Highlights
- •
Full-coverage occlusal veneers of different thicknesses were tested.
- •
The lithium disilicate occlusal veneers were exposed to masticatory simulation.
- •
Only specimens with the thickest dimension fully survived the artificial ageing.
- •
Thickness of the occlual veneers had significant influence on fracture resistance.
- •
A thickness of 0.7–1 mm is suggested for occlusal lithium disilicate veneers.
Abstract
Objectives
The purpose of this in-vitro study was to evaluate the influence of ceramic thickness and type of dental bonding surface on the fracture resistance of non-retentive full-coverage adhesively retained occlusal veneers made from lithium disilicate ceramic.
Methods
Seventy-two extracted molars were divided into three test groups ( N = 24) depending on the location of the occlusal veneer preparation: solely within enamel, within enamel and dentin or within enamel and an occlusal composite resin filling. For each test group, occlusal all-ceramic restorations were fabricated from lithium disilicate ceramic blocks (IPS e.max CAD) in three subgroups with different thicknesses ranging from 0.3 to 0.7 mm in the fissures and from 0.6 to 1.0 mm at the cusps. The veneers were etched (5% HF), silanated and adhesively luted using a self etching primer and a composite luting resin (Multilink Primer A/B and Multilink Automix). After water storage at 37 °C for 3 days and thermal cycling for 7500 cycles at 5–55 °C, specimens were subjected to dynamic loading in a chewing simulator with 600,000 loading cycles at 10 kg combined with thermal cycling. Unfractured specimens were loaded until fracture using a universal testing machine. Statistical analysis was performed using Kruskal–Wallis and Wilcoxon tests with Bonferroni–Holm correction for multiple testing.
Results
Only specimens in the group with the thickest dimension (0.7 mm in fissure, 1.0 mm at cusp) survived cyclic loading without any damage. Survival rates in the remaining subgroups ranged from 50 to 100% for surviving with some damage and from 12.5 to 75% for surviving without any damage. Medians of final fracture resistance ranged from 610 to 3390 N. In groups with smaller ceramic thickness, luting to dentin or composite provided statistically significant ( p ≤ 0.05) higher fracture resistance than luting to enamel only. The thickness of the occlual ceramic veneers had a statistically significant ( p ≤ 0.05) influence on fracture resistance.
Significance
The results suggest to use a thickness of 0.7–1 mm for non-retentive full-coverage adhesively retained occlusal lithium disilicate ceramic restorations.
1
Introduction
The prosthodontic treatment of severly abraded teeth or patients with a lateral open bite after orthodontic treatment is an important aspect in dental therapy. Modern dental ceramics such as lithium disilicate nowadays offer the option of minimally invasive replacement of lost tooth substance while having a high fracture resistance . Failures of all-ceramic restorations are mainly based on fractures of the ceramic material .
Fracture resistance of all-ceramic restorations is influenced by different factors. The adhesive technique used plays an important role in this regard since adhesively bonded all-ceramic restorations show a higher fracture resistance than conventionally cemented restorations . Today resin cements based on the Bisphenol A glycidylmethacrylate compound are being used primarily. In order to bond to the surfaces of the different components (tooth structure as well as restoration) they need to be conditioned specifically; since each interface quality influences the fracture resistance of the restoration .
The type of bonding surface and the type of surface conditioning affects the bond strength of the ceramic to the tooth structure. Restorations bonded to teeth using the total etch technique achieved a bond strength of up to 28 MPa within the enamel and 13 to 20 MPa within the dentin (depending on the adhesive system used) . An improved bond strength to dentin can be reached when an immediate sealing of the dentin after the preparation is done . Today many manufacturers offer self-etching primers which are supposed to simplify the adhesive bonding procedure. Different bond strength values have been reported in the literature regarding these self-etching primers. The bond strength of ceramic bonded to enamel was about 26 MPa regardless of the manufacturer while the bond strength of ceramic bonded to dentin was 15 to 29 MPa depending on the adhesive system used . In these studies specimens were not subjected to thermal cycling, water storage or masticatory simulation though. For example a study of Zhang et al. reported a bond strength to dentin of up to 36 MPa using a self-etching primer.
