Highlights
- •
A novel method for inducing bioactivity of dental ceramic/bioglass composites by using laser energy has been proposed.
- •
Application of specific laser irradiation parameters results in 3 times faster formation of HA coating compared to conventional biomimetic method.
- •
A HA film with mineral composition close to dental cementum could be obtained.
- •
LAB process is potentially applicable for functionalization of materials with low bioactivity under precise control of laser irradiation parameters.
Abstract
Objectives
Aims of this study were to investigate the optimal conditions of laser irradiation of a novel Bioactive Glass/Dental Ceramic-BP67 composite for acceleration of hydroxyapatite-HA formation and to assess cellular responses on the precipitated HA region.
Methods
BP67 (Bioactive Glass: 33.3%, Dental Ceramic: 66.7%) was fabricated by the sol–gel method. A laser assisted biomimetic-LAB process was applied to BP67 sintered specimens immersed in 1.5-times concentrated simulated body fluid-1.5×-SBF. The effect of various energy densities of pulsed nanosecond Nd-YAG (1064 nm) laser and irradiation exposure times (30 min, 1 and 3 h) were evaluated for HA precipitation. The HA film was characterized by FTIR, XRD, SEM and micro Raman techniques. ICP-AES was used for revealing changes in chemical composition of the 1.5×-SBF during irradiation. Cell viability and morphological characteristics of periodontal ligament fibroblasts-PDLFs, human gingival fibroblasts-HGFs and SAOS-2 osteoblasts on the HA surface were evaluated by MTT assays and SEM.
Results
At optimal energy fluence of 1.52 J/cm 2 and irradiation time for 3 h followed by immersion in 1.5×-SBF at 60 °C, a dense HA layer was formed on laser-irradiated BP67 within 7 days. The resulting HA film was tightly bonded to the underlying substrate and had mineral composition similar to cementum. MTT assay showed a consistent reduction of cell proliferation on the HA layer in comparison to conventional control ceramic and BP67 for all 3 cell lines studied.
Significance
These findings suggest LAB is an effective method for acceleration of HA formation on materials with low bioactivity, while cellular responses need further investigation.
1
Introduction
Bioactive glasses are applied for bone repair and regeneration, because of their excellent biocompatibility and osteoconductivity . Upon implantation, they form a layer of biological hydroxyapatite on their surface, which enables their direct bonding to the adjacent bone or, even to soft tissues . Nevertheless, bioactive glasses exhibit low mechanical strength , high degradation rates and mismatch of thermal expansion coefficients with structural ceramics , which limit their applications as coating materials for dental and bone replacing implants.
Recently, various bioactive glass formulations containing K 2 O, Na 2 O and other metal oxides have been proposed as suitable coatings on zirconia implants in order to reduce healing time or enhance osteointegration rate even in osteoporotic bone . Novel bioactive glass (B)/dental feldspahic porcelain (P) composites could be potentially applied for coating of zirconia dental implants in order to improve their bone-bonding ability. As it was recently reported, B/P materials with high dental ceramic content (60–80%) present sufficient flexural strength and are able to form a layer of non-stoichiometric biological hydroxyapatite (HA) on their surface in simulated body fluids (SBFs) . However, due to the high amount of leucite phase present in the structure of conventional ceramics, these materials exhibit significant delay in HA crystallization.
The precipitation of HA on the surface of bioactive ceramics during exposure to body fluids is based on a sequence of surface reactions that lead to dissolution of silica network and formation of high surface-area, hydrated silica-rich layer, which promotes heterogeneous nucleation of HA . The rate of nucleation reactions mainly depends on activation energy of nucleation and solution supersaturation . As soon as Ca-P nuclei are initially formed on the substrate, they continue to grow out into mature HA crystals by incorporating the calcium and phosphate ions from the solution followed by phase transformation reactions .
