Abstract
Objective
To determine streptococcal adhesion forces with composite resins with different surface roughness.
Methods
Polishing and grinding were applied to obtain smooth (roughness 20 nm), moderately rough (150 nm) and rough (350 nm) surfaces of two orthodontic, light-cured composites. Adhesion forces between Streptococcus sanguinis and Streptococcus mutans and the composite surfaces were measured using atomic force microscopy in absence or presence of a salivary conditioning film. Initial adhesion forces were measured as well adhesion after 120 s of contact, as longer contact times are known to result in stronger adhesion forces (“bond-strengthening”). Surface roughness in absence and presence of salivary conditioning films were compared using ANOVA, while adhesion forces were subjected to a Weibull analysis.
Results
Initial adhesion forces in absence of a salivary conditioning film amounted between −0.7 and −0.9 nN for smooth composite resins and increased between −1.0 and −2.0 nN for the roughest surfaces. Streptococcal adhesion forces after bond-strengthening were significantly stronger than upon initial contact, irrespective of the composite type. Salivary conditioning films significantly decreased the surface roughness of the composites, as well as the streptococcal adhesion forces. Yet, also in the presence of a conditioning film, rougher composite surfaces exerted stronger adhesion forces, irrespective of composite type or bacterial strain.
Conclusion
Streptococcal adhesion forces to orthodontic composite resins increase with increasing roughness of the composite surfaces. Composite surface roughness less affects adhesion forces with S. mutans than with S. sanguinis .
1
Introduction
White spot lesions around orthodontic brackets form one of the most prevalent side effects of orthodontic treatment with fixed appliances, and affect approximately 50% of all orthodontic patients . These lesions can remain visible as a permanent enamel scar or “tooth decay” even 5 years after treatment , compromising facial esthetics after an often lengthy and costly course of orthodontic treatment. The junction between bracket, adhesive and enamel constitutes a favorable place for oral bacteria to adhere and form a biofilm and especially excessive bonding composite around the bracket has been demonstrated to be prone to biofilm accumulation . Lee et al. found that orthodontic composite had a higher ability to retain oral streptococci than metallic brackets . Oral biofilms are only able to maintain position in the oral cavity if the forces by which they adhere are stronger than the prevailing oral detachment forces or the forces exerted by toothbrushing. We have previously investigated oral bacterial adhesion forces to the different materials constituting the bracket–adhesive–enamel junction using atomic force microscopy (AFM) and observed that, in line with the above, different oral bacterial strains adhered more strongly to orthodontic composite resins than to enamel or metal surfaces involved in the junction. It remained unclear though, whether this was due to the hydrophobicity of the composite surface (water contact angle 71°) or its higher roughness (28.5 nm) as compared with enamel (30°; 7.0 nm) or stainless steel (68°; 2.7 nm).
Initial bacterial adhesion on smooth surfaces is reversible and becomes irreversible within seconds to minutes after initial contact . Measurement of bacterial adhesion forces using AFM has demonstrated that longer contact times between a bacterium and a substratum surface results in stronger adhesion forces (“bond-strengthening”) on surfaces in the presence as well as in the absence of a salivary conditioning film . Although it is known that rougher surfaces promote bacterial adhesion to an extend that exceeds the influence of hydrophobicity or surface free energy , the influence of surface roughness on bacterial adhesion forces and their strengthening over time, has not yet been studied. We hypothesize that bacterial adhesion forces and their strengthening over time are dependent on the surface roughness of the composite surfaces. In order to test this hypothesis, we investigated the influence of surface roughness of two different orthodontic composite resins on the adhesion forces of two oral streptococcal strains using AFM. Force measurements were carried out in the absence or presence of an adsorbed salivary conditioning film.
2
Materials and methods
2.1
Bacterial strains and growth conditions
Streptococcus sanguinis ATCC10556 and Streptococcus mutans ATCC700610 were precultured in 10 mL Todd Hewitt Broth (THB, Oxoid, Basingstoke, UK) for 24 h and then inoculated into 200 mL of THB for 16 h at 37 °C. Bacteria were harvested by centrifugation (5 min, 5000 × g , 10 °C), washed twice with demineralized water, and resuspended in demineralized water for AFM.
