear data were available from clinical trials that were conducted by TRAC Research Foundation (formerly CRA) together with general practitioners. For each of the n = 28 materials (21 composite resins for intra-coronal restorations [20 direct and 1 indirect], 5 resin materials for crowns, 1 amalgam, enamel) a minimum of 30 restorations had been placed in posterior teeth, mainly molars. The recall intervals were up to 5 years with the majority of materials ( n = 27) being monitored, however, only for up to 2 years. For the laboratory data, the databases MEDLINE and IADR abstracts were searched for wear data on materials which were also clinically tested by TRAC Research Foundation. Only those data for which the same test parameters ( e.g. number of cycles, loading force, type of antagonist) had been published were included in the study. A different quantity of data was available for each laboratory method: Ivoclar ( n = 22), Zurich ( n = 20), Alabama ( n = 17), OHSU and ACTA ( n = 12), Munich ( n = 7). The clinical results were summed up in an index and a linear mixed model was fitted to the log wear measurements including the following factors: material, time (0.5, 1, 2 and 3 years), tooth (premolar/molar) and gender (male/female) as fixed effects, and patient as random effect. Relative ranks were created for each material and method; the same was performed with the clinical results.
Results
The mean age of the subjects was 40 (±12) years. The materials had been mostly applied in molars (81%) and 95% of the intracoronal restorations were Class II restorations. The mean number of individual wear data per material was 25 (range 14–42). The mean coefficient of variation of clinical wear data was 53%. The only significant correlation was reached by OHSU (abrasion) with a Spearman r of 0.86 ( p = 0.001). Zurich, ACTA, Alabama generalized wear and Ivoclar (volume) had correlation coefficients between 0.3 and 0.4. For Zurich, Alabama generalized wear and Munich, the correlation coefficient improved if only composites for direct use were taken into consideration. The combination of different laboratory methods did not significantly improve the correlation.
Significance
The clinical wear of composite resins is mainly dependent on differences between patients and less on the differences between materials. Laboratory methods to test conventional resins for wear are therefore less important, especially since most of them do not reflect the clinical wear.
1
Introduction
In restorative dentistry, the wear resistance of restorative materials is mainly evaluated with laboratory methods that use an electric device that puts a counterpart object ( e.g. stylus) into contact with the material that is to be tested. Some devices simulate chewing movements that occur in the mouth and some methods use an abrasive medium . The methods differ with regard to the applied force, the material type and movement of the counterpart, the number of cycles, etc. . In 2001 the International Standard Organization ISO published a technical specification “Wear by two- and/or three-body contact” describing 8 laboratory methods without, however, giving further comments on them . Furthermore, discrepancies in wear rates of the same material using the same wear method and wear parameters were found . The variations maybe due to different material batches, light curing conditions or the wear generating device which does not always generate reproducible results.
For contemporary resin materials wear of posterior restorations seems to be no longer a clinical problem, also over a period of ten years or more because it is restricted to contact areas and does not affect the entire restoration with complete loss of the anatomy as it was the case with the early composite resins of the eighties. Wear can be measured on replicas but may not be detected during clinical inspection. Therefore, the issue of wear should not be over-estimated. However, wear is important if materials with new monomers and/or fillers are developed and their wear behavior cannot be deduced from similar products. In this case, laboratory methods may help to assess the wear resistance before the material is evaluated in a clinical trial, whose results will be present only years after the beginning of the study.
Ideally, the laboratory wear rates of specific materials should reflect the wear that is measured in vivo with the same materials. Some methods claim to have a high correlation between in vitro and in vivo wear, but these correlations are only based on a limited number of restorations with mostly 2–3 materials . In addition, they are linearly extrapolated from 6-month data , the clinical wear was measured with inadequate and inaccurate methods such as the Moffa–Lugassy scale or the correlation analysis was carried out with pooled clinical data from studies with different study protocols .
To date, no effort has been undertaken to systematically compare data of laboratory wear methods with clinical wear data. Such an approach has been hampered by the fact that the peer-reviewed dental journals contain very few clinical wear data which were generated by applying a reliable and accurate quantitative wear method . In the nineties, the Technologies in Restorative and Caries (TRAC) Research Foundation (formerly Clinical Research Associates CRA) clinically evaluated many resin materials for both direct and indirect Class II restorations and for indirect crowns and fixed dental prostheses.
