Highlights
- •
The degree of C C conversion was dependent on the CQ:amine.
- •
The influence of viscosity on DC was dependent on the CQ:amine ratio.
- •
The CQ:amine ratio had a significant influence on the yellow–blue axis data.
- •
The overall color stability was only affected by the CQ:amine ratio.
- •
The overall color stability was not affected by the materials’ viscosity.
Abstract
Objectives
To investigate the influence of camphorquinone (CQ):amine ratio on the degree of C C conversion (DC) and color stability of experimental dental composites formulated with different co-monomer viscosities, indirectly determined by variations in the co-monomer ratios.
Materials and methods
Experimental composites were formulated in two different BisGMA:TEGDMA molar ratios (50:50 and 70:30). Viscosities were assessed with a viscometer. For each composite formulation, four different CQ:amine ratios were added: 1:1, 1:2, 1:3 or 1:4 mol%. Materials were loaded with 40 wt% of silanized glass particles. DC was determined by Fourier-transformed infrared spectroscopy with attenuated reflectance mode (ATR-FTIR). A spectrophotometer was used to measure the CIE L * a * b * color coordinates 24 h after polymerization and after 2 months stored in water. Color changes (Δ E ) were calculated. Data were statistically analyzed using analyses of variance (ANOVA), Tukey’s and Student- t tests ( α = 0.05).
Results
The 50:50 BisGMA:TEGDMA co-monomer showed lower viscosity than 70:30. DC was affected by CQ:amine ratio, and not by the co-monomer viscosity, but the interaction between these two factors was significant. a * and b * coordinates were dependent on CQ:amine or BisGMA:TEGDMA ratios, while L * was not. b * values were directly related to the amount of amine in the formulation, regardless of co-monomer viscosity. Δ E was dependent on the amount of amine, but not on the viscosity of the material.
Significance
DC was influenced by the CQ:amine ratio. The influence of viscosity on DC was dependent on the CQ:amine ratio and exhibited distinguished behavior. a * and b * coordinates were affected by CQ:amine and BisGMA:TEGDMA ratios. The color change (Δ E ) was affected by CQ:amine ratio, but not by viscosity.
1
Introduction
Dental resin composites contain inorganic fillers coated by a coupling agent dispersed into a resin matrix commonly composed by a mixture of dimethacrylate monomers. The monomer 2,2-bis[4-(2-hydroxy-3-methacryloyloxypropyl)phenyl]-propane (BisGMA) is a usual component of the resin matrix. BisGMA is high molecular weight monomer with high viscosity due to restrictions imposed by its stiff aromatic backbone and high hydrogen bridging potential resulting from the OH radicals. These characteristics do not allow this monomer to be used alone in the formulation of dental composites. Co-monomers with lower viscosity are used as diluents, particularly triethylenoglycol dimethacrylate
(TEGDMA) .
Current photoactivated dental composites are mainly formulated with camphorquinone (CQ) as photosensitizer. However, its efficiency is dependent on its association with an electron/proton donor substance, usually a tertiary amine . Absorption of light by CQ typically leads to creation of two excited states: (i) the “singlet state”, which does not involve reversal of electron spin, and (ii) the “triplet state”, which is the one relevant to free radical formation, but has a very short half-life . While in the triplet state, CQ may interact with an amine molecule and generate an excited state complex, the “exciplex”. CQ abstracts a hydrogen atom from the tertiary amine resulting in free radical formation . Therefore, factors such as CQ and amine concentrations, their ratio, molecular structure of the amine, and reactivity of the formed radicals to initiate monomer polymerization, all play important roles on the polymerization reaction properties of the resulting polymer . Although a direct correlation between co-initiator concentration and polymerization efficiency might be considered, it is known that above certain concentration limits, amines may act as retarders since excess amine may trap initiating radicals by termination reactions . Excess amine might also cause color instability because of the eventual formation of oxidative products .
