Graphical abstract
Highlights
- •
The ion-releasing resin induces mineral precipitation at the resin–dentine–dentin interface.
- •
Biomimetic analogues phosphorylate acid-etched dentin and remineralize the hybrid layer.
- •
Remineralization through biomimetic primers increases the elastic modulus of hybrid layers.
- •
The micropermeability of hybrid layers reduces considerably after biomimetic remineralization.
- •
The biomimetic remineralization increases the durability of resin–dentin bonds.
Abstract
Objective
The study aimed at evaluating the remineralization of acid-etched dentin pre-treated with primers containing biomimetic analogs and bonded using an ion-releasing light-curable resin-based material.
Methods
An experimental etch-and-rinse adhesive system filled with Ca 2+ , PO 4 3− -releasing Ca-Silicate micro-fillers was created along with two experimental primers containing biomimetic analogs such as sodium trimetaphosphate (TMP) and/or polyaspartic acid (PLA). Dentin specimens etched with 37% H 3 PO 4 were pre-treated with two different aqueous primers containing the polyanionic biomimetic analogs or deionized water and subsequently bonded using the experimental resin-based materials. The specimens were sectioned and analyzed by AFM/nanoindentation to evaluate changes in the modulus of elasticity (Ei) across the resin–dentin interface at different AS storage periods (up to 90 days). Raman cluster analysis was also performed to evaluate the chemical changes along the interface. The phosphate uptake by the acid-etched dentin was evaluated using the ATR-FTIR. Additional resin–dentin specimens were tested for microtensile bond strength. SEM examination was performed after de-bonding, while confocal laser microscopy was used to evaluate the interfaces ultramorphology and micropermeability.
Results
Both biomimetic primers induced phosphate uptake by acid-etched dentin. Specimens created with the ion-releasing resin in combination with the pre-treatment primers containing either PLA and TMA showed the greatest recovery of the Ei of the hybrid layer, with no decrease in μTBS ( p > 0.05) after 3-month AS storage. The ion-releasing resin applied after use of the biomimetic primers showed the greatest reduction in micropermeability due to mineral precipitation; these results were confirmed using SEM.
Significance
The use of the ion-releasing resin-based system applied to acid-etched dentin pre-treated with biomimetic primers containing analogs of phosphoproteins such as poly- l -aspartic acid and/or sodium trimetaphosphate provides a suitable bonding approach for biomimetic remineralization of resin–dentin interfaces.
1
Introduction
Dental caries represents the major reason for placement and replacement of restorations . The concept of minimally invasive (MI) was applied for the treatment of deeper carious lesions by adopting the atraumatic restorative treatment (ART) approach (1990s) with the aim of selective caries removal to excavate infected carious tissue leaving as much caries-affected dentin as possible for therapeutic remineralization .
Contemporary ART concepts coupled with the advent of the latest generation of adhesive systems have radically influenced modern operative management of dental caries . The application of resin-based materials on demineralized dentin (e.g. acid-etched dentin) followed by placement of esthetic light-curing resin composites represents a revolution in adhesive dentistry . Acid-etching is the first step in resin–dentin bonding. Using 32–37% phosphoric acid in etch-and-rinse adhesive systems, this step removes smear layers and completely demineralizes dentin to a depth of 5–8 μm. That step also uncovers and activates all preforms of the endogenous proteases of dentin matrices metalloproteinases (MMPs) and cathepsin . As the mineral phase of dentin is 50 vol.% mineral, complete demineralization replaces all that minerals with 50 vol.% of water. Because the proteases are hydrolases, that is, they hydrolyze peptide bonds by enzymatically adding water across specific peptide bonds, it is important that resins replace all of that water, so that the proteases cannot degrade collagen.
Unfortunately, resin infiltration of water-saturated dentin matrices is not uniform, in particular, in thick layers of caries-affected dentin. Indeed, some areas of hybrid layers are well infiltrated and exhibit little residual water, while adjacent regions are not properly infiltrated and may contain very little resin, but 30–40% residual water . It is thought that the resin-sparse, water-rich zones in resin-bonded interfaces degrade over 1–2 years and their stiffness is so low that they may undergo excessive cyclic strain under normal function and fatigue failure .
