Highlights
- •
We prepared novel bioactive glass (BAG) and fluoride-containing BAG composites.
- •
The effects of biofilm on the optical properties of these composites were investigated.
- •
The biofilm may contribute to decreasing the color change of these composites.
- •
Bacterial Biofilm decreased the translucency of the highly polished BAG composites.
Abstract
Objective
Bioactive glass (BAG) has been suggested as a possible additive for dental restorative materials because of its antimicrobial effect and potential for promoting apatite formation in body fluids. The purpose of this study was to investigate the effects of bacterial biofilm on the change of colorimetric value and translucency of novel BAG-containing composites having different initial surface roughness.
Methods
Composites with 72 wt% total filler load were prepared by replacing 15% of the silanized Sr glass with BAG (65 mol % Si; 4% P; 31% Ca), BAG-F (61% Si; 31% Ca; 4% P; 3% F; 1% B), or silanized silica. Light-cured discs of 2-mm thickness ( n = 10/group) were divided into 4 different surface roughness subgroups produced by wet polishing with 600 and then up to 1200, 2400, or 4000 grit SiC. CIE L*a*b* were measured and the color difference and translucency parameter (TP) were calculated before and after incubating in media with or without a Streptococcus mutans (UA 159) biofilm for 2 wks (no agitation). Results were analyzed using ANOVA/Tukey’s test ( α = 0.05).
Results
All the color differences for BAG and BAG-F composite showed significant decreases with bacterial biofilm compared to media-only. The mean TP (SD) of BAG and BAG-F composite before aging [10.0 (2.8) and 8.5 (1.4)] was higher than that of the control composite [4.9 (0.8)], while the change in TP with aging was greater compared to the control with or without bacteria. BAG-F composites with the smoothest surfaces showed a greater decrease in TP under bacterial biofilm compared to the BAG composite.
Significance
Highly polished dental composites containing bioactive glass additives may become slightly rougher and show reduced translucency when exposed to bacterial biofilms, but do not discolor any more than control composites that do not contain the BAG.
1
Introduction
New dental composites are being developed with bioactive additives having the potential to render them less susceptible to oral bacteria and promoting of tooth remineralization . These composites will be used in all areas of the mouth, and will therefore have the same requirement for initial and long term esthetics as current materials.
Bioactive glasses (BAGs) area group of ceramic materials with the ability to bond chemically to both soft and hard tissues . BAGs are considered a potential candidate as filler particles in resin-based dental composites, because they can enhance hard tissue regeneration and show some antimicrobial effect on oral microorganisms by the dissolution of the glass and releasing of ions in body fluids . A recent study reported that BAG containing composites showed mechanical properties comparable to commercial composites . Also, adding a filler particle based on a fluoride-containing BAG (BAG-F) enabled the composite to release both calcium and fluoride ions in solution, and to be rechargeable with fluoride upon exposure to external fluoride solutions . While these properties provide optimism about the potential use of BAG additives in composites, the potential dissolution of the glass filler may cause concern about the integrity of the resin composite surface, and its overall esthetic appearance during aging.
The color of a resin composite is influenced by various factors including its light scattering and absorption characteristics, light reflectivity and translucency . When light passes through a resin composite, it can be scattered in many different directions, primarily at the surface of the filler particles. Some of the light passes directly through as a more straight-line transmission while other light scatters through diffuse transmission, depending on the thickness of the composite . The characteristics of the fillers and other additives used in the materials will therefore play a very significant role in determining their esthetic properties.
The characteristic of translucency allows an underlying background to show through by allowing light to at least partially pass through a material . The inherent translucency of resin composites can be clinically beneficial for shade matching with an adjacent tooth by allowing the underlying and adjacent tooth structure to reflect or show through the restoration . The translucency of tooth-colored restorative material is considered no less important than color, because the material with the same composite shade can look significantly different over different background colors . A study reported that the translucency of resin composite was significantly correlated with diffuse light transmission, but not with the straight-line transmission . It has been shown that the shape, size, and content of filler particles are all capable of affecting the light transmittance characteristics and color of resin composites .