The purpose of the present study was to correlate these data with wear data published in the literature gained from the most common and current laboratory wear methods, which tested the same materials. Four of these six selected methods are also part of the ISO Technical Specification . A round-robin test that involved five of the six wear methods examined in the present study revealed that the results from one wear method to another were hardly comparable . The reason for that is that these laboratory wear methods follow different tribological concepts . It can therefore be expected that the correlation to clinical wear is also different. As the clinical wear process is a complex mechanism with different tribological phenomena occurring at the same time, it can be assumed that there is no single laboratory wear method capable of showing a good correlation with clinical wear. However, if two or three methods that come very close to the clinical wear are combined, the correlation may be improved.
The following hypotheses were examined:
- 1.
There is a method-dependent correlation between laboratory wear methods and the clinical wear rates.
- 2.
If two or three wear methods are combined, the correlation can be improved.
2
Materials and methods
2.1
Wear in vivo
Clinical wear data were derived from clinical studies, which the TRAC Research Foundation (formerly CRA) in Provo, USA, organized and carried out together with general practitioners. Some of the results were previously reported at AADR/IADR conferences .
Wear was mainly analyzed in big molar restorations, sometimes including the build-up of a cusp. A minimum of 30 restorations was placed in 30 subjects. The drop-out rate after 2–3 years was about 10%. For composite materials for indirect use, full-coverage crowns were fabricated in especially selected dental laboratories.
A non-surface-contact method was devised to determine the in vivo wear of dental restorations by quantifying the focal distance of a microscope with long focal length (Olympus BH-2, Olympus Optical Co. Ltd., Japan) in sharp focus with a narrow depth of sharpness (about 2 μm) at 200× on the surface of an epoxy replica of the restored tooth . Custom software which was initially developed for SEM mapping provided the following parameters for each sample placed onto the test apparatus: establishment of a zero elevation from which all measurements were made, reference points defining the perimeter of the surfaces to be measured, precise movement of an x – y stage, determination of the point of sharpest focus, and acquisition of x – y – z data every 50 μm over the pre-defined occlusal table of the replica of the restored tooth. The precision of the system was determined by digitizing and fitting the same specimens repeatedly at random times over a period of one year which resulted in an outcome of a ±3 μm difference over fifty runs performed over a twelve-month period. This accuracy is comparable to measurements carried out by Roulet using a measuring stylus .
A human check was performed on each separate profile within each matrix to locate illogical outliers that could form if the system suffered any unusual vibrations, impact, or software glitches during its unsupervised runs at night and over weekends. Six axes of rotation were used along with a best fit algorithm for the fitting of the two matrices digitized representing two different points in time. A color-coded display obtained after digitization was compared very carefully to the high resolution dies as well as the scanning electron microscope photographs of the test teeth to make sure the topography matched perfectly between the three representations. Two operators worked separately and independently to perform final adjustments of all the best-fit analyses, and were required to compare and reach agreement if there were differences. Knowns were constructed by a firm recommended by the National Bureau of Standards, and these knowns were digitized with each die set every time samples were set up and the system was operated to check for system errors. The data from the knowns were subjected to the fit program as well as the digitizing programs to validate all steps in the process. For final analyses, two operators worked independently with every die set fitted. It was required they achieve a final solution within ±5 μm of each other.
The individual data of each subject were available, including age, gender, type of tooth restored, number of restored surfaces, and wear after each recall interval.
2.2
Wear in vitro
The following laboratory wear methods were selected according to their significance and frequency of citation in the literature .
2.3
ACTA-Method
The ACTA-Method has been developed at the Dental College in Amsterdam (The Netherlands) (ACTA = Academisch Centrum Tandheelkunde Amsterdam) . Two metal wheels rotate in different directions with about 15% difference in the circumferential speed while having near contact. The test specimens ( n = 24 and 28, resp.) are placed on the circumference of the wheel while the other wheel serves as antagonist. The force with which the two wheels come together is adjusted to 15 N. The wheels are placed in a slurry of white millet seeds in a buffer solution. After 50,000, 100,000 and 200,000 cycles the maximal vertical loss of the test specimens is measured with a profilometry device.