Clinicians only have one chance for resin infiltration. If normal resin–dentin bonds include 30–50% of their volume filled with water instead of resin, how can these water-filled defects be corrected? One answer to that problem is to displace the residual water by filling the voids with nano-sized crystals of apatite. Remineralization is a form of dehydration . By removing residual water by remineralization, the activated MMPs in the matrix can be inactivated .
It has been recently proposed that all resin bonds be covered with a flowable resin composite containing amorphous calcium phosphate (ACP)/calcium silicates/and biomimetic polyanions that can slowly diffuse through any water-filled channels (e.g. water trees in adhesives and hybrid layers, residual water in un-infiltrated dentin) and “back-fill” any such defects with apatite-like mineral crystals, displacing all residual water and inactivating all proteases by mineralizing the enzymes; this approach was shown to increase the durability of such resin–dentin bonds .
One more critical problem associated with such esthetic restorations is the absence of therapeutic remineralization of caries-affected dentin and the poor durability/integrity of the resin–dentin interface during aging . ART is considered to have a combined technique–material effect. It requires removal of the caries-infected dental tissues in order to arrest the caries progression (Massler’s theory) and induces dental remineralization while utilizing the healing potential of glass ionomer cements (GICs) .
Unfortunately, Yiu et al. demonstrated that, even though specific GICs developed for the ART treatments of carious dentin may favor the penetration of particular ions deep into caries-affected dentin , they fail to remineralize apatite-depleted dentin due to a lack of nucleation of new apatite. This lack of remineralization has also been confirmed by Kim et al. who reported the failure of GICs to remineralize apatite-depleted dentin, even in the presence of biomimetic remineralizing analogs. Also in this case, the application of biomimetic analogs as therapeutic remineralizing strategies has been advocated as a possible method to achieve a more reliable approach to restore the elastic modulus values due to the creation of apatite crystallites similar to those of sound dentin . The advent of these new therapeutic concepts in minimally invasive restorative/adhesive dentistry has captured the attention of many researchers so far, showing encouraging results both in terms of healing properties (i.e. remineralization) and longevity of the bonding interface . Nevertheless, it is still necessary to generate bioactive/biomimetic strategies with enhanced therapeutic remineralization properties at the hybrid layer.
Thus, the present study aimed at evaluating the remineralizing of water-saturated collagen that remains uninfiltrated with resin when using an experimental light-curable resin-based etch-and-rinse dental adhesive containing ion-releasing micro-fillers applied on acid-etched dentin pre-treated with one of the different biomimetic primers containing either sodium trimetaphosphate or polyaspartic acid as a nano-precursor cluster stabilizer . The null hypothesis to be tested was that the pre-treatment of acid-etched dentin with biomimetic primers does not improve the remineralization ability of an experimental light-curable resin-based system containing ion-releasing micro-fillers.
2
Materials and methods
2.1
Preparation of the experimental resin and biomimetic primers
A control co-monomer blend (Res.CTR) was formulated using urethane dimethacrylate (UDMA: 30 wt%), ethoxylated bisphenol-A dimethacrylate (Bis-GMA: 5 wt%), triethyleneglycol dimethacrylate (TEGDMA: 25 wt%) (Esstech, Essington, PA), hydroxyethyl methacrylate (HEMA: 18 wt%), absolute ethanol (15 wt%), camphorquinone (CQ: 1.0 wt%) and ethyl-4-dimethylaminobenzoate (EDAB: 1.0 wt%) (Sigma-Aldrich, St. Louis, MO). A control primer (Pr.CTR) was also created by solvating Res.CTR (50 wt%) with absolute ethanol (50 wt%). A type I Portland cement (Italcementi Group, Italy) (80 wt%) was mixed with 20 wt% β-TCP (Sigma–Aldrich) and deionized water (ratio 2:1). After setting (24 h), the cement was incubated at 40 ̊C for 12 h, milled, sieved to <30 μm and incorporated as a filler into the unfilled resin control (filler/resin ratio: 40/60 wt%) to create the experimental ion-releasing resin (Res.IR) .