Using an approach that mimics the process of biological apatite formation, a novel method has been developed that utilizes a laser beam to deliver energy in the system and enhance the Ca-P deposition . This, laser assisted biomimetic (LAB) method is based on the laser irradiation of a solid substrate while immersed in supersaturated SBF solution at ambient temperature and pressure. During LAB process the laser energy accelerates CaP nucleation onto surface of the material by activating several chemical and physical processes in the solid-liquid interface. By this way, the time needed for HA deposition can be significantly reduced to some days or even few hours . In particular, Pramatarova et al. developed a CaP coating for ion-modified surfaces of stainless steel, silicon and silica glass substrates using LAB with a high-energy pulsed CuBr laser followed by aging in a supersaturated CaP solution for 24 h. Recently, Oyane et al. applied this coating technique to a EVOH polymer and sintered hydroxyapatite substrates immersed in a supersaturated CaP solution using a pulsed Nd:YAG laser. Moreover, it has already been reported by our group, that precipitation of HA film on the surface of BP50 (50% of bioactive glass, 50% of dental ceramic) material could be induced by pulsed microsecond Nd:YAG and Er:YAG lasers . However, the application of the LAB method to bioactive ceramic composites with high wt % of dental ceramic has not been attempted so far.
Based on the aforementioned, it was the objective of this study to investigate the optimal conditions of laser irradiation of a novel, high wt % of dental ceramic, BP67 composite (Bioactive Glass 33.3%, Dental Ceramic 66.7%) for acceleration of HA formation on its surface. An additional purpose was to investigate the topography and chemical composition of the deposited HA film and to assess cellular responses to the HA surface. The two research hypotheses were that certain conditions of laser energy irradiation can induce the formation of HA on the surface of BP67 composites in short time period and that the HA film is able to enhance proliferation of different cell types, including osteoblasts, and gingival and periodontal fibroblasts.
2
Materials and methods
2.1
Preparation of specimens
The experimental procedures included the fabrication of disc shaped control specimens made of commercial ceramic for margins (P) of MCRs (IPS Inline-Margin Ceramic System, Ivoclar, Schaan, Liechtenstein) as well as specimens of novel bioactive glass-dental ceramic composite material BP67 (BPc), containing 33.3 wt% of 58S bioactive glass and 66.7 wt% of dental ceramic (IPS Inline Margin, Ivoclar).
Novel BP67 composite material was synthesized by sol–gel method, as previously described . Briefly, the precursors of bioactive glass 58S (SiO 2 60 wt%, CaO 36 wt% and P 2 O 5 4 wt%) distilled water, nitric acid, tetraethyoxysilane (TEOS), tri-ethyl-phosphate (TEP) and calcium nitrate tetra-hydrate (Ca(NO 3 ) 2 4H 2 O) have been mixed together. The TEOS to water ratio (R ratio) used was 1.39 . Dental porcelain was added in a ratio of 66.7%. After mixing of the components, the sol was sintered at 60 °C for 55 h, dried, and underwent firing cycle up to 700 °C in a programmable furnace (Nabertherm, Germany), so that the material remained at the stabilization temperature (700 °C) for 18 h. The resulting products were pulverized and sieved to powders of <40 μm.
For the fabrication of the specimens, the powders of BP67 and dental ceramic were mixed with modeling liquid (IPS Classic Margin Build-Up Liquid, Ivoclar), using the same liquid to powder ratio (0.335), transferred into silicone moulds and condensed using a vibrator, to accomplish excess liquid removal. The discs (d = 6 mm × 1.5 mm) were then removed and sintered in a ceramic oven (Programat P95, Ivoclar) at 930 °C with a rate of 60 °C/min under vacuum and according to manufacturers’ instructions for the conventional ceramic.
2.2
Preparation of 1.5 × SBF solution
Simulated body fluid concentrated 1.5-times (1.5×-SBF) was prepared by weighing and dissolving NaCl, NaHCO 3 , KCl, K 2 HPO 4 ·3H 2 O, MgCl 2 ·6H 2 O, CaCl 2 , and NaSO 4 in distilled water, as previously described . The pH of the solution was adjusted to 7.3–7.4 at a temperature of 36.5 °C in a water bath by the addition of 1.0 M hydrochloric acid (HCl) and tris-hydroxymethylaminomethane (CH 2 OH) 3 CNH 2.