2.4
Alabama-Method
The Alabama wear method was developed at the University of Alabama (USA) by Leinfelder and Suzuki and is therefore also called Leinfelder wear method. The method has been first published in 1989 and is a modification of a device that has been originally designed by Roulet . Several major modifications were made over the years. In the first publication in 1989, a polyethylene tape was used as intermediate substance, driven by a tape advancing system . The tape was replaced by a slurry of PMMA beads ten years later . The original force was 55 N, which was increased to 75 N ten years later. In the first publication, a stainless steel stylus with 2 mm radius hit the specimen without rotation. In the new method an additional 30° clockwise rotation was integrated as soon as the stylus hits the surface of the specimen which was then called “localized wear” (Alabama loc). Additionally, with a new specimen a flat stylus made of polyacetal is brought into contact with the flat specimen; the wear produced by this approach is called “generalized wear” (Alabama gen). The materials specimens are incorporated into extracted molars that are trimmed flat. The stylus for generalized wear is made of polyacetal, the one for localized wear is stainless steel. The original publication states that each spring is calibrated with a 200 kg load cell in conjunction with a universal testing machine prior to testing, but no data have been reported with regard to the deviations, the scattering of results and the time intervals of force measurements or the replacement frequency of the spring.
2.5
Ivoclar-Method
After processing and before testing, the specimens ( n = 8) are kept dry at a temperature of 37 °C for 24 h. The specimens are mounted in a chewing simulator that is commercially available (CS-4, SD Mechatronik, Germany). Antagonists are made by pressed IPS Empress ceramic (Ivoclar Vivadent) which is glazed two times at a temperature of 870 °C. The diameter of the antagonist is 2.4 mm at a height of 600 μm. A weight of 5 kg is put on each vertical bar. The sliding movement is fixed at 0.7 mm. The frequency of the antagonist movement is 1.6 Hz. A total of 120,000 cycles of unidirectional antagonist movements are carried out. Thermocycling with a frequency of 320/120,000 cycles is included in the wear setting with a temperature difference between 5 °C and 55 °C. After completing the wear generating procedure, replicas are made with white super hard plaster (Fuji Superhard Rock, GC Corporation, Japan). The plaster replicas are analyzed with a commercially available laser scanner device (Laserscan 3D or etkon es 1, Willytec, Germany) and the appropriate Match-3-D-software . The volumetric (Ivoclar vol) as well as the maximal vertical loss (Ivoclar vert) (1% percentile) are calculated by the software.
2.6
Munich-Method
The Munich-Method has been developed at the Maximilian University in Munich (Germany) . After processing the specimens (Ø 7.5 mm, n = 8) were kept in Ringer’s solution for 24 h. After that period the specimens are mounted in a sliding wear tester (Munich Artificial Mouth) with pneumatic cylinders as actuator. The wear tester is configured as a pin-on-block-design allowing the test specimens to slide under permanent contact to the spherical antagonist (Degussit aluminum oxide, 5 mm diameter) at a linear distance of 8 mm and a vertical load of 50 N. During the chewing simulation the specimens are rinsed with distilled water with a temperature of 37 °C. At 6000, 10,000, 30,000, and 50,000 double cycles (bidirectional forth-and back-movement), replicas are made with white plaster (Fuji Superhard Rock, GC Corporation, Japan). The volumetric loss of the wear facet is quantified on the plaster models with the Laserscan 3D device (Willytec, Germany) described above and converted to vertical loss.