2.2
Composite resins and salivary conditioning film formation
Two light-cured composite resins, Transbond XT™ (3M Unitek, Monrovia, CA, USA) and PADLock ® (Reliance Orthodontic Products, Inc., Itasca, IL, USA) were included in this study. Transbond XT™ consists of 10–20% Bis-GMA, 5–10% Bis-EMA, 70–80% fillers (silylated quartz and submicron silica) next to <0.2% diphenyliodonium hexafluorophosphate, while PADLock ® contains 8–20% Bis-GMA, 15–60% glass filler and 3–12% amorphous silica, next to 1–3% sodium fluoride, as taken from the respective Materials Safety Data Sheets. Both composite resins are commonly used for bonding of orthodontic brackets to enamel surfaces. Composites were made into 1 cm diameter discs with a thickness of 1 mm by pressing between two glass plates, covered with copier overhead films. Composite discs were cured using a halogen lamp (Optilux 501 Curing Light; Kerr/Demetron, Danbury, CT, USA) for 20 s at a distance of 2 mm from the surface. Light intensity was higher than 400 mW/cm 2 , as verified by a radiometer after every 3 specimens.
Cured discs were used as prepared and after roughening with 0.05 μm wetted aluminum oxide particles (Buehler, Lake Bluff, USA) or with 800 grit wetted sandpaper. Subsequently, discs were cleaned by sonication for 2 × 5 min at 35 kHz in an ultrasonic bath (Transsonic TP 690-A, 154W; Elma, Singen, Germany), and rinsed with demineralized water. X-ray photoelectron spectroscopy (XPS) confirmed the effective removal of grinding and polishing particles from the composite surfaces. In addition, water contact angles were measured in triplicate with a homemade contour monitor and on smooth composite surfaces amounted 80 ± 3° for Transbond XT and 77 ± 8° for PADLock, typical for acrylic surfaces .
Composite discs were used with or without an adsorbed salivary conditioning film. Human whole saliva from 20 healthy volunteers (10–15 mL) of both sexes was collected into ice-chilled flasks after stimulation by chewing Parafilm™. All collections were performed in the morning. After the saliva was pooled and centrifuged twice (10,000 × g , 15 min, 10 °C), phenylmethylsulfonyl fluoride was added to a final concentration of 1 mM as a protease inhibitor in order to reduce protein breakdown and preserve high-molecular weight mucins. Afterwards, the solution was centrifuged again, dialyzed (24 h, 4 °C) against demineralized water, and lyophilized for storage. Note that recently it has been shown that freeze–thawing does not alter a saliva which has been stored at −80 °C for a period of 6 months . Lyophilized saliva was reconstituted in adhesion buffer (2 mM potassium phosphate, 50 mM potassium chloride, and 1 mM calcium dichloride, pH 6.8) at a concentration of 1.5 mg/mL . All volunteers gave their informed consent to saliva donation, in agreement with the policies of the Ethics Committee at the University Medical Center Groningen. Half of all composite discs was immersed in reconstituted human whole saliva for 16 h at 20 °C, after which the discs were dipped three times in demineralized water and immediately used for AFM measurements. The other half of the discs were studied in the absence of a salivary conditioning film and immersed in 100 mL adhesion buffer for 16 h instead of in reconstituted human whole saliva, in order to obtain a comparable degree of surface swelling (if occurring). Salivary conditioning film formation was evidenced by a decrease in water contact angle of the composites to 23 ± 3° (±SD over three different discs), irrespective of the type of composite involved, and an increase in the amount of proteinaceous nitrogen detected by XPS.