Dental composites are susceptible to discoloration after prolonged exposure to the oral environment, and this is a function of their formulation . One of the primary reasons dental composite restorations are replaced is due to unacceptable color change . It is clinically important for these materials to maintain color stability for prolonged periods of time . Therefore, conditions in which changes in color and translucency are produced within or on the surface during aging may cause the restoration to become clinically unacceptable. For example, it has been shown that organic acids and enzymes produced by bacteria within the oral biofilm can soften the resin matrix of dental composites , which can increase the susceptibility of the surface to staining , and may therefore influence the overall esthetics of the restoration. However, little is known about the direct effect of bacterial biofilm formation on the stability of the optical properties of dental composites.
As new dental composite materials are produced with additives, such as BAG and calcium phosphates that may impart bioactive characteristics, it will be important to evaluate the color stability during aging, especially after exposure to clinically relevant oral conditions, such as biofilm formation. The aim of this study was to investigate the effect of bacterial biofilm on the optical properties of composites with different levels of polish, especially those containing potentially bioactive additives such as BAG and BAG-F. The hypothesis to be tested was that all composites would experience a change in color and translucency as a result of surface degradation, but that the BAG and BAG-F-containing composites would experience less change due to the presence of some antimicrobial effect. It was also expected that aging in media with or without bacterial would cause a dissolution of the BAG, creating a slight surface roughening, especially when the composite was highly polished. However, the effect of this roughening on the color change, if any, was not predictable and therefore important to assess.
2
Materials and methods
2.1
Preparation of BAG
BAG fillers were synthesized via sol–gel methods in our lab as previously described . The synthetic glasses were ball milled in ethanol and sieved to a total particle size of less than 38 μm. The particles were then further processed using a Micronizer Jet Mill (Sturtevant Inc., Hanover, MA, USA), determined by laser particle size measurements (Beckman Coulter LS13 320, Brea, CA, USA) to routinely produce a fine particle size range (0.04–3.0 μm).
2.2
Formulation of experimental composites
The three experimental composites all contained 57 wt% of strontium glass filler treated with silane (1–3 μm average size, Bisco, Inc.), further modified as follows: The control group included a silane-treated aerosol-silica (OX-50), while micronized BAG (BAG65) and fluorine-containing micronized BAG (BAG61) replaced the silica in the groups designated as BAG and BAG-F ( Table 1 ). The fillers were mixed with Bis-GMA and TEGDMA monomers in a 50:50 formulation using a centrifugal mixing device (Speed-Mixer DAC 150 FVZ, Hauschild, Germany) for 2 min at 2400 rpm.
Group | Fillers | Monomers |
---|---|---|
Control | 57 wt% SG, 15 wt% OX-50 | 28 wt% combination of Bis-GMA and TEGDMA (50:50) with 0.4 wt% CQ photoinitiator, 0.8 wt% EDMAB tertiary amine accelerator, and 0.05 wt% BHT inhibitor. |
BAG | 57 wt% SG, 15 wt% BAG65 | |
BAG-F | 57 wt% SG, 15 wt% BAG61 |
2.3
Specimen preparation
Forty disk-shaped specimens of each composite group (10 mm diameter by 2 mm thickness) were prepared in vinyl polysiloxane molds with both top and bottom surfaces pressed with microscope slides to extrude excess resin. Specimens were light-activated using a curing unit (Demi™, Kerr, Orange, CA, USA) for 40 s on each side at 520–580 mW/cm 2 . Specimens were separated from the mold and any flash on the side edge was carefully removed. They were then aged dry for 24 h. A holder was created to maintain firm hand pressure on the specimen while it was rotating under cooling water on a polishing wheel covered with silicon carbide paper (Struers Inc., Cleveland, OH, USA). Every group was divided into four polishing subgroups of twelve specimens each in order to produce four distinct ranges of surface roughness as previously described ; Subgroup P600 was polished only on 600-grit SiC paper; P1200 sequentially on 600- and 1200-grit; P2400 sequentially on 600-, 1200-, and 2400-grit; and P4000 sequentially on 600-, 1200-, 2400-, and 4000-grit. The baseline surface roughness (Ra, μm) was measured using a surface roughness tester (TR200, TIME Group, Pittsburgh, PA, USA) at four different positions by rotating 90 degrees clockwise between measurements with five cut-offs of 0.25 mm each for a total length measured of 1.25 mm.