2.7
OHSU-Method
This method has been developed at the Oral Health and Science University (OHSU) in Portland (USA) . In principle, enamel cusps are forced into contact with the specimens through a layer of food like slurry (mixture of poppy seeds and PMMA beads). The enamel cusps are drilled out of human upper molars of similar shape giving them a spherical shape of a diameter of 10 mm. The enamel stylus are polished with 600 grit and 1000 grit silicon carbide and polished with 5 μm aluminum oxide paste and then ultrasonically cleaned for 1 min. Before mounting the specimens ( n = 10) in the wear simulator, they are kept in water for 24 h at 37 °C. First the cusp is forced with a load of 50 N on the surface, sliding across a linear path of 8 mm producing abrasive wear. At the end of each path, a static load of 80 N is applied to produce localized attrition wear. For a whole test sequence 100,000 cycles at 1 Hz with unidirectional movements are run. The mean vertical loss of the abrasion and attrition wear facets is measured with a profilometry device at 10 defined tracks. The values of tracks 4–6 correspond to the abrasion wear (OHUS ab) and the tracks 8–9 to the attrition wear facet (OHUS at).
2.8
Zurich-Method
The Zurich wear method has been developed at the University of Zurich (Switzerland) . With a load of 49 N and a frequency of 1.7 Hz the palatal cusps cut out of similar upper molars push against the surface of the specimens ( n = 6) that are mounted on a rubber socket at 45° angle allowing the antagonist to glide over the surface of the test specimen. The test specimens are kept in water with exchanged temperature according to a thermocycling protocol (3000× 5 °C/55 °C). After 120,000, 240,000, 640,000, and 1,200,000 cycles of loading the specimens are submitted to a toothbrushing device with a slurry of toothpaste for 30 min, 30 min, 100 min, and 140 min, resp. . Additionally, at the end of the first phase (120,000 cycles) the specimens are put into a solution of 75% ethanol for 20 h to simulate the chemical degradation. After each thermomechanical sequence, the maximal vertical loss of both the specimens (occlusal contact area OCA) and the antagonists as well as the vertical loss in the contact free area (CFA) are calculated by using a computerized 3D-scanner . The scanner is driven by step motors which scan the object in 1 μm steps in the z -direction and in 100 μm steps in the xy -direction .
The wear methods with their typical parameters are listed in Table 1 .
Wear method | Force | Force actuator | Movement of stylus | Stylus material | Abrasive medium | Number of cycles | Outcome variables |
---|---|---|---|---|---|---|---|
ACTA | 15 N | Spring | Wheel runs against specimens | Metal wheel, slip rate 15% | Millet seed shells/rice | 200,000 | Vertical wear (μm) |
Alabama | 75 N | Spring | 30° rotation | Polyacetal | PMMA beads | 400,000 | Localized wear (μm) |
(Ø 2.5 mm) | |||||||
Polyacetal | Generalized wear (μm) | ||||||
(Ø 6.5 mm) | |||||||
Ivoclar | 5 kg | Dead weight driven with step motor | 0.7 mm | Leucite reinforced ceramic (Empress) | Water | 120,000 | Vertical wear (μm) |
(Ø 2.4 mm) | Volumetric wear (10 7 μm 3 ) | ||||||
Munich | 5 kg | Dead weight driven with pneumatic cylinder | 8 mm | Aluminum oxide ceramic (Degussit) | Water | 50,000 | Vertical wear (μm) |
(Ø 5 mm) | |||||||
OHSU | 20 N abrasion 80 N attrition | Electro-magnetic | 6 mm | Enamel | Poppy seeds/PMMA beads | 50,000 | Mean vertical wear (μm) |
(Ø 10 mm) | Abrasion | ||||||
Attrition | |||||||
Zurich | 49 N | Electro-magnetic | Sliding movement of specimen | Enamel | Water | 120,000 | Vertical wear (μm) |
(Ø 5 mm) | 240,000 | ||||||
640,000 | |||||||
1,200,000 |
Four variables were used in conjunction with the Zurich wear method: Phase 1 after 120,000 loading cycles, phase 2 after 240,000 cycles, phase 3 after 640,000 cycles and phase 4 after 1,200,000 cycles. These values were claimed to correspond to six months, one year, 2.7 years and 5 years of clinical wear, respectively . For the Alabama method two variables had been included in the analysis: generalized and localized wear; for the OHSU method two different wear zones were generated: abrasion and attrition. For the Ivoclar method the vertical as well as the volumetric wear was taken into consideration. A single variable was available for ACTA and Munich (vertical loss after completion of all cycles).