A further factor influencing the fracture resistance of all-ceramic restorations is the design of the preparation. For all-ceramic restorations the preparation has to be rounded carefully and no sharp angles should exist . The thickness of the ceramic restoration is another factor influencing its fracture resistance . Scientific data on the minimum thickness of lithium disilicate ceramic partial crowns or occlusal veneers is rare. In vitro studies on bonded occlusal veneers made of emax CAD showed that restorations with a thickness of 1.2–1.8 mm withstood forces of up to 1000 N and thicknesses of 0.6–1.0 mm withstood 800 N . A study of Guess et al. investigated the influence of the thickness and the extension of different premolar partial crowns made of a pressed lithium disilicate ceramic. No significant effect on the fracture resistance of pressable lithium disilicate ceramic onlay restorations was found in this study when the preparation depth was reduced down to 0.5 mm.
The success of all-ceramic restorations is related to multiple factors. The purpose of this study was to evaluate the effect of varying thicknesses of non-retentive full-coverage adhesively retained occlusal lithium disilicate ceramic restorations (further on referred to as occlusal veneers) bonded to different surfaces using a bonding system based on a self-etching primer.
2
Materials and methods
2.1
Specimen fabrication
Seventy two human molars without any caries or fillings were cleaned and stored in a 0.1% thymol solution. They were embedded in a technique which proved to be effective in previous studies . The root portion apically of the cemento-enamel junction was coated with an artificial periodontal membrane made of gum resin (Anti-Rutsch-Lack, Wenko-Wenselaar, Hilden, Germany). The roots of the teeth were then embedded in custom made standard brass cylinders (Ø 15 mm) positioned along their long axis with auto-polymerizing acrylic resin material (Technovit 4000, Heraeus Kulzer, Wehrheim, Germany). The enamel-cement junction was located 2 mm above the level of the embedded resin. The roots were retained in the resin by a thin steel bar (Ø 0.9 mm) inserted in the apical third of the root.
Specimens were divided into three groups ( n = 24 each). In the first group the preparation was only within the enamel (group EN), in the second group the preparation was not limited to the enamel but extended into the dentin (group ED) and in the third group the preparation extended into the dentin also but the dentin core was reduced by 1.5 mm and a composite filling (Tetric EvoCeram, Ivoclar Vivadent, Schaan, Liechtenstein) was placed into the cavity (group EC) using a three-step bonding system (Optibond FL, Kerr, Charlotte, NC, USA). Finally all preparations and all composite fillings were smoothed and sharp edges were rounded. In all three groups the circumferential outline of the preparation was strictly within the enamel. An angle of 150 degrees was prepared between the cusps ( Fig. 1 ). After tooth preparation, impressions were made using a simultaneous dual-mix technique with polyether material (Permadyne Penta H und L, 3 M ESPE, Seefeld, Germany). The impressions were cast with die stone type 4 (Hydrobase300, Dentona, Dortmund, Germany). The teeth were stored in a 0.1% thymol solution until adhesive luting.

In order to achieve a constant ceramic thickness the occlusal surface received a semi-anatomic shaping. In the CAD/CAM software the occlusal surface of the tooth was virtually elevated and then reduced again in the fissure area until the desired thickness was obtained. The master casts were sent to a commercial milling center (Biodentis, Leipzig, Germany). The unsintered ceramic occlusal veneers were then milled out of lithium disilicate blocks (IPS e.max.CAD, Ivoclar Vivadent, Schaan, Liechtenstein) in accordance to the planned design using CAD/CAM technique. They were fitted to the master casts and sintered according to the manufacturers’ directions. Test groups are summarized in Table 1 .