The first biomimetic primer solution was created by dissolving 150 μg/mL of poly- l -aspartic acid (PLA) with a molecular weight of 27 kDa (Sigma-Aldrich) as previously described . A second biomimetic primer solution (TMP + PLA) was created by dissolving 10 wt% sodium trimetaphosphate (TMP; Mw 367.8, Sigma-Aldrich), an analog of dentin matrix proteins, and 150 μg/mL of PLA in deionized water; the pH of both solutions was adjusted to 7.1 . This experiment was designed to have the presence of such chemical species released by the bioactive ion-releasing filler and from the artificial saliva solution (AS) used in this study. The composition of artificial saliva was 0.7 mM/L CaCl 2 , 0.2 mM/L MgCl 2 , 4.0 mM/L KH 2 PO 4 , 30.0 mM/L KCl, 20.0 mM/L HEPES .
2.2
ATR-FTIR analysis of dentin collagen
Six sound human third molars were obtained with informed consent from donors and used in accordance with the ethical guidelines of the Research Ethics Committee (REC) for medical investigations. The teeth were stored at 4 °C in 0.5% chloramine-T up to 30 days before use. Two middle-dentin specimens (2 × 2 × 1.5 mm) were obtained from each tooth using a hard tissue microtome (Accutom-50; Struers, Copenhagen, Denmark) equipped with a slow-speed water-cooled diamond wafering saw (330-CARS-70300, Struers, Copenhagen, Denmark). A 320-grit silicon carbide (SiC) abrasive paper mounted on a water-cooled polishing machine (LaboPol-4, Struers, Copenhagen, Denmark) was used to produce a clinically relevant smear layer on both surfaces of the specimens. The dentin specimens were immersed in a 37% H 3 PO 4 solution and etched for 15 s; they were subsequently rinsed with distilled water (5 s). These specimens (no. sp. =2/each group) were used to evaluate the chemical changes (phosphate uptake) induced by the two experimental biomimetic primers compared to the single control primer (no biomimetic agents) when applied onto demineralized dentin for 1 min and subsequently rinsed with distilled water (5 s); etched-dentin specimens pre-treated with deionized water were also analyzed. Three different positions of each specimen were analyzed using the ATR-FTIR Spectrometer (Spectrum One, Perkin-Elmer, UK) in the region of 650–4000 cm −1 with a resolution of 4 cm −1 and 64 scans per spectrum. A moderate pressure was applied (5 psi) to establish good contact between the specimen and the ATR surface .
2.3
Dentin specimen preparation and bonding procedures
Thirty smear-layer covered middle-dentin specimens obtained from additional sound human third molars were prepared as previously described. After acid-etching (37% H 3 PO 4 ; 15 s), the specimens were pre-treated by immersion in deionized water (DW) (no. sp. = 10) or were immersed in one of the two biomimetic primer solutions tested in this study: (i) PLA ( n = 10); (ii) TMP + PLA ( n = 10). Subsequently, these specimens were washed with DW (5 s) air-dried (3 s) and immediately primed using two consecutive layers of the adhesive control primer Pr.CTR. Two consecutive layers of the filler-free Res.CTR (no. sp. = 5) or ion-releasing resin (Res.IR) (no. sp. = 5) were applied and light-cured for 30 s using a Translux EC halogen light-curing unit (KulzerGmbH, BereichDental, Wehrheim, Germany) at a distance of 1 mm. The bonding procedures were completed by applying the resin composite (TetricEvo Cerams, Ivoclar Vivadent, Schaan, Liechtenstein) with five 1 mm layer increments and light-cured for 40 s. Further details about the bonding procedures are depicted in Fig. 1 .