2.4
Colorimetric evaluation
Initial color for all specimens was measured by CIE values, L * (lightness), a * (red-green coordinate), and b * (yellow-blue coordinate) against a white background and a black background using a Chroma Meter (CR-221; Minolta, Osaka, Japan). The device has a 3-mm diameter measuring area and uses 45-degree circumferential illumination and a 0-degree viewing angle geometry for measuring precise areas of surfaces. The color values for each background alone were as follows, based on three individual measurements: white background ( L * = 93.879 ± 0.106, a * = 2.148 ± 0.044, b * = −5.757 ± 0.173) and black background ( L * = 12.197 ± 0.219, a * = −0.010 ± 0.084, b * = −1.075 ± 0.077). Calibration of the chromameter was performed before each measuring session.
2.5
Biofilm procedure
Overnight cultures of Streptococcus mutans (strain UA159) grown in brain heart infusion (BHI) at 37 °C in a 5% CO 2 incubator were measured for optical density at 600 nm (OD 600 ) and then diluted to an OD 600 of 0.4–0.6. A 1:10 dilution of the stock solution in new BHI medium was then incubated for 3 h to obtain OD 600 = 0.3, which we previously determined from calibration curves represents a bacterial concentration of 9 × 10 7 CFU/mL. Culture media were prepared by adding 3 wt% sterile sucrose (Fischer Science Education, Hanover Park, IL, USA) to trypticase soy broth (BBL™ Trypticase™ Soy Broth, BD diagnostics, MD, USA). Five specimens from each surface roughness group were randomly chosen for the biofilm exposure group and were inoculated with S. mutans . An additional five specimens were used for the group to be aged in media only. All specimens ( n = 120) were sterilized in 70% ethyl alcohol in an ultrasonic bath for 15 min followed by 100% ethyl alcohol for 15 min. The sample disks were placed in six well culture plates. For the S. mutans group, a subculture (1:100) of S. mutans was added to the culture media and 5 mL was placed in each well using a sterilized pipette. For the control group, only 5 mL of the culture media free of S. mutans was placed in an individual well. All specimens were incubated with 5% CO 2 at 37 °C for 14 days, with the culture media being removed every 24 h and replaced with 5 mL of fresh sterilized culture media using a sterilized pipette. On the 13th experimental day, the samples were examined for contamination by culturing in BBL™ Trypticase™ Soy Agar with 5% Sheep Blood (BD diagnostics, Hunt Valley, MD, USA). The biofilms were then removed from the samples by gently wiping with Kimwipes™ (Kimberly-Clark, Dallas, TX, USA) and rinsing with sterile water.
2.6
Post incubation color assessment
Ra, and colorimetric value measurements on both the white and black backgrounds, were repeated on the surfaces after biofilm removal, in the same way, as the baseline measurements.
2.7
Calculation of translucency and the color difference ( <SPAN role=presentation tabIndex=0 id=MathJax-Element-1-Frame class=MathJax style="POSITION: relative" data-mathml='ΔEab*’>ΔE∗abΔEab*
Δ E a b *
)
The translucency of composite materials before and after treatment is usually measured using the translucency parameter (TP) . TP was obtained by calculating the color difference of the specimen over a black (B) and white background (W) according to the following formula:
TP = [ ( L W * − L B * ) 2 + ( a W * − a B * ) 2 + ( b W * − b B * ) 2 ] 1 / 2
If the material is absolutely opaque, the TP is zero. The higher the TP value, the more translucent is the composite material.