2.3
Laser assisted biomimetic process—LAB
2.3.1
Experimental setup
The experiments were carried out using the 1064 nm line of a Q-switched Nd:YAG laser (Quantel YG980) emitting 8 ns duration pulses at 10 Hz maximum repetition rate. The pulse energy was adjustable from a maximum of 650 mJ down to almost zero.
The laser beam was directed through 50/50% beam splitter and a mirror and then focused by a 60 mm focal length lens onto the surface of the BP sample. The sample to be processed was immersed in 15 ml of 1.5 × SBF in a Plexiglas container, so that its surface was perpendicular to the incident laser beam. In turn, the container with sample and 1.5 × SBF liquid was mounted on a computer-controlled xy motorized transition stage, so that the sample’s irradiated area could be selected before each laser pulse. Laser energy was continuously monitored by a pyroelectric energy detector (gentec-EO), connected to a supervisory PC recording the energy of every single pulse. The focal area of the laser beam on the BP surface was controlled micro-metrically by adjusting the sample to lens distance.
2.3.2
Estimation of the ablation behavior of the material
In order to estimate the optimum value of laser energy density that would disrupt the energy state of the surface of material without causing structural damage, the samples were irradiated by single pulse, 10 pulses or 3000 pulses with a frequency of 10 Hz and at energy densities ranging from 1.19 to 4.10 J/cm 2 . The calculated spot size was 1.52 mm. The samples were then carbon coated and examined by Scanning Electron Microscopy-SEM (JEOL J.S.M. 840A, Tokyo, Japan) in 50× magnifications.
2.3.3
Irradiation of the specimens for different time periods
The LAB process was applied to BP67 sintered specimens. The sample-mirror distance was set at 32 mm and the calculated spot diameter was 2.82 mm. The laser beam was directed to the center of each sample, while it was immersed in 15 ml of 1.5 × SBF precursor liquid. Specimens were irradiated at laser fluence 1.52 J/cm 2 for 30 min (BP_30), 1 h (BP_1) or 3 h (BP_3). Immediately after laser treatment, specimens were re-immersed in 1.5 × SBF and incubated at 60 °C for up to 7 days (d). Laser-untreated BP67 specimens incubated under the same conditions were used as control (BP_c). The solution was renewed after 6 h, 24 h and then every 2 days, and the solid to liquid ratio was preserved at 1 mg/1 ml. At the end of the incubation period, the samples were removed from the 1.5 × SBF solution, rinsed with acetone and distilled water and characterized by FTIR, XRD, SEM-EDS and micro-Raman techniques.
2.4
Surface characterization of the samples by means of different techniques
2.4.1
Fourier transformed infrared (FTIR) analysis
FTIR reflectance spectra were obtained by a FTIR Spectrometer (IFS113v, Bruker) in the MIR (4000–400 cm −1 ) region with a number of 64 scans per spectrum and a resolution 2 cm −1 .
2.4.2
X-ray diffraction (XRD) analysis
The XRD analysis was performed using a Philips PW1710 diffractometer with Ni-filtered CuKα radiation on the surface of the studied samples. The counting statistics were: start angle 5°, end angle 75° (2 θ ), step size 0.05° (2 θ ), time per step 1 s and scan speed 0.01°2 θ /s. The ICDD PDF-4+ (2009) database was used for the identification of the phases contained in the studied samples.