2.3
AFM surface roughness measurements
Surface roughnesses of composite discs, with or without a salivary conditioning film, were measured using AFM (see also below) in the contact mode with a silicon nitride cantilever tip (DNP from Veeco, Woodbury, NY, USA). Each disc was imaged at three randomly chosen sites and surface plots were made to provide a three-dimensional perspective of the surface, from which the mean surface roughness ( R a ) was calculated, representing the average distance of the roughness profile to its imaginary center plane. Three different discs were used for the measurements.
2.4
AFM adhesion force measurements
Streptococci from suspension were immobilized on “V”-shaped tipless AFM cantilevers (VEECO, DNP-0, USA). Cantilevers were first immersed in a drop of 0.01% (w/v) poly- l -lysine (Sigma, Poole, UK) for 1 min with a micromanipulator (Leica, Wetzlar, Germany) to create a positive charge on its surface. Subsequently, the cantilever was dried in air for 2 min using the micromanipulator, and then dipped into a drop of bacterial suspension for 1 min to allow bacterial attachment. Each thus prepared bacterial AFM probe was used immediately for further measurement. All AFM measurements were performed in a Dimension 3100 system (Nanoscope IV, Digital Instruments, Woodbury, NY, USA) in the contact mode at room temperature in adhesion buffer, at a scan rate of 1.0 Hz, ramp size 1.5 μm, and trigger threshold of 1 V. The loading force was applied by setting the trigger mode to relative, i.e. a trigger threshold of 1 V, roughly corresponding with a loading force of 12 nN at the inversion point between approach and retract. The bacterial probe was assumed to contact the composite surface when the slope of the approach curves abruptly increases. Retraction of the bacterial probe from a composite surface with and without saliva was done after 0 s and 120 s surface delay in order to reveal possible bond-strengthening. Scanning electron micrographs were regularly taken to confirm the integrity of the bacterial probe