The use of osseointegrated dental implants has gained momentum, mainly in the last 20 years. Research and development in the field of implantology are constantly focusing on implant redesign to continue to try and improve implant success. The current aim of implant design is to address situations prone to failure, such as cases of low bone quality or cases of concomitant systemic diseases that compromise healing.
Since Branemark coined the term osseointegration following his 1952 accidental discovery, the use of osseointegrated dental implants has gained momentum, mainly in the last 20 years. Dental implants are now the state of the art for dental restorative therapy. Yet, research and development in the field of implantology are constantly focusing on implant redesign (eg, topography, implant surface, macro-design) to continue to try and improve implant success. The current aim of implant design is to address situations prone to failure, such as cases of low bone quality or cases of concomitant systemic diseases that compromise healing such as diabetes mellitus.
As opposed to other implants used throughout the human body, the dental implant presents with a unique dual complexity. The implant must engage bone to become osseointegrated but also presents outside the body in the oral cavity connected to a prosthetic tooth.
Today, there are over 1300 dental implant types, each with differing properties: size, shape, and surface. Emerging new developments are based mainly on modification of either chemical or mechanical properties of an implant. Thus, it is expected to improve host tissue response and accelerate the healing process.
Clinical findings suggest that these changes, particularly in implant surface design, have contributed immensely to dental implant success. Nevertheless, implant macrotopography also has made a contribution.
The aim of this article is to present a comprehensive review of current innovations in dental implant design and discuss variations in bone stock that may impact a clinician’s implant selection.
Dental implant surface micro-topography
Since the mid-1980s, a rationale for roughened titanium surfaces compared with turned titanium (smooth) has been formalized.
Roughened titanium surfaces appear to provide micromechanical locking at the micrometric scale and at the same time enhance superior osseointegration by absorption of ions and proteins after implant tissue contact has been achieved, while smooth surfaces do not. Moreover, implant design and surface technology may have an influence on marginal bone response during immediate functional loading.
Several experimental investigations have demonstrated that bone response is influenced by implant surface. Relatively smooth (S(a) <0.5 μm) and minimally rough (S(a) 0.5–1 μm) surfaces showed less strong bone responses than rougher surfaces. However, this microarchitecture does not substantially contribute to primary implant stability.
Immediate loading is among the most innovative techniques used in implant therapy today. The biomechanical outcome of various designs and surfaces that claim to shorten implant treatment is the focus of intense academic debate. The biomechanical outcome of various designs and surfaces claims to shorten implant treatment time. One animal study showed that the highest mean removal torque values were recorded with a bone screw with a wide thread profile (titanium plasma spray coating) at 24.4 Newton per centimeters (NCm)/mm followed by thread profiles that were sandblated and acid-etched at 22.3 NCm/mm. A low thread profile (anodic oxidized surface) was next at 18.7 NCm/mm. The lowest score was obtained by low thread (double-etched and machined surface) at 12.0 NCm/mm. The authors concluded that immediate loading was possible with various designs and surfaces, but when high primary stability was needed, an aggressive thread profile was best.
A retrospective human study concluded that there was no impact of design or surface treatment on implant survival in the completely edentulous mandible where most immediate load experience has been reported clinically. Bone preservation in immediately loaded implants in the mandible appears to be influenced by implant design and was significantly better on surface-modified AstraTech implants (Astra Tech AB, Mölndal, Sweden) compared with machined Branemark implants (Branemark Implant System, Nobel Biocare, Zürich-Flughafen, Switzerland). In the mandible, a microthread design of the implant collar did not seem to improve bone preservation.
One thing to consider in implant design is bone quality. If the mandible is taken as a baseline example, according to Lekholm and Zarb, there may be 4 different types of bone present, from high cortical content (type 1) to poorly mineralized, even osteoporotic (type 4).