Due to the potential problems associated with the CQ:amine combination, alternative photoinitiator systems have been considered . Unfortunately, most of these materials present some drawbacks, such as lower depth of cure , unpredictable color stability or no color improvement when compared to materials using CQ:amine . Consequently, the CQ–amine combination is still the most used photoinitiator system in commercially available resin-based dental materials. Nevertheless, questions on the CQ:amine system still persist and studies have been performed to CLARIFY the CQ ability to leach out from polymerized materials , the toxicity potential of CQ and the influence of CQ and co-initiator concentrations/ratios on a wide range of materials properties, including few reports on the optical characteristics .
In addition to the photoinitiator and co-initiators, the viscosity of the reaction medium might also be an important factor affecting polymerization. It has been demonstrated that at the early polymerization stages of methacrylates the polymer experiences a sudden increase in viscosity, which impairs mobility and leads to a rapid decrease in the termination rate, with reaction becoming diffusion controlled . Viscosity of the reactive medium might also play a significant role on polymerization rate and reaction-diffusion-controlled termination . In such a scenario, materials with higher viscosity might impose limitations for photopolymerization . As CQ needs to react with an amine to create a free radical, it is reasonable to assume that the co-monomer viscosity may impose limitations for the diffusion of the photoinitiator and co-initiator molecules. In a study reporting on the effect of co-initiator ratio on the polymer properties CQ- and phenyl-propanedione-based materials, the authors hypothesized that photoinitiator consumption, and also the resultant optical properties, could be related to co-monomer viscosity, instigating further studies to address such effect .
To date, it seems that there is no study demonstrating the impact of CQ:amine ratio and co-monomer viscosity on the degree of C C conversion (DC) and optical properties of resin-based composites. Therefore, the objective of this study was to evaluate the DC and the color stability of experimental resin composites formulated with different CQ:amine ratios and co-monomer viscosities, tested by variations in the Bis-GMA:TEGDMA ratio. As the high amount of molecules available could benefit the polymerization reaction it is hypothesized that the amine content affects the DC. Also, it is hypothesized that reducing the co-monomer viscosity increases the DC due to reduced limitation for mobility. In addition, as excess amine could suffer oxidative processes and the increased viscosity could impose limitations for molecules to interact, with consequent increase of unreacted CQ and amine molecules, it is hypothesized that high amine content and high co-monomer viscosity decrease color stability.
2
Materials and methods
2.1
Formulation of the experimental materials and viscosity determination
Experimental composites were formulated in two different BisGMA:TEGDMA ratios (Esstech Inc., Essington, USA): the “low-BisGMA” series with 50:50 BisGMA:TEGDMA mol% ratio, and the “high-BisGMA” series with 70:30 BisGMA:TEGDMA mol% ratio. The viscosity of each co-monomer was analyzed with an oscillatory viscometer (Brookfield Engineering, Middleboro, MA, USA) using the regular viscosity mode at 2 rpm. Three measurements were performed for each material. A 0.5% molar fraction of CQ (Sigma–Aldrich, St. Louis, MO, USA) relative to the resin content was added as photosensitizer. The amine co-initiator ethyl 4-dimethylaminobenzoate (EDMAB–Sigma–Aldrich) was added at the following CQ:amine molar concentrations per gram of resin: 0.5:0.5, 1:2, 0.5:1.5, and 0.5:2, that were respectively named as 1:1, 1:2, 1:3 and 1:4 ratios. In total, eight different experimental materials ( Table 1 ) were evaluated.