2.4
AFM imaging and nano-indentation
The resin-bonded dentin specimens prepared for each group were longitudinally sectioned into multiple 1.5-mm-thick slabs (three each specimen) and polished through ascending SiC papers from #800 up to #4000-grit. Further final polishing procedure was performed using diamond pastes (Buheler-MetaDi, Buheler Ltd., Lake Bluff, IL, USA) through 1 μm down to 0.25 μm. The specimens were cleaned in ultrasonic bath (Model QS3, Ultrawave Ltd, Cardiff, UK) containing deionized water [pH 7.4] for 5 min at each polishing step.
An atomic force microscope (AFM-Nanoscope V, Digital Instruments, Veeco Metrology group, Santa Barbara, CA, USA) equipped with a Triboscope indentor system (Hysitron Inc., Minneapolis, MN) and a Berkovich indenter (tip radius ∼20 nm) was employed for the imaging and nano-indentation processes in a fully hydrated status as recently described by Sauro et al. . Briefly, five indentations with a load of 4000 nN and a time function of 10 s were performed after different AS storage periods (24 h, 30 days, 90 days) in a straight line starting from the top of hybrid layer down to the underlying intertubular dentin in order to evaluate changes in the modulus of elasticity (Ei) of hybrid layers. Three indentation lines 15 (±5) μm from each other were made in five different mesio-distal positions along the interface. The apical-occlusal distance between each indentation was kept constant (5 ± 1 μm) by adjusting the distance intervals steps ( Fig. 1 ) and the load function .
A Kolmogorov–Smirnov test was conducted to assess data distribution ( p > 0.05) and data were analyzed by ANOVA including analysis of interactions ( p < 0.05). Bonding procedure and storage period in AS were considered as independent variables. Student’s t -test ( p < 0.001) and Student–Newman–Keuls ( p < 0.05) were used for post hoc comparisons.
2.5
Dye-assisted confocal laser scanning microscopy evaluation (micropermeability analysis)
Three acid-etched dentin specimens for each group were bonded as previously described with the tested resin-based systems mixed with 0.2 wt% Rhodamine B (Sigma–Aldrich). After 24 h, all the specimens had their pulp chambers (PCs) exposed and immediately filled with 0.1 wt% sodium fluorescein solution (Sigma–Aldrich) for 3 h. The PCs of the other half part of specimens were immersed in AS for 90 days and only subsequently the PCs were filled with the fluorescein solution; the AS was replaced every 72 h .
All the specimens immersed in fluorescein were treated in an ultrasonic bath for 2 min, sectioned into slabs (1.5 mm) and finally polished using a 1200-grit SiC paper for 1 min. A confocal laser scanning microscope (DM-IRE2 CLSM; Leica, Heidelberg, Germany) equipped with a 63×/1.4 NA oil immersion lens and a 488-nm argon/krypton (fluorescein excitation) or 568-nm helium/neon ions laser (rhodamine B excitation) was used to analyze the ultra-morphology and micropermeability along the bonded-dentin interfaces; the emission fluorescence was recorded at 512–538 and 585–650 nm, respectively. Reflection and fluorescence optical images were captured 5 μm below the outer surface up to 25 μm depth (1 μm z-step) and converted into topographic and/or single projections using the Leica SP2 CLSM image-processing software (Leica, Heidelberg, Germany) . The configuration of the system was standardized and used at the same level throughout the entire investigation.
2.6
Qualitative Raman spectroscopy and quantitative cluster analysis
The same specimens submitted to AFM imaging/nano-indentation were also analyzed using a dispersive Raman spectrometer/microscope (Horiba Scientific Xplora, Villeneuve d’Ascq, France) after 24 h and 90 days of AS storage ( Fig. 1 ). A 785-nm diode laser (100 mW sample power) equipped with a ×100/0.90 NA water-immersion objective was employed. Raman signals were acquired using a 600-lines/mm grating centered between 900 and 1700 cm −1 . Three chemical mappings were captured along the interfaces (two in proximity of the pulpal horns and one in the middle of the pulpal floor) and submitted to K-means cluster (KMC) analysis as described by Toledano et al. , using multivariate analysis (ISyss Horiba), which includes statistical patterning to derive independent clusters; the biochemical content of each cluster was analyzed using the average cluster spectra. Principal component analysis (PCA) decomposed data were set into a bilinear model of linear independent variables, the so-called principal components (PCS). Five to six clusters were identified but only three representative clusters, always present in all the specimens before and after AS storage, were considered and values for each cluster such as demineralized dentin, sound dentin and resin-collagen hybrid zone within the interface were independently obtained. At this point, the mineral matrix ratio (MMR: the ratio of integrated areas of the phosphate P–O symmetric stretch [ v 1 —961 cm −1 ] and contour of the CH 2 deformation of the collagen [1465 cm −1 ]) was measured for the same cluster in each group, in order to quantitatively calculate the extent of mineralization along the interface. Data were analyzed by ANOVA and Tukey’s post hoc test for pairwise comparisons ( p < 0.05).