Preparation (group code) | Ceramic thickness | ||
---|---|---|---|
0.3–0.6 mm ( n = 24) | 0.5–0.8 mm ( n = 24) | 0.7–1.0 mm ( n = 24) | |
Restricted to enamel (EN) ( n = 24) | EN1 ( n = 8) | EN2 ( n = 8) | EN3 ( n = 8) |
Enamel and dentin (ED) ( n = 24) | ED1 ( n = 8) | ED2 ( n = 8) | ED3 ( n = 8) |
Enamel and dentin with composite filling (EC) ( n = 24) | EC1 ( n = 8) | EC2 ( n = 8) | EC3 ( n = 8) |
2.2
Bonding procedure
A self-etching primer (Multilink Primer A and B, Ivoclar Vivadent, Schaan, Liechtenstein) was mixed for 10 s with a ratio of 1:1. The teeth were then pretreated according to the following protocol: in groups EN and EC the primer was applied onto the bonding surface of the tooth with a brush for 15 s and after another 15 s the surface was gently air dried. In group ED the primer was first applied to the enamel surface for 15 s before it was applied to the dentin surface for 15 s, followed by gentle air drying of the surface.
The bonding surfaces of the restorations were etched for 20 s using 5% hydrofluoric acid etching gel (IPS Ceramic Ätzgel, Ivoclar Vivadent). The etched ceramic surface was thoroughly cleaned using water spray for 60 s. After airdrying a silane coupling agent (Monobond Plus, Ivoclar Vivadent) was applied and air dried again after 60 s.
A self-curing luting composite (Multilink Automix, Ivoclar Vivadent) was dispensed from the automix syringe onto the bonding surface of the restoration and onto the fissure area of the tooth. The restoration was positioned by hand and kept in place by a special loading apparatus with a constant force of 50 N. Subsequently, all margins were light-cured for 20 s according to the manufacturer’s instructions.
After a curing period of 5 min the specimens were removed from the loading apparatus, the margins were polished and the specimens were stored in water for 3 days at 37 °C in order to achieve complete curing.
2.3
Cyclic loading and fracture load
After water storage all specimens were first thermocycled 7500 times between 5 and 55 °C in tap water with a 30 s dwell time at each temperature in a computerized masticatory simulator (Willytec, Munich, Germany), followed by combined thermocycling (60 s dwell time) and dynamic loading for another 600,000 cycles with a weight of 10 kg. A loading cycle frequency of 2.0 Hz with a lateral sliding component of 0.3 mm towards the central fissure was chosen to simulate conditions in the oral cavity. The descending velocity was 30 mm/s, the ascending velocity was 55 mm/s and the vertical motion was 6 mm. The antagonistic tooth was simulated by a steatite ceramic ball 5 mm in diameter (Hoechst Ceram Tec, Wunsiedel, Germany) which was positioned so that it first contacted the supporting cusp when moving down.
Following masticatory simulation all unfractured specimens were examined using a stereomicroscope (Wild, Heerbrugg, Switzerland) and they were photographed (Leica DC 100, Leica Microsystems, Cambridge, UK) in order to record possible damage.
The specimens were then loaded until fracture in a universal testing machine (Zwick Z010/TN2A, Ulm, Germany). A steel bar with a 6 mm ball end was centered on the main fissure of each specimen in order to apply the load evenly to the triangular ridges of the oral and buccal cusps. Additionally a 0.6 mm tin foil was placed between the ball end and the specimen in order to distribute the load homogenously. The steel bar descended at a cross-head speed of 2 mm/min while a computer software (testXpert II, Zwick, Ulm, Germany) recorded the maximum load until fracture in Newton.
After static loading all specimens underwent a stereomicroscopic evaluation again and were photographed. In addition sampled fragments of specimens that did not withstand dynamic loading as well as sampled fragments of specimens after static loading were evaluated using a scanning electron microscope (XL 30 CP, Philips, Eindhoven, Netherlands) in order to gain information on the failure mode of the adhesive bond. Some specimens which already showed damage after dynamic loading were also inspected using the scanning electron microscope.