A systematic search was carried out by using the databases of MEDLINE (PUBMED, since 1966) and IADR (abstracts, starting 1984). The search month was March 2008. The search terms were “wear” and/or “abrasion”, “ in vitro ” and the name or acronym of the respective wear method. The wear data were only used if the identical wear method parameters listed in Table 1 were applied. As far as enamel wear is concerned, the test parameters were different to those applied for composite or amalgam materials for the Alabama and OHSU method. The studies included in the comparative analysis are listed in Table 2 .
ACTA | Alabama | Ivoclar | Munich | OHSU | Zurich |
---|---|---|---|---|---|
. |
Although the test parameters are identical, the wear data may be influenced by the fact that different research institutes carried out the tests. Therefore, the studies were grouped into two groups: PUB 1 = from the same institute, namely the ones who first published the method; PUB 2 = other institutes.
The materials were classified into 4 categories:
-
CAT 1: composites for intra-coronal restorations (direct and indirect);
-
CAT 2: composites for crowns and bridges;
-
CAT 3: amalgam;
-
CAT 4: enamel.
2.9
Statistical analysis
In order to summarize the information of clinical measurements, a single index was created for each material. Using an average value of the measurements for each material, however, would not provide a fair comparison between the materials because of the unbalanced design and the increase of wear over time. Instead, a linear mixed model with a fixed material effect, a fixed time effect and a random patient effect was considered as follows:
Y i j k = m i + t j + p k + ε i j k
where Y ijk denotes the logarithm of in vivo measurement of material i at time j for patient k , m i is the effect of material i , t j is the effect of time j , p k is the effect of patient k and ε ijk is an error term; p k and ε ijk were assumed to be independent and normally distributed. The age of the patients could not be considered, because this information was missing for about 43% of the patients. A logarithm transformation was necessary to reach normality. Calculating exp(effect) − 1 provided us with an estimate of the percentage of median wear increase on the original scale. Estimates of material effects m i were then used as a summarizing in vivo index, to be correlated with the various in vitro variables. Further covariates such as gender of the patients or tooth type were also included in the model. Linear mixed models were calculated using the lme routine implemented in the package nlme (version 3.1–83) from the free statistical package R.
In order to be closer to a normal distribution, in vitro variables were log-transformed, as suggested in a previous publication where data of different laboratory methods were compared . To facilitate comparison, the log-values x of each variable were further transformed into relative ranks, calculated as 1 + ( n − 1)( x − m )/( M − m ), where n was the number of materials considered ( n = 28), m was the minimum (mean) and M was the maximum in vitro value (mean). The scale of each variable was hence transformed into a “rank scale” from 1 to K (with all variables being directly comparable), while the relative differences between the materials was taken into account.
To evaluate whether the combination of different laboratory methods improves the correlation with in vivo data, stepwise linear regression modeling was applied (SPSS release 16.0).
2
Materials and methods
2.1
Wear in vivo
Clinical wear data were derived from clinical studies, which the TRAC Research Foundation (formerly CRA) in Provo, USA, organized and carried out together with general practitioners. Some of the results were previously reported at AADR/IADR conferences .
Wear was mainly analyzed in big molar restorations, sometimes including the build-up of a cusp. A minimum of 30 restorations was placed in 30 subjects. The drop-out rate after 2–3 years was about 10%. For composite materials for indirect use, full-coverage crowns were fabricated in especially selected dental laboratories.
A non-surface-contact method was devised to determine the in vivo wear of dental restorations by quantifying the focal distance of a microscope with long focal length (Olympus BH-2, Olympus Optical Co. Ltd., Japan) in sharp focus with a narrow depth of sharpness (about 2 μm) at 200× on the surface of an epoxy replica of the restored tooth . Custom software which was initially developed for SEM mapping provided the following parameters for each sample placed onto the test apparatus: establishment of a zero elevation from which all measurements were made, reference points defining the perimeter of the surfaces to be measured, precise movement of an x – y stage, determination of the point of sharpest focus, and acquisition of x – y – z data every 50 μm over the pre-defined occlusal table of the replica of the restored tooth. The precision of the system was determined by digitizing and fitting the same specimens repeatedly at random times over a period of one year which resulted in an outcome of a ±3 μm difference over fifty runs performed over a twelve-month period. This accuracy is comparable to measurements carried out by Roulet using a measuring stylus .