2.7
Micro-tensile bond strength (μTBS) and fracture ultra-morphology analysis (FEG-SEM)
A further five resin-bonded dentin specimens were prepared for each group and sectioned in both X and Y directions to obtain multiple bonded-sticks of 0.9 mm 2 . Their microtensile bond strengths were tested after 24 h or 90 days of AS storage using a customized microtensile jig on a linear actuator (SMAC Europe Ltd., Horsham, West Sussex, UK). Mean bond strength values were analyzed by ANOVA, which was performed including the bond strength (MPa) as the dependent variable. Bonding groups (CTR, PLA and TMP + PLA) and period of AS storage (24 h and 90 days) were considered as independent variables. Analysis of interactions was also conducted. Student–Newman–Keuls test was used for multiple comparisons. Statistical analysis was set at a significance level of α = 0.05. Modes of failure were classified as percentage of adhesive (A) or mixed (M) or cohesive (C) failures using a stereo microscope (magnification ×60). Three representative fractured specimens for each group were mounted on aluminum stubs using carbon tape, gold-sputter-coated and finally imaged using FEG-SEM (Gemini, Carl Zeiss, Oberkochen, Germany) at 3 kV and a working distance of 15 mm.
2
Materials and methods
2.1
Preparation of the experimental resin and biomimetic primers
A control co-monomer blend (Res.CTR) was formulated using urethane dimethacrylate (UDMA: 30 wt%), ethoxylated bisphenol-A dimethacrylate (Bis-GMA: 5 wt%), triethyleneglycol dimethacrylate (TEGDMA: 25 wt%) (Esstech, Essington, PA), hydroxyethyl methacrylate (HEMA: 18 wt%), absolute ethanol (15 wt%), camphorquinone (CQ: 1.0 wt%) and ethyl-4-dimethylaminobenzoate (EDAB: 1.0 wt%) (Sigma-Aldrich, St. Louis, MO). A control primer (Pr.CTR) was also created by solvating Res.CTR (50 wt%) with absolute ethanol (50 wt%). A type I Portland cement (Italcementi Group, Italy) (80 wt%) was mixed with 20 wt% β-TCP (Sigma–Aldrich) and deionized water (ratio 2:1). After setting (24 h), the cement was incubated at 40 ̊C for 12 h, milled, sieved to <30 μm and incorporated as a filler into the unfilled resin control (filler/resin ratio: 40/60 wt%) to create the experimental ion-releasing resin (Res.IR) .
The first biomimetic primer solution was created by dissolving 150 μg/mL of poly- l -aspartic acid (PLA) with a molecular weight of 27 kDa (Sigma-Aldrich) as previously described . A second biomimetic primer solution (TMP + PLA) was created by dissolving 10 wt% sodium trimetaphosphate (TMP; Mw 367.8, Sigma-Aldrich), an analog of dentin matrix proteins, and 150 μg/mL of PLA in deionized water; the pH of both solutions was adjusted to 7.1 . This experiment was designed to have the presence of such chemical species released by the bioactive ion-releasing filler and from the artificial saliva solution (AS) used in this study. The composition of artificial saliva was 0.7 mM/L CaCl 2 , 0.2 mM/L MgCl 2 , 4.0 mM/L KH 2 PO 4 , 30.0 mM/L KCl, 20.0 mM/L HEPES .