Fig. 1 shows a schematic of these proposed bone quality differences. Although the relationship of the drill preparation to the diameter of the implant is very important, implant insertion may be optimized by using bone screw designs that are specifically made to address bone type. Examples of 4 different implants are illustrated in Fig. 1 , including their use in angulated placement strategies. Suggested by the schematic is that the surgeon may make implant selection based on clinical criteria of operative bone density.
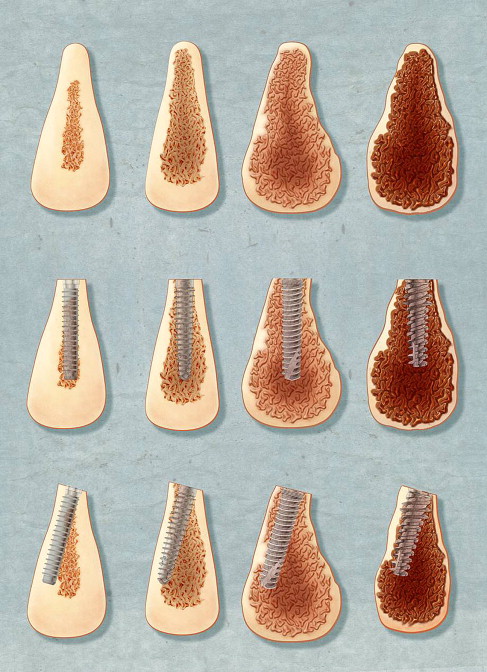
Despite the varied implant designs available for use in different bone density situations, the surgeon can most often adapt techniques to control insertion torque and therefore primary stability no matter what implant type is used. For example, by using a 1° to 2° tapered implant, placed with a straight, often stepped-down bur, implants can gain fixation in both hard and soft bone provided the implant has self-tapping threads for hard bone. By underpreparation, poorly mineralized bone can still secure implant fixation. By tapping or the use of hard bone drills (overpreparation) in type 1 bone, most implant designs can still be used to gain fixation by modifying the surgical technique.
In the special situation of use of an implant in osteoid, such as found in newly forming bone in large BMP-2 grafts, an aggressive implant design is better, as the cortex may be absent and mineralization content is often much less than 15%.
An example of the use of an aggressive threaded implant used in an extraction site associated with soft bone for immediate temporization is seen in Figs. 2–8 .
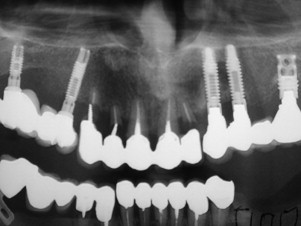
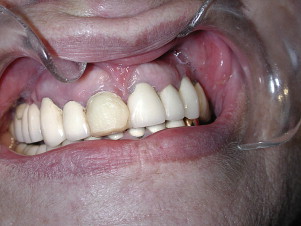
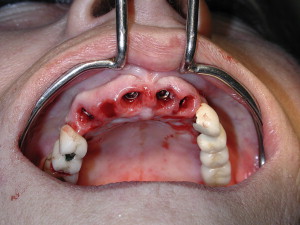
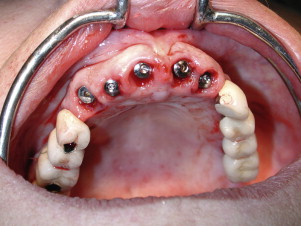
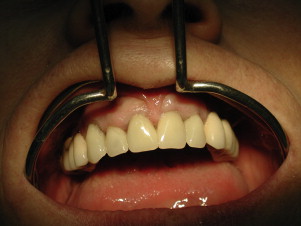
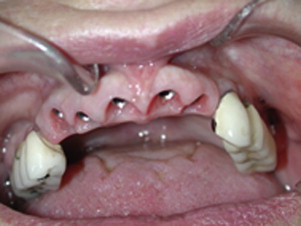
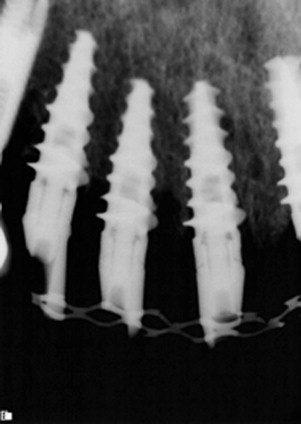
Implant surface chemical properties
The implant surface energy and wetting capability influence the ability to absorb biomolecules, mainly plasma proteins, thus affecting the first phase of osseointegration.