2.4
Analysis of results and statistics
The Shapiro–Wilk test was performed on all groups and revealed, that some groups were not distributed normally. Hence the Kruskal–Wallis test was performed on all groups and revealed statistically significant differences. This test was followed by multi-pairwise comparisons of all groups using the Wilcoxon rank sum test and the risk of an alpha error was reduced by performing the procedure of Bonferroni–Holm on each resulting p -value.
2
Materials and methods
2.1
Specimen fabrication
Seventy two human molars without any caries or fillings were cleaned and stored in a 0.1% thymol solution. They were embedded in a technique which proved to be effective in previous studies . The root portion apically of the cemento-enamel junction was coated with an artificial periodontal membrane made of gum resin (Anti-Rutsch-Lack, Wenko-Wenselaar, Hilden, Germany). The roots of the teeth were then embedded in custom made standard brass cylinders (Ø 15 mm) positioned along their long axis with auto-polymerizing acrylic resin material (Technovit 4000, Heraeus Kulzer, Wehrheim, Germany). The enamel-cement junction was located 2 mm above the level of the embedded resin. The roots were retained in the resin by a thin steel bar (Ø 0.9 mm) inserted in the apical third of the root.
Specimens were divided into three groups ( n = 24 each). In the first group the preparation was only within the enamel (group EN), in the second group the preparation was not limited to the enamel but extended into the dentin (group ED) and in the third group the preparation extended into the dentin also but the dentin core was reduced by 1.5 mm and a composite filling (Tetric EvoCeram, Ivoclar Vivadent, Schaan, Liechtenstein) was placed into the cavity (group EC) using a three-step bonding system (Optibond FL, Kerr, Charlotte, NC, USA). Finally all preparations and all composite fillings were smoothed and sharp edges were rounded. In all three groups the circumferential outline of the preparation was strictly within the enamel. An angle of 150 degrees was prepared between the cusps ( Fig. 1 ). After tooth preparation, impressions were made using a simultaneous dual-mix technique with polyether material (Permadyne Penta H und L, 3 M ESPE, Seefeld, Germany). The impressions were cast with die stone type 4 (Hydrobase300, Dentona, Dortmund, Germany). The teeth were stored in a 0.1% thymol solution until adhesive luting.
In order to achieve a constant ceramic thickness the occlusal surface received a semi-anatomic shaping. In the CAD/CAM software the occlusal surface of the tooth was virtually elevated and then reduced again in the fissure area until the desired thickness was obtained. The master casts were sent to a commercial milling center (Biodentis, Leipzig, Germany). The unsintered ceramic occlusal veneers were then milled out of lithium disilicate blocks (IPS e.max.CAD, Ivoclar Vivadent, Schaan, Liechtenstein) in accordance to the planned design using CAD/CAM technique. They were fitted to the master casts and sintered according to the manufacturers’ directions. Test groups are summarized in Table 1 .
Preparation (group code) | Ceramic thickness | ||
---|---|---|---|
0.3–0.6 mm ( n = 24) | 0.5–0.8 mm ( n = 24) | 0.7–1.0 mm ( n = 24) | |
Restricted to enamel (EN) ( n = 24) | EN1 ( n = 8) | EN2 ( n = 8) | EN3 ( n = 8) |
Enamel and dentin (ED) ( n = 24) | ED1 ( n = 8) | ED2 ( n = 8) | ED3 ( n = 8) |
Enamel and dentin with composite filling (EC) ( n = 24) | EC1 ( n = 8) | EC2 ( n = 8) | EC3 ( n = 8) |
2.2
Bonding procedure
A self-etching primer (Multilink Primer A and B, Ivoclar Vivadent, Schaan, Liechtenstein) was mixed for 10 s with a ratio of 1:1. The teeth were then pretreated according to the following protocol: in groups EN and EC the primer was applied onto the bonding surface of the tooth with a brush for 15 s and after another 15 s the surface was gently air dried. In group ED the primer was first applied to the enamel surface for 15 s before it was applied to the dentin surface for 15 s, followed by gentle air drying of the surface.