2.2
ATR-FTIR analysis of dentin collagen
Six sound human third molars were obtained with informed consent from donors and used in accordance with the ethical guidelines of the Research Ethics Committee (REC) for medical investigations. The teeth were stored at 4 °C in 0.5% chloramine-T up to 30 days before use. Two middle-dentin specimens (2 × 2 × 1.5 mm) were obtained from each tooth using a hard tissue microtome (Accutom-50; Struers, Copenhagen, Denmark) equipped with a slow-speed water-cooled diamond wafering saw (330-CARS-70300, Struers, Copenhagen, Denmark). A 320-grit silicon carbide (SiC) abrasive paper mounted on a water-cooled polishing machine (LaboPol-4, Struers, Copenhagen, Denmark) was used to produce a clinically relevant smear layer on both surfaces of the specimens. The dentin specimens were immersed in a 37% H 3 PO 4 solution and etched for 15 s; they were subsequently rinsed with distilled water (5 s). These specimens (no. sp. =2/each group) were used to evaluate the chemical changes (phosphate uptake) induced by the two experimental biomimetic primers compared to the single control primer (no biomimetic agents) when applied onto demineralized dentin for 1 min and subsequently rinsed with distilled water (5 s); etched-dentin specimens pre-treated with deionized water were also analyzed. Three different positions of each specimen were analyzed using the ATR-FTIR Spectrometer (Spectrum One, Perkin-Elmer, UK) in the region of 650–4000 cm −1 with a resolution of 4 cm −1 and 64 scans per spectrum. A moderate pressure was applied (5 psi) to establish good contact between the specimen and the ATR surface .
2.3
Dentin specimen preparation and bonding procedures
Thirty smear-layer covered middle-dentin specimens obtained from additional sound human third molars were prepared as previously described. After acid-etching (37% H 3 PO 4 ; 15 s), the specimens were pre-treated by immersion in deionized water (DW) (no. sp. = 10) or were immersed in one of the two biomimetic primer solutions tested in this study: (i) PLA ( n = 10); (ii) TMP + PLA ( n = 10). Subsequently, these specimens were washed with DW (5 s) air-dried (3 s) and immediately primed using two consecutive layers of the adhesive control primer Pr.CTR. Two consecutive layers of the filler-free Res.CTR (no. sp. = 5) or ion-releasing resin (Res.IR) (no. sp. = 5) were applied and light-cured for 30 s using a Translux EC halogen light-curing unit (KulzerGmbH, BereichDental, Wehrheim, Germany) at a distance of 1 mm. The bonding procedures were completed by applying the resin composite (TetricEvo Cerams, Ivoclar Vivadent, Schaan, Liechtenstein) with five 1 mm layer increments and light-cured for 40 s. Further details about the bonding procedures are depicted in Fig. 1 .
2.4
AFM imaging and nano-indentation
The resin-bonded dentin specimens prepared for each group were longitudinally sectioned into multiple 1.5-mm-thick slabs (three each specimen) and polished through ascending SiC papers from #800 up to #4000-grit. Further final polishing procedure was performed using diamond pastes (Buheler-MetaDi, Buheler Ltd., Lake Bluff, IL, USA) through 1 μm down to 0.25 μm. The specimens were cleaned in ultrasonic bath (Model QS3, Ultrawave Ltd, Cardiff, UK) containing deionized water [pH 7.4] for 5 min at each polishing step.
An atomic force microscope (AFM-Nanoscope V, Digital Instruments, Veeco Metrology group, Santa Barbara, CA, USA) equipped with a Triboscope indentor system (Hysitron Inc., Minneapolis, MN) and a Berkovich indenter (tip radius ∼20 nm) was employed for the imaging and nano-indentation processes in a fully hydrated status as recently described by Sauro et al. . Briefly, five indentations with a load of 4000 nN and a time function of 10 s were performed after different AS storage periods (24 h, 30 days, 90 days) in a straight line starting from the top of hybrid layer down to the underlying intertubular dentin in order to evaluate changes in the modulus of elasticity (Ei) of hybrid layers. Three indentation lines 15 (±5) μm from each other were made in five different mesio-distal positions along the interface. The apical-occlusal distance between each indentation was kept constant (5 ± 1 μm) by adjusting the distance intervals steps ( Fig. 1 ) and the load function .