Rough-surfaced implants are known to have hydrophobic properties. When in contact with body fluids, these surfaces show hydrophilic traits, a phenomenon of air microbubble entrapment at the dry surface.
Implant surface properties influence resonance frequency analysis measurements of implant stability. Surface chemistry-modified titanium implants showed higher mean implant stability values than did topographically changed implants. In particular, cation (magnesium)-incorporated micropatterns in magnesium incorporated implants may play a primary role in implant stability.
Implants with nanometer-scale surface topography (NanoTite BIOMET 3i, Palm Beach Gardens, FL, USA) were created by discrete crystalline depositions (DCDs) of calcium phosphate nanocrystals onto a dual acid-etched (DAE) surface. These implants show enhanced early fixation in preclinical studies when compared with DAE-surfaced implants. A clinical study showed a cumulative survival rate of 94.9% after 1 year. Relative to other prospective, multicenter studies of immediately loaded implants with various surface enhancements, NanoTite implants perform comparatively well when immediately provisionalized with single-tooth and fixed restorations.
Surface chemistry also appears to play a significant role in cell response to sandblasted and acid-etched surfaces in the presence of titanium hydride TiH[(2)], which may promote attachment, proliferation, and differentiation of preosteoblasts.
Implant surface chemical properties
The implant surface energy and wetting capability influence the ability to absorb biomolecules, mainly plasma proteins, thus affecting the first phase of osseointegration.
Rough-surfaced implants are known to have hydrophobic properties. When in contact with body fluids, these surfaces show hydrophilic traits, a phenomenon of air microbubble entrapment at the dry surface.
Implant surface properties influence resonance frequency analysis measurements of implant stability. Surface chemistry-modified titanium implants showed higher mean implant stability values than did topographically changed implants. In particular, cation (magnesium)-incorporated micropatterns in magnesium incorporated implants may play a primary role in implant stability.
Implants with nanometer-scale surface topography (NanoTite BIOMET 3i, Palm Beach Gardens, FL, USA) were created by discrete crystalline depositions (DCDs) of calcium phosphate nanocrystals onto a dual acid-etched (DAE) surface. These implants show enhanced early fixation in preclinical studies when compared with DAE-surfaced implants. A clinical study showed a cumulative survival rate of 94.9% after 1 year. Relative to other prospective, multicenter studies of immediately loaded implants with various surface enhancements, NanoTite implants perform comparatively well when immediately provisionalized with single-tooth and fixed restorations.
Surface chemistry also appears to play a significant role in cell response to sandblasted and acid-etched surfaces in the presence of titanium hydride TiH[(2)], which may promote attachment, proliferation, and differentiation of preosteoblasts.