The total color difference ( <SPAN role=presentation tabIndex=0 id=MathJax-Element-3-Frame class=MathJax style="POSITION: relative" data-mathml='ΔEab*’>ΔE∗abΔEab*
Δ E a b *
) from baseline to post-treatment was calculated for each specimen as:
Δ E a b * = [ ( Δ L * ) 2 + ( Δ a * ) 2 + ( Δ b * ) 2 ] 1 / 2
Where <SPAN role=presentation tabIndex=0 id=MathJax-Element-5-Frame class=MathJax style="POSITION: relative" data-mathml='ΔL*=Lf*−LI*’>ΔL∗=L∗f−L∗IΔL*=Lf*−LI*
Δ L * = L f * − L I *
; <SPAN role=presentation tabIndex=0 id=MathJax-Element-6-Frame class=MathJax style="POSITION: relative" data-mathml='Δa*=af*−aI*’>Δa∗=a∗f−a∗IΔa*=af*−aI*
Δ a * = a f * − a I *
; <SPAN role=presentation tabIndex=0 id=MathJax-Element-7-Frame class=MathJax style="POSITION: relative" data-mathml='Δb*=bf*−bi*’>Δb∗=b∗f−b∗iΔb*=bf*−bi*
Δ b * = b f * − b i *
. <SPAN role=presentation tabIndex=0 id=MathJax-Element-8-Frame class=MathJax style="POSITION: relative" data-mathml='LI*’>L∗ILI*
L I *
, <SPAN role=presentation tabIndex=0 id=MathJax-Element-9-Frame class=MathJax style="POSITION: relative" data-mathml='aI*’>a∗IaI*
a I *
, <SPAN role=presentation tabIndex=0 id=MathJax-Element-10-Frame class=MathJax style="POSITION: relative" data-mathml='bi*’>b∗ibi*
b i *
are referred to as the initial color measurement and <SPAN role=presentation tabIndex=0 id=MathJax-Element-11-Frame class=MathJax style="POSITION: relative" data-mathml='Lf*’>L∗fLf*
L f *
, <SPAN role=presentation tabIndex=0 id=MathJax-Element-12-Frame class=MathJax style="POSITION: relative" data-mathml='af*’>a∗faf*
a f *
, <SPAN role=presentation tabIndex=0 id=MathJax-Element-13-Frame class=MathJax style="POSITION: relative" data-mathml='bf*’>b∗fbf*
b f *
as the final color measurement.
2.8
Statistical analysis
Statistical comparisons of changes of <SPAN role=presentation tabIndex=0 id=MathJax-Element-14-Frame class=MathJax style="POSITION: relative" data-mathml='ΔEab*’>ΔE∗abΔEab*
Δ E a b *
, Ra, and TP between pre- and post-treatment were performed among each polishing level of P600, P1200, P2400, and P4000 for all three materials. The normality of the studied parameters was tested using the Kolmogorov–Smirnov test. If all followed a normal distribution, the values n each group were compared by paired t -test. Otherwise, Wilcoxon Signed Ranks Test was used. For within-group comparison, if the data fulfilled the assumptions of normality and homogeneity of variance (Levene’s test), they were compared by ANOVA. According to the results of the Levene statistic, post hoc comparisons were conducted using Tukey’s or Dunnett’s T3. If the parameters were not normally distributed, they were analyzed using the Kruskal–Wallis test. All statistical analyses were performed at α = 0.05 using SPSS 21 (IBM Corp., Somers, NY, USA).