The bonding surfaces of the restorations were etched for 20 s using 5% hydrofluoric acid etching gel (IPS Ceramic Ätzgel, Ivoclar Vivadent). The etched ceramic surface was thoroughly cleaned using water spray for 60 s. After airdrying a silane coupling agent (Monobond Plus, Ivoclar Vivadent) was applied and air dried again after 60 s.
A self-curing luting composite (Multilink Automix, Ivoclar Vivadent) was dispensed from the automix syringe onto the bonding surface of the restoration and onto the fissure area of the tooth. The restoration was positioned by hand and kept in place by a special loading apparatus with a constant force of 50 N. Subsequently, all margins were light-cured for 20 s according to the manufacturer’s instructions.
After a curing period of 5 min the specimens were removed from the loading apparatus, the margins were polished and the specimens were stored in water for 3 days at 37 °C in order to achieve complete curing.
2.3
Cyclic loading and fracture load
After water storage all specimens were first thermocycled 7500 times between 5 and 55 °C in tap water with a 30 s dwell time at each temperature in a computerized masticatory simulator (Willytec, Munich, Germany), followed by combined thermocycling (60 s dwell time) and dynamic loading for another 600,000 cycles with a weight of 10 kg. A loading cycle frequency of 2.0 Hz with a lateral sliding component of 0.3 mm towards the central fissure was chosen to simulate conditions in the oral cavity. The descending velocity was 30 mm/s, the ascending velocity was 55 mm/s and the vertical motion was 6 mm. The antagonistic tooth was simulated by a steatite ceramic ball 5 mm in diameter (Hoechst Ceram Tec, Wunsiedel, Germany) which was positioned so that it first contacted the supporting cusp when moving down.
Following masticatory simulation all unfractured specimens were examined using a stereomicroscope (Wild, Heerbrugg, Switzerland) and they were photographed (Leica DC 100, Leica Microsystems, Cambridge, UK) in order to record possible damage.
The specimens were then loaded until fracture in a universal testing machine (Zwick Z010/TN2A, Ulm, Germany). A steel bar with a 6 mm ball end was centered on the main fissure of each specimen in order to apply the load evenly to the triangular ridges of the oral and buccal cusps. Additionally a 0.6 mm tin foil was placed between the ball end and the specimen in order to distribute the load homogenously. The steel bar descended at a cross-head speed of 2 mm/min while a computer software (testXpert II, Zwick, Ulm, Germany) recorded the maximum load until fracture in Newton.
After static loading all specimens underwent a stereomicroscopic evaluation again and were photographed. In addition sampled fragments of specimens that did not withstand dynamic loading as well as sampled fragments of specimens after static loading were evaluated using a scanning electron microscope (XL 30 CP, Philips, Eindhoven, Netherlands) in order to gain information on the failure mode of the adhesive bond. Some specimens which already showed damage after dynamic loading were also inspected using the scanning electron microscope.
2.4
Analysis of results and statistics
The Shapiro–Wilk test was performed on all groups and revealed, that some groups were not distributed normally. Hence the Kruskal–Wallis test was performed on all groups and revealed statistically significant differences. This test was followed by multi-pairwise comparisons of all groups using the Wilcoxon rank sum test and the risk of an alpha error was reduced by performing the procedure of Bonferroni–Holm on each resulting p -value.