A human check was performed on each separate profile within each matrix to locate illogical outliers that could form if the system suffered any unusual vibrations, impact, or software glitches during its unsupervised runs at night and over weekends. Six axes of rotation were used along with a best fit algorithm for the fitting of the two matrices digitized representing two different points in time. A color-coded display obtained after digitization was compared very carefully to the high resolution dies as well as the scanning electron microscope photographs of the test teeth to make sure the topography matched perfectly between the three representations. Two operators worked separately and independently to perform final adjustments of all the best-fit analyses, and were required to compare and reach agreement if there were differences. Knowns were constructed by a firm recommended by the National Bureau of Standards, and these knowns were digitized with each die set every time samples were set up and the system was operated to check for system errors. The data from the knowns were subjected to the fit program as well as the digitizing programs to validate all steps in the process. For final analyses, two operators worked independently with every die set fitted. It was required they achieve a final solution within ±5 μm of each other.
The individual data of each subject were available, including age, gender, type of tooth restored, number of restored surfaces, and wear after each recall interval.
2.2
Wear in vitro
The following laboratory wear methods were selected according to their significance and frequency of citation in the literature .
2.3
ACTA-Method
The ACTA-Method has been developed at the Dental College in Amsterdam (The Netherlands) (ACTA = Academisch Centrum Tandheelkunde Amsterdam) . Two metal wheels rotate in different directions with about 15% difference in the circumferential speed while having near contact. The test specimens ( n = 24 and 28, resp.) are placed on the circumference of the wheel while the other wheel serves as antagonist. The force with which the two wheels come together is adjusted to 15 N. The wheels are placed in a slurry of white millet seeds in a buffer solution. After 50,000, 100,000 and 200,000 cycles the maximal vertical loss of the test specimens is measured with a profilometry device.
2.4
Alabama-Method
The Alabama wear method was developed at the University of Alabama (USA) by Leinfelder and Suzuki and is therefore also called Leinfelder wear method. The method has been first published in 1989 and is a modification of a device that has been originally designed by Roulet . Several major modifications were made over the years. In the first publication in 1989, a polyethylene tape was used as intermediate substance, driven by a tape advancing system . The tape was replaced by a slurry of PMMA beads ten years later . The original force was 55 N, which was increased to 75 N ten years later. In the first publication, a stainless steel stylus with 2 mm radius hit the specimen without rotation. In the new method an additional 30° clockwise rotation was integrated as soon as the stylus hits the surface of the specimen which was then called “localized wear” (Alabama loc). Additionally, with a new specimen a flat stylus made of polyacetal is brought into contact with the flat specimen; the wear produced by this approach is called “generalized wear” (Alabama gen). The materials specimens are incorporated into extracted molars that are trimmed flat. The stylus for generalized wear is made of polyacetal, the one for localized wear is stainless steel. The original publication states that each spring is calibrated with a 200 kg load cell in conjunction with a universal testing machine prior to testing, but no data have been reported with regard to the deviations, the scattering of results and the time intervals of force measurements or the replacement frequency of the spring.
2.5
Ivoclar-Method
After processing and before testing, the specimens ( n = 8) are kept dry at a temperature of 37 °C for 24 h. The specimens are mounted in a chewing simulator that is commercially available (CS-4, SD Mechatronik, Germany). Antagonists are made by pressed IPS Empress ceramic (Ivoclar Vivadent) which is glazed two times at a temperature of 870 °C. The diameter of the antagonist is 2.4 mm at a height of 600 μm. A weight of 5 kg is put on each vertical bar. The sliding movement is fixed at 0.7 mm. The frequency of the antagonist movement is 1.6 Hz. A total of 120,000 cycles of unidirectional antagonist movements are carried out. Thermocycling with a frequency of 320/120,000 cycles is included in the wear setting with a temperature difference between 5 °C and 55 °C. After completing the wear generating procedure, replicas are made with white super hard plaster (Fuji Superhard Rock, GC Corporation, Japan). The plaster replicas are analyzed with a commercially available laser scanner device (Laserscan 3D or etkon es 1, Willytec, Germany) and the appropriate Match-3-D-software . The volumetric (Ivoclar vol) as well as the maximal vertical loss (Ivoclar vert) (1% percentile) are calculated by the software.