after measurements. No force–distance curves had to be discarded due to visual damage to the bacterial probe in the study. For each combination of composite, roughness and bacterial strain, 30 force–distance curves were recorded that comprised a total of 6 different bacterial probes out of 3 independent bacterial cultures and 3 different composite discs.
Adhesion forces were calculated after each surface delay time from the AFM deflection data, using
in which K sp is the spring constant and D is the deflection of the cantilever. The spring constant of each cantilever was experimentally determined, using the thermal method .
2.5
Statistical analysis
Surface roughnesses ( R a ) were presented as averages ± standard deviations (SD) and analyzed using ANOVA. Bacterial adhesion forces were presented as medians and interquartile ranges, and compared using non-parametric analyses (Kruskal–Wallis test, followed by Dunns multiple-comparison post hoc analysis, when overall differences were significant). The level of significance was set at p < 0.05. Linear regression analysis was used to assess the relationship between the adhesion forces and R a values.
Typically, data for bacterial adhesion forces were not normally distributed and contained a large spread . Weibull analysis has been originally designed for statistical analysis of macroscopic tensile bond strength measurements, which also suffer from a large spread in data, but is equally applicable to microscopic adhesion forces measured using AFM . Weibull analysis uses spread in data to calculate the so-called Weibull distribution according to
where the P F is the adhesion force probability, F is the adhesion force measured, F 0 is a scaling parameter and m is the Weibull modulus. P F is obtained from a ranking of all measured adhesion forces in ascending order according to
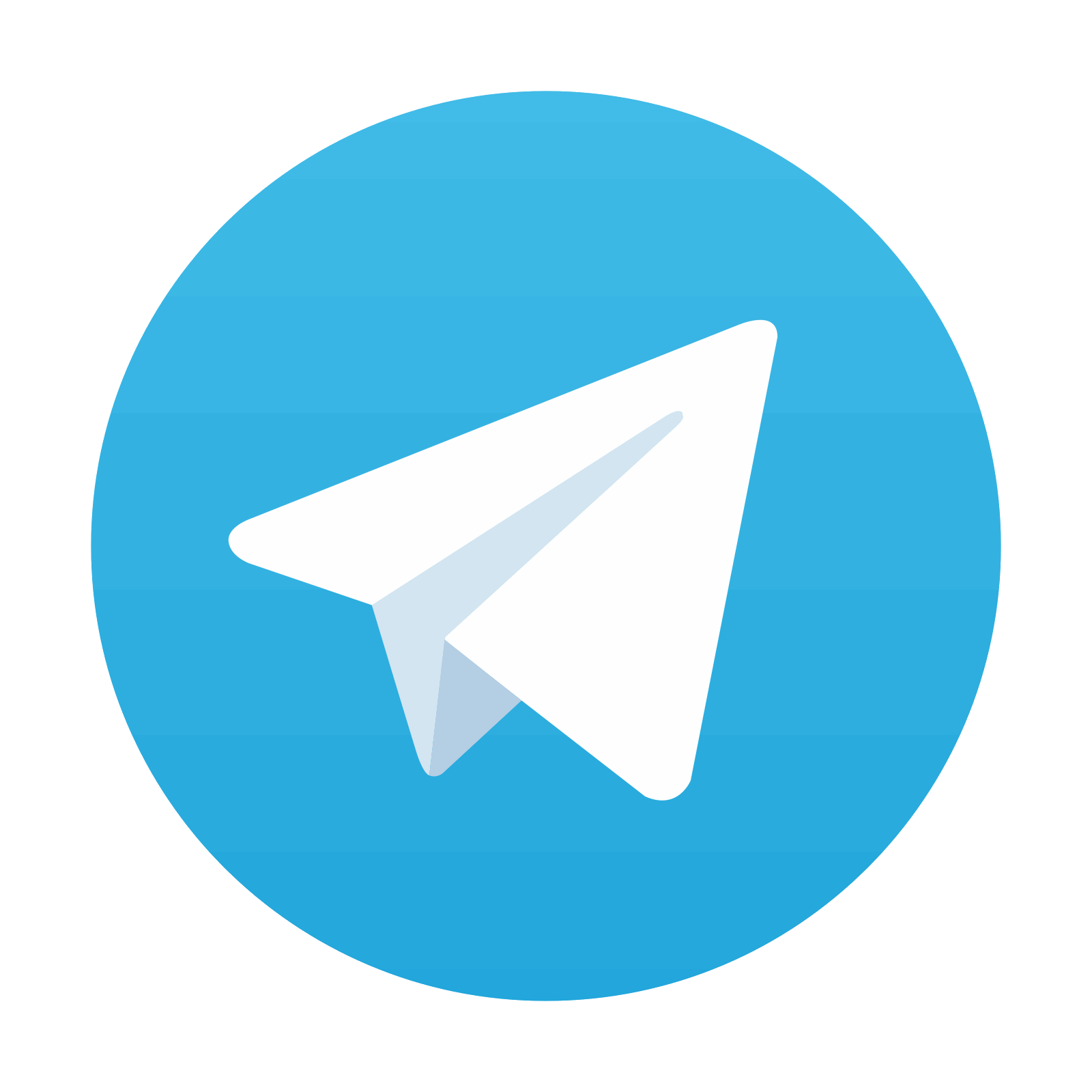
Stay updated, free dental videos. Join our Telegram channel

VIDEdental - Online dental courses
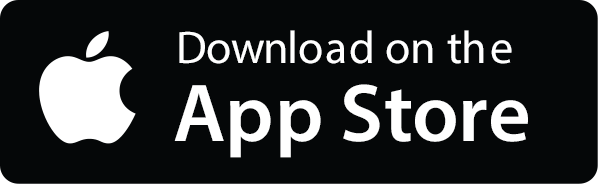
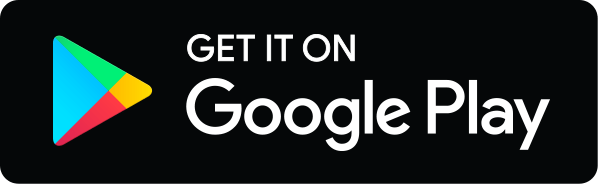