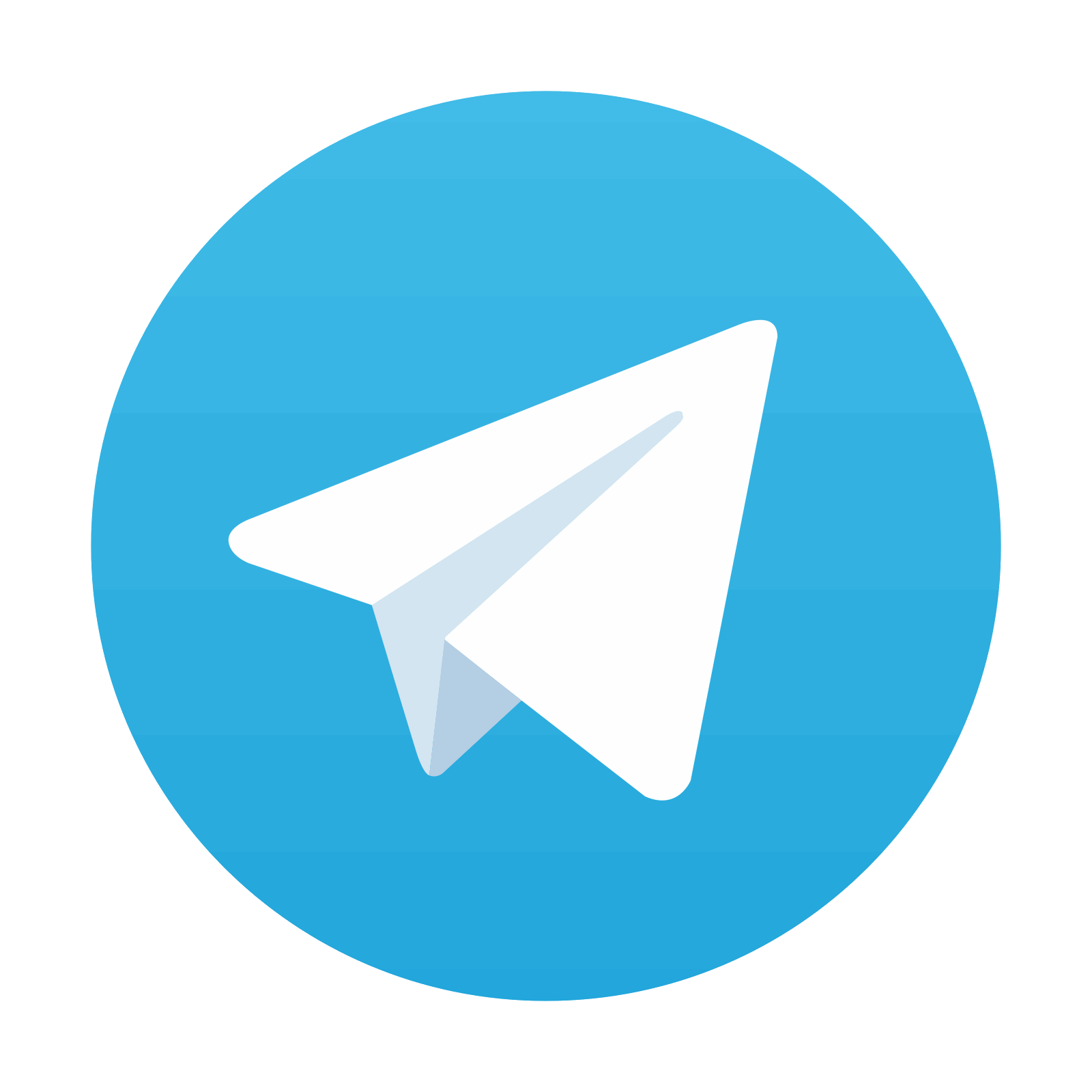
Stay updated, free dental videos. Join our Telegram channel

VIDEdental - Online dental courses
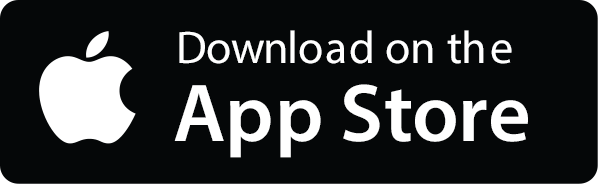
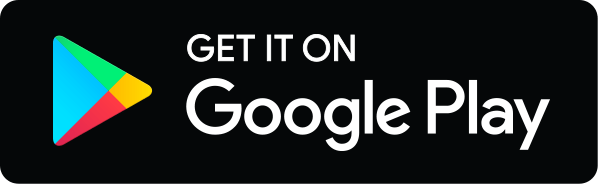