2.4.3
Micro Raman analysis
Raman measurements were conducted with a LabRAM HR (Horiba) or a T64000 (Horiba) spectrometer equipped with a Peltier and a liquid nitrogen cooled charge coupled device, respectively. Excitation was provided by a Cobolt Fandango™ diode pumped solid state laser at 515 nm with a power of ∼9 mW focused on the sample by a standard 10× objective at a spot diameter of ∼2.5 μm. Presented spectra were collected from an area of 50 × 50 μm 2 , taking advantage of the DuoScan™ scanner. It allows fast scanning of the laser beam on the sample, resulting in more representative spectra and lower effective average power.
2.4.4
Scanning electron microcopy and energy dispersive X-ray microanalysis (SEM-EDS)
For topographical evaluation and analysis of surface elemental composition the specimens were carbon coated with an average thickness of 200 Å, using a vacuum evaporator JEOL-4X and were observed using a Scanning Electron Microscope (JEOL J.S.M. 840A, Tokyo, Japan) with associated EDS (Oxford INKA-300). All analyses were done with a 20 kV accelerating voltage and 0.4 mA probe. The images were taken in magnification ×1000. The cross-sections were prepared by embedding the specimens in epoxy resin (Epofix resin, Struers) under vacuum, followed by cutting using a water-cooled, diamond rotary saw. Sections were ground by hand using 400, 600, 800 and 1000 grit silicon carbide and polished in turn with diamond paste with a grain diameter of 6, 3 and 1 μm.
2.5
Investigation of changes in chemical composition of the 1.5 × SBF before and after laser irradiation
In order to evaluate changes in chemical composition of the 1.5 × SBF solution before and after laser irradiation for 3 h, Si, P, Al and Ca concentrations were analyzed in the same reacted solutions by Inductively Coupled Plasma Atomic Emission Spectrometry (ICP-AES), using a PerkinElmer 3100XL Axial Viewing Spectrometer, equipped with a cyclonic spray chamber. The sample introduction flow rate was 2 ml/min. Detection limits were as follows: Si 4+ (0.05 ppm), Al 3+ (0.01 ppm), Ca 2+ (0.005 ppm) and for P (0.03 ppm). Potential temperature changes of the 1.5 × SBF solution were monitored after 30 min, 1 h and 3 h of LAB process by a digital platinum resistance thermometer (Guildline 9540/B), while the pH of the 1.5 × SBF solution was measured before and after 3 h of laser irradiation (B&C PH125,2 pH/ORP meter), as well as during the process of liquid renewal.
2.6
Establishment of primary cell cultures
Human primary gingival fibroblast (hGF) and human primary periodontal ligament fibroblast (PDLF) cell cultures were established from free gingiva and periodontal ligament biopsies respectively of young healthy donors after routine third molar extraction. The research protocol was approved by the Ethical committee of the Institutional review board and all patients signed an informed consent form. The gingival tissue biopsies were minced into 1–2 mm fragments and placed in 25 cm 2 culture flasks with Dulbecco modified Eagle medium (DMEM) supplemented with 10% Fetal Bovine Serum (FBS) and antibiotics/antimycotics (100 units/ml penicillin, 100 mg/ml streptomycin and 0.25 mg/ml Amphotericin B). (all from Invitrogen, Karlsruhe, Germany) The flasks were incubated at 37 °C in humidified atmosphere of 5% CO 2 . After reaching confluency, the cells were transferred to a larger 75 cm 2 flask (passage 1), and then continuously subcultured for further experiments. Cells from passages 3–6 were used for all experiments.
For the establishment of PDLF cultures the teeth were washed with sterile PBS and disinfected with sodium hypochlorite and then placed into a falcon tube containing 0.25% trypsin/1 mM Ethylene Diamine Tetraacetic Acid-EDTA solution and kept in water bath at 37 °C for 45 min. Subsequently, complete culture medium (DMEM with 10% FBS and antibiotics/antimycotics) was added into the falcon tube to stop the trypsin action. The culture medium containing cells was transferred into a new centrifuge tube and centrifuged for 10 min at 115 × g . The supernatant was discarded and the cells were re-suspended in fresh medium and pipetted into 25 cm 2 culture flasks. The flasks were incubated at 37 °C in humidified atmosphere of 5% CO 2 . After reaching confluency, the cells were transferred to a 75 cm 2 flask (passage 1), and then continuously subcultured for further experiments. Cells from passages 2–4 were used for all experiments.