A Kolmogorov–Smirnov test was conducted to assess data distribution ( p > 0.05) and data were analyzed by ANOVA including analysis of interactions ( p < 0.05). Bonding procedure and storage period in AS were considered as independent variables. Student’s t -test ( p < 0.001) and Student–Newman–Keuls ( p < 0.05) were used for post hoc comparisons.
2.5
Dye-assisted confocal laser scanning microscopy evaluation (micropermeability analysis)
Three acid-etched dentin specimens for each group were bonded as previously described with the tested resin-based systems mixed with 0.2 wt% Rhodamine B (Sigma–Aldrich). After 24 h, all the specimens had their pulp chambers (PCs) exposed and immediately filled with 0.1 wt% sodium fluorescein solution (Sigma–Aldrich) for 3 h. The PCs of the other half part of specimens were immersed in AS for 90 days and only subsequently the PCs were filled with the fluorescein solution; the AS was replaced every 72 h .
All the specimens immersed in fluorescein were treated in an ultrasonic bath for 2 min, sectioned into slabs (1.5 mm) and finally polished using a 1200-grit SiC paper for 1 min. A confocal laser scanning microscope (DM-IRE2 CLSM; Leica, Heidelberg, Germany) equipped with a 63×/1.4 NA oil immersion lens and a 488-nm argon/krypton (fluorescein excitation) or 568-nm helium/neon ions laser (rhodamine B excitation) was used to analyze the ultra-morphology and micropermeability along the bonded-dentin interfaces; the emission fluorescence was recorded at 512–538 and 585–650 nm, respectively. Reflection and fluorescence optical images were captured 5 μm below the outer surface up to 25 μm depth (1 μm z-step) and converted into topographic and/or single projections using the Leica SP2 CLSM image-processing software (Leica, Heidelberg, Germany) . The configuration of the system was standardized and used at the same level throughout the entire investigation.
2.6
Qualitative Raman spectroscopy and quantitative cluster analysis
The same specimens submitted to AFM imaging/nano-indentation were also analyzed using a dispersive Raman spectrometer/microscope (Horiba Scientific Xplora, Villeneuve d’Ascq, France) after 24 h and 90 days of AS storage ( Fig. 1 ). A 785-nm diode laser (100 mW sample power) equipped with a ×100/0.90 NA water-immersion objective was employed. Raman signals were acquired using a 600-lines/mm grating centered between 900 and 1700 cm −1 . Three chemical mappings were captured along the interfaces (two in proximity of the pulpal horns and one in the middle of the pulpal floor) and submitted to K-means cluster (KMC) analysis as described by Toledano et al. , using multivariate analysis (ISyss Horiba), which includes statistical patterning to derive independent clusters; the biochemical content of each cluster was analyzed using the average cluster spectra. Principal component analysis (PCA) decomposed data were set into a bilinear model of linear independent variables, the so-called principal components (PCS). Five to six clusters were identified but only three representative clusters, always present in all the specimens before and after AS storage, were considered and values for each cluster such as demineralized dentin, sound dentin and resin-collagen hybrid zone within the interface were independently obtained. At this point, the mineral matrix ratio (MMR: the ratio of integrated areas of the phosphate P–O symmetric stretch [ v 1 —961 cm −1 ] and contour of the CH 2 deformation of the collagen [1465 cm −1 ]) was measured for the same cluster in each group, in order to quantitatively calculate the extent of mineralization along the interface. Data were analyzed by ANOVA and Tukey’s post hoc test for pairwise comparisons ( p < 0.05).