Material | Organic phase – total 60 wt% | Inorganic phase – total 40 wt% | ||||||
---|---|---|---|---|---|---|---|---|
Monomers (mol %) | Photoinitiator and co-initiator (mol %) | 2-μm BaAlSi | 0.7-μm BaAlSi | 14-nm SiO2 | ||||
BisGMA | TEGDMA | CQ | EDMAB | Ratio | ||||
1 | 50 | 50 | 0.5 | 0.5 | 1:1 | 20 wt% | 20 wt% | 60 wt% |
2 | 1.0 | 1:2 | ||||||
3 | 1.5 | 1:3 | ||||||
4 | 2.0 | 1:4 | ||||||
5 | 70 | 30 | 0.5 | 1:1 | ||||
6 | 1.0 | 1:2 | ||||||
7 | 1.5 | 1:3 | ||||||
8 | 2.0 | 1:4 |
The resin phase was loaded with 40 wt% of silanized filler particles. The inorganic phase was composed by 20 wt% 2-μm barium–aluminum–silicate (Esstech), 20 wt% 0.7-μm barium–aluminum–silicate (Esstech), and 60 wt% 14-nm silicon dioxide particles (Evonik Industries AG, Hanau-Wolfgang, Germany). Table 1 details the materials’ formulation. All photoactivation procedures were carried out with a light-emitting diode (LED) curing unit (RadiiCal; SDI, Bayswater, Victoria, Australia) with 800 mW/cm 2 irradiance.
2.2
Degree of C C conversion (DC)
The DC was evaluated using Fourier-transform mid-infrared spectroscopy (Prestige21; Shimadzu, Tokyo, Japan) with an attenuated total reflectance device composed of a horizontal multiple-reflection ZnSe crystal with 45° mirror angle (Pike Technologies; Madison, WI, USA). A preliminary reading for the unpolymerized material (monomer) was taken under the following conditions: 24 scans co-addition, 4 cm −1 resolution, Happ–Genzel apodization, and 2.8 mm s −1 mirror speed. After the monomer reading, the composite was immediately photoactivated for 40 s. A support was coupled to the spectrometer to hold the curing unit and standardize a 