SAOS-2 osteoblast-like cell line was obtained from the American Type Culture Collection (ATCC No. HTB 85). Cells were cultured at 37 °C in humidified atmosphere of 5% CO 2 in a-Modification of Eagle’s Medium (aMEM) (Invitrogen), supplemented with 10% FBS and antibiotics/antimycotics, as described above.
2.7
Evaluation of viability by the MTT assay
For the evaluation of viability of cells seeded in direct contact with the experimental materials, the MTT [3-(4,5-dimethylthiazol-2-yl)-2,5-diphenyltetrazolium bromide] assay was performed. Three groups of ceramic specimens (6 × 1.5 mm), prepared as described above were tested: (1) feldspathic dental ceramic (P), (2) bioactive glass ceramic composite (BP) and (3) laser-treated BP specimens coated with the developed HA layer (BP/HA specimens) (laser irradiated for 3 h and subsequent aging in 1.5 × SBF solution at 60 °C for 7 days). Prior to cell culture, sintered specimens were sterilized in an autoclave for 20 min at 2 bar pressure and then placed in each well of a 48-well plate (Corning, Cambridge, MA) and conditioned in 0.5 mL of culture medium for 24 h at 37 °C in an incubator. Cells (HGFs, PDLFs or SAOS-2) were seeded at a density of 2.5 × 10 4 for HGFs and PDLFs and 5 × 10 4 for SAOS-2 on the top of the experimental specimens. Cells grown on tissue culture plastic (C) were simultaneously evaluated as control.
After 24, 48 or 72 h, MTT (Sigma Aldrich, Taufkirchen, Germany) at final concentration of 0.5 mg/ml was added in each well. Afterwards, the plates were incubated at 37 °C for an additional 3 h. The purple formazan crystals that were formed were diluted by dimethyl-sulfoxide (DMSO) (Sigma Aldrich) for 1.5 h at 37 °C . The absorbance was measured against blank (DMSO) at a wavelength of 545 nm and a reference filter of 630 nm by a microplate reader (Epock, Biotek, Biotek instruments, Inc., Vermont, U.S.A). Experiments were performed in four replicates and repeated three times. The results were expressed as the averaged absorbance as % percentage of the control value.
2.8
SEM evaluation of the cell morphology
After 72 h of cell culturing, the specimens were washed twice with PBS, fixed in 3% glutaraldehyde in 0.1 M sodium cacodylate buffer containing 0,1 M sucrose (pH 7.4), dehydrated in a graded series of alcohols (35%, 50%, 70%, 95%, and two changes of 100% ethanol) and air-dried in a hood for 20 min. Finally, the specimens were carbon coated and observed by SEM (JEOL J.S.M. 840A) in magnification ×1000.
2.9
Statistical analysis
Statistical analysis of the cell viability data was performed using two-way ANOVA analysis. Follow up comparisons between groups were carried out using Tukey’s post-hoc test. Analysis was performed with Prism 6.0 Software (GraphPad, CA, USA) and the level of statistical difference was 0.05 ( p < 0.05).
2
Materials and methods
2.1
Preparation of specimens
The experimental procedures included the fabrication of disc shaped control specimens made of commercial ceramic for margins (P) of MCRs (IPS Inline-Margin Ceramic System, Ivoclar, Schaan, Liechtenstein) as well as specimens of novel bioactive glass-dental ceramic composite material BP67 (BPc), containing 33.3 wt% of 58S bioactive glass and 66.7 wt% of dental ceramic (IPS Inline Margin, Ivoclar).