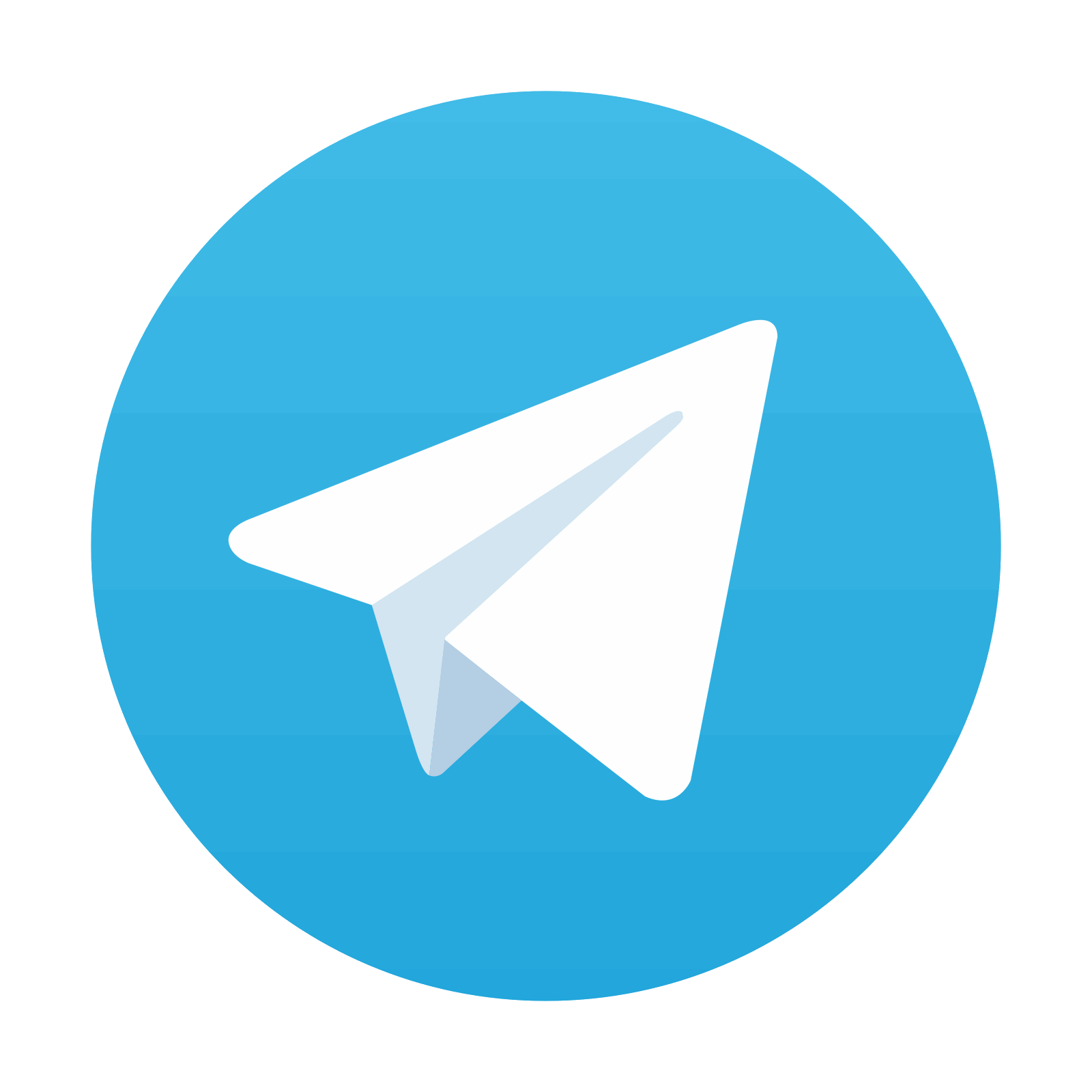
Stay updated, free dental videos. Join our Telegram channel

VIDEdental - Online dental courses
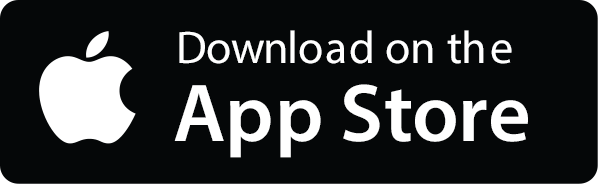
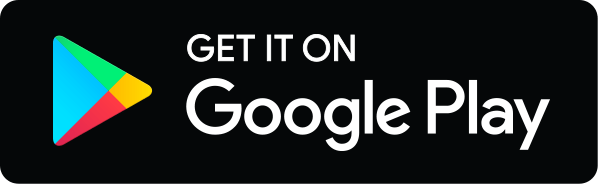