2.7
Micro-tensile bond strength (μTBS) and fracture ultra-morphology analysis (FEG-SEM)
A further five resin-bonded dentin specimens were prepared for each group and sectioned in both X and Y directions to obtain multiple bonded-sticks of 0.9 mm 2 . Their microtensile bond strengths were tested after 24 h or 90 days of AS storage using a customized microtensile jig on a linear actuator (SMAC Europe Ltd., Horsham, West Sussex, UK). Mean bond strength values were analyzed by ANOVA, which was performed including the bond strength (MPa) as the dependent variable. Bonding groups (CTR, PLA and TMP + PLA) and period of AS storage (24 h and 90 days) were considered as independent variables. Analysis of interactions was also conducted. Student–Newman–Keuls test was used for multiple comparisons. Statistical analysis was set at a significance level of α = 0.05. Modes of failure were classified as percentage of adhesive (A) or mixed (M) or cohesive (C) failures using a stereo microscope (magnification ×60). Three representative fractured specimens for each group were mounted on aluminum stubs using carbon tape, gold-sputter-coated and finally imaged using FEG-SEM (Gemini, Carl Zeiss, Oberkochen, Germany) at 3 kV and a working distance of 15 mm.
3
Results
3.1
ATR-FTIR analysis of dentin collagen
Dentin before acid-etching presented phosphate bands at 885–1180 cm −1 (phosphate stretching mode) representative of mineral components, amide bands from organic components (1200–1725 cm −1 ) and O–H stretching of water (H 2 O) at 3200–3400 cm −1 . When dentin was acid-etched, a clear presence of collagen at 1650 and 1540 cm −1 (C O—amide I and CNH—amide II, respectively) as well as very low-intensity peaks at 1083 and 1034 cm −1 identified the loss of the P–OR esters ( Fig. 2 ). The application of the biomimetic primers PLA or TMP + PLA biomimetic solutions increased the phosphate peaks at 1083 and 1034 cm −1 and induced the formation a further PO stretching peak at 967 cm −1 ( v 3 —asymmetric stretching mode of hydroxyapatite) . The vibrational stretching of the CO C alcohol group (1048 cm −1 ) was also evident after biomimetic primer treatments, identifying the presence of HEMA (resin primer) which also showed the C O C peak at 1090 cm −1 .
3.2
AFM Nano-indentation and dye-assisted CLSM imaging
The Young’s modulus of elasticity (Ei) of the hybrid layer in the second (HL) and the third (BHL) indentations were influenced by the main effects: ( p < 0.001) bonding procedure, ( p < 0.001) storage period. Interactions between factors were also significant ( p < 0.001) ( Table 1 ).
The first indentation performed along each resin–dentin interface was localized on the top part of the hybrid layer ( Fig. 3C and D ). There was no significant difference ( p > 0.05) between the Ei values of each bonding procedure at the same indentation position (1st hybrid layer). Moreover, the aging periods (30 and 90 days) induced no significant change ( p > 0.05) in any of the tested bonding strategies.
The resin–dentin interface created using the control resin (Res.CTR) showed a significant decrease in the nano-elasticity ( p < 0.05) of the hybrid layer (2nd indentation) both after 30 and 90 days of storage in AS ( Table 1 ); the bottom of the hybrid layer showed no change in the Ei values ( p > 0.05). The same resin applied after dentin pre-treatment using the primers containing PLA or PLA + TMP showed no significant change ( p > 0.05) in the Ei values of the 2nd indentation at the hybrid layer only after 30 days of AS storage; these values dropped significantly ( p > 0.05) after 90 days of AS immersion. However, the specimens created with Res.CTR/PLA and Res.CTR/TMP + PLA showed no significant Ei change ( p > 0.05) at the bottom of the hybrid layer both after 30 and 90 days of AS storage.
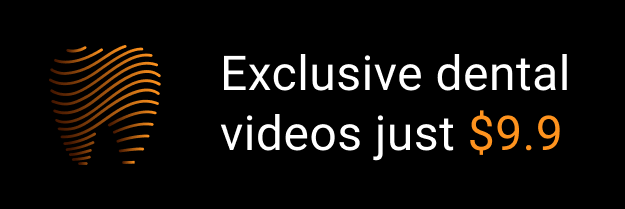