2.6
Munich-Method
The Munich-Method has been developed at the Maximilian University in Munich (Germany) . After processing the specimens (Ø 7.5 mm, n = 8) were kept in Ringer’s solution for 24 h. After that period the specimens are mounted in a sliding wear tester (Munich Artificial Mouth) with pneumatic cylinders as actuator. The wear tester is configured as a pin-on-block-design allowing the test specimens to slide under permanent contact to the spherical antagonist (Degussit aluminum oxide, 5 mm diameter) at a linear distance of 8 mm and a vertical load of 50 N. During the chewing simulation the specimens are rinsed with distilled water with a temperature of 37 °C. At 6000, 10,000, 30,000, and 50,000 double cycles (bidirectional forth-and back-movement), replicas are made with white plaster (Fuji Superhard Rock, GC Corporation, Japan). The volumetric loss of the wear facet is quantified on the plaster models with the Laserscan 3D device (Willytec, Germany) described above and converted to vertical loss.
2.7
OHSU-Method
This method has been developed at the Oral Health and Science University (OHSU) in Portland (USA) . In principle, enamel cusps are forced into contact with the specimens through a layer of food like slurry (mixture of poppy seeds and PMMA beads). The enamel cusps are drilled out of human upper molars of similar shape giving them a spherical shape of a diameter of 10 mm. The enamel stylus are polished with 600 grit and 1000 grit silicon carbide and polished with 5 μm aluminum oxide paste and then ultrasonically cleaned for 1 min. Before mounting the specimens ( n = 10) in the wear simulator, they are kept in water for 24 h at 37 °C. First the cusp is forced with a load of 50 N on the surface, sliding across a linear path of 8 mm producing abrasive wear. At the end of each path, a static load of 80 N is applied to produce localized attrition wear. For a whole test sequence 100,000 cycles at 1 Hz with unidirectional movements are run. The mean vertical loss of the abrasion and attrition wear facets is measured with a profilometry device at 10 defined tracks. The values of tracks 4–6 correspond to the abrasion wear (OHUS ab) and the tracks 8–9 to the attrition wear facet (OHUS at).
2.8
Zurich-Method
The Zurich wear method has been developed at the University of Zurich (Switzerland) . With a load of 49 N and a frequency of 1.7 Hz the palatal cusps cut out of similar upper molars push against the surface of the specimens ( n = 6) that are mounted on a rubber socket at 45° angle allowing the antagonist to glide over the surface of the test specimen. The test specimens are kept in water with exchanged temperature according to a thermocycling protocol (3000× 5 °C/55 °C). After 120,000, 240,000, 640,000, and 1,200,000 cycles of loading the specimens are submitted to a toothbrushing device with a slurry of toothpaste for 30 min, 30 min, 100 min, and 140 min, resp. . Additionally, at the end of the first phase (120,000 cycles) the specimens are put into a solution of 75% ethanol for 20 h to simulate the chemical degradation. After each thermomechanical sequence, the maximal vertical loss of both the specimens (occlusal contact area OCA) and the antagonists as well as the vertical loss in the contact free area (CFA) are calculated by using a computerized 3D-scanner . The scanner is driven by step motors which scan the object in 1 μm steps in the z -direction and in 100 μm steps in the xy -direction .
The wear methods with their typical parameters are listed in Table 1 .
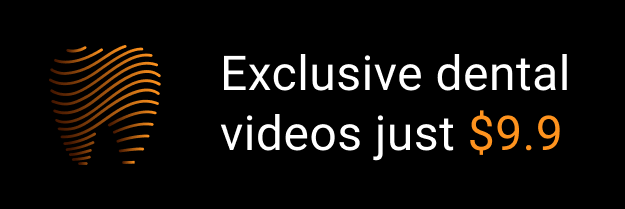