0.5-mm distance between the fiber tip and material. The DC was evaluated in the absorbance mode 10 min after photoactivation. Calculation was carried out using a baseline technique considering the intensity of C C stretching vibration (peak height) at 1635 cm −1 and as an internal standard using the symmetric ring stretching at 1608 cm −1 . Five specimens per material were tested under controlled temperature (25 ± 2 °C) and humidity (45 ± 5%) conditions. %DC was calculated with the following formula:
2.3
Optical properties
The composites were photoactivated for 40 s inside a stainless steel mold (8.7 mm inner diameter, 1 mm thickness) placed between two clear matrix strips ( n = 5). The photoactivated specimens were dry-stored at room temperature (25 ± 1 °C) in the dark. A spectrophotometer (CM2600d; Konica Minolta, Ramsey, NJ, USA) was used to evaluate the CIE L * a * b * coordinates of the specimens. The CIELAB system is composed of three axes or coordinates: L * (lightness, from 0 = black to 100 = white), a * (from − a = green to + a = red) and b (from − b = blue to + b = yellow). The measurements considered D65 illuminant and a 10° observer. Color readings were taken 24 h after photoactivation (baseline) and repeated after the specimens were stored in distilled water at room temperature for 2 months (storage medium changed weekly). The color stability was reported as the color change (Δ E ) after storage using the following equation :
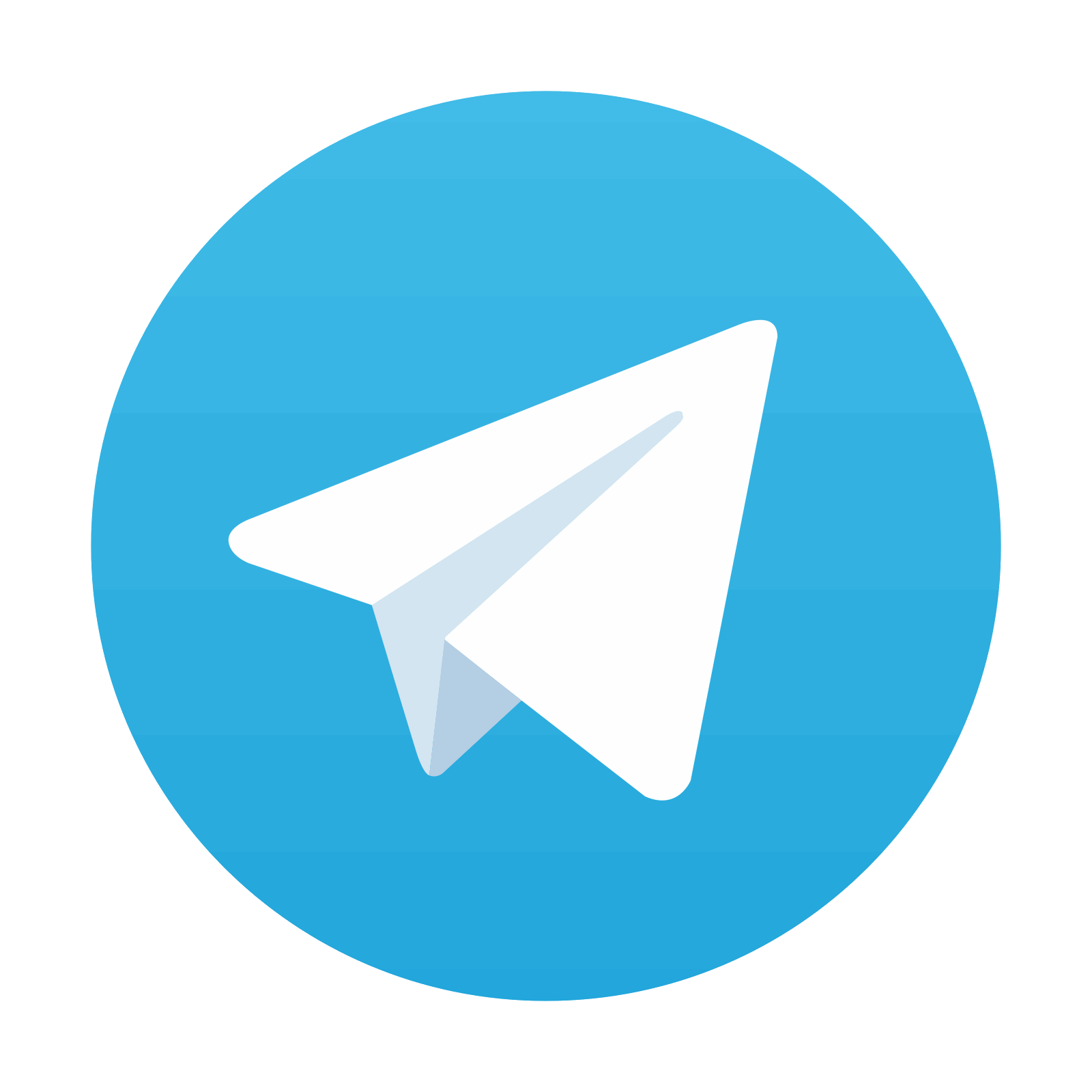
Stay updated, free dental videos. Join our Telegram channel

VIDEdental - Online dental courses
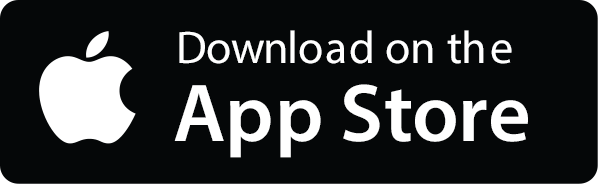
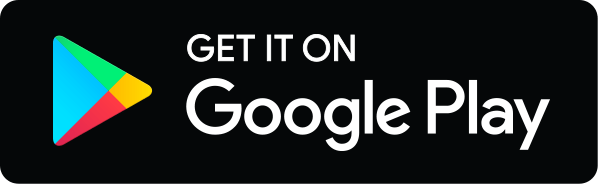