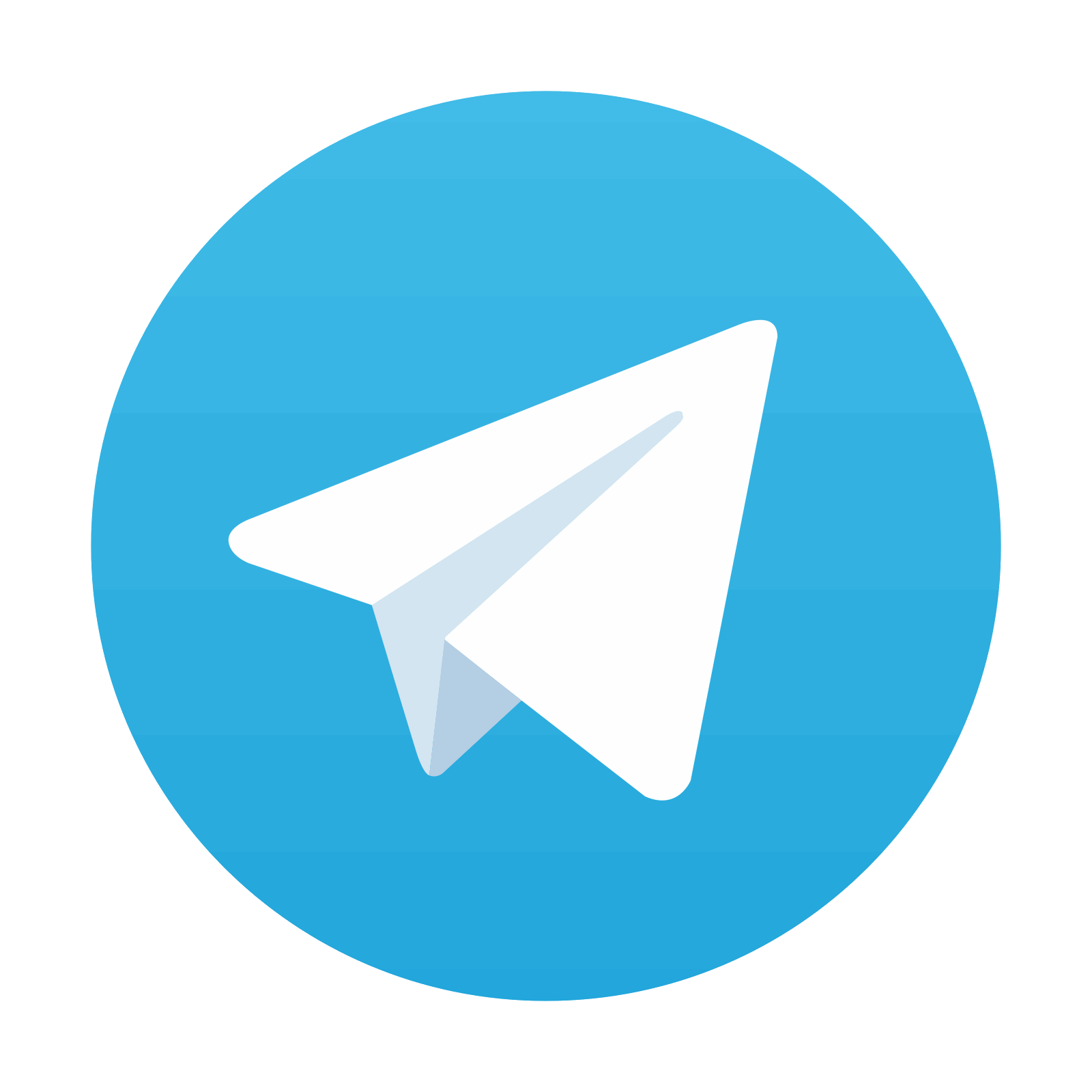
Stay updated, free dental videos. Join our Telegram channel

VIDEdental - Online dental courses
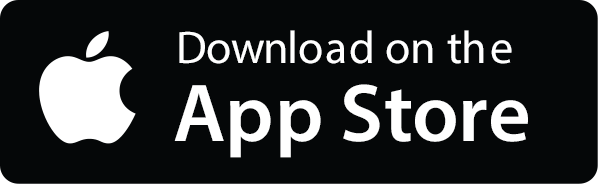
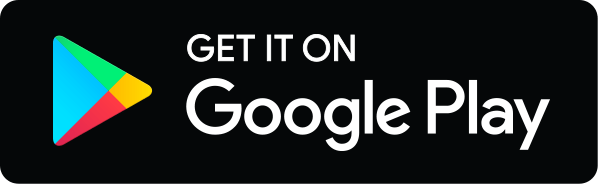