Novel BP67 composite material was synthesized by sol–gel method, as previously described . Briefly, the precursors of bioactive glass 58S (SiO 2 60 wt%, CaO 36 wt% and P 2 O 5 4 wt%) distilled water, nitric acid, tetraethyoxysilane (TEOS), tri-ethyl-phosphate (TEP) and calcium nitrate tetra-hydrate (Ca(NO 3 ) 2 4H 2 O) have been mixed together. The TEOS to water ratio (R ratio) used was 1.39 . Dental porcelain was added in a ratio of 66.7%. After mixing of the components, the sol was sintered at 60 °C for 55 h, dried, and underwent firing cycle up to 700 °C in a programmable furnace (Nabertherm, Germany), so that the material remained at the stabilization temperature (700 °C) for 18 h. The resulting products were pulverized and sieved to powders of <40 μm.
For the fabrication of the specimens, the powders of BP67 and dental ceramic were mixed with modeling liquid (IPS Classic Margin Build-Up Liquid, Ivoclar), using the same liquid to powder ratio (0.335), transferred into silicone moulds and condensed using a vibrator, to accomplish excess liquid removal. The discs (d = 6 mm × 1.5 mm) were then removed and sintered in a ceramic oven (Programat P95, Ivoclar) at 930 °C with a rate of 60 °C/min under vacuum and according to manufacturers’ instructions for the conventional ceramic.
2.2
Preparation of 1.5 × SBF solution
Simulated body fluid concentrated 1.5-times (1.5×-SBF) was prepared by weighing and dissolving NaCl, NaHCO 3 , KCl, K 2 HPO 4 ·3H 2 O, MgCl 2 ·6H 2 O, CaCl 2 , and NaSO 4 in distilled water, as previously described . The pH of the solution was adjusted to 7.3–7.4 at a temperature of 36.5 °C in a water bath by the addition of 1.0 M hydrochloric acid (HCl) and tris-hydroxymethylaminomethane (CH 2 OH) 3 CNH 2.
2.3
Laser assisted biomimetic process—LAB
2.3.1
Experimental setup
The experiments were carried out using the 1064 nm line of a Q-switched Nd:YAG laser (Quantel YG980) emitting 8 ns duration pulses at 10 Hz maximum repetition rate. The pulse energy was adjustable from a maximum of 650 mJ down to almost zero.
The laser beam was directed through 50/50% beam splitter and a mirror and then focused by a 60 mm focal length lens onto the surface of the BP sample. The sample to be processed was immersed in 15 ml of 1.5 × SBF in a Plexiglas container, so that its surface was perpendicular to the incident laser beam. In turn, the container with sample and 1.5 × SBF liquid was mounted on a computer-controlled xy motorized transition stage, so that the sample’s irradiated area could be selected before each laser pulse. Laser energy was continuously monitored by a pyroelectric energy detector (gentec-EO), connected to a supervisory PC recording the energy of every single pulse. The focal area of the laser beam on the BP surface was controlled micro-metrically by adjusting the sample to lens distance.
2.3.2
Estimation of the ablation behavior of the material
In order to estimate the optimum value of laser energy density that would disrupt the energy state of the surface of material without causing structural damage, the samples were irradiated by single pulse, 10 pulses or 3000 pulses with a frequency of 10 Hz and at energy densities ranging from 1.19 to 4.10 J/cm 2 . The calculated spot size was 1.52 mm. The samples were then carbon coated and examined by Scanning Electron Microscopy-SEM (JEOL J.S.M. 840A, Tokyo, Japan) in 50× magnifications.
2.3.3
Irradiation of the specimens for different time periods
The LAB process was applied to BP67 sintered specimens. The sample-mirror distance was set at 32 mm and the calculated spot diameter was 2.82 mm. The laser beam was directed to the center of each sample, while it was immersed in 15 ml of 1.5 × SBF precursor liquid. Specimens were irradiated at laser fluence 1.52 J/cm 2 for 30 min (BP_30), 1 h (BP_1) or 3 h (BP_3). Immediately after laser treatment, specimens were re-immersed in 1.5 × SBF and incubated at 60 °C for up to 7 days (d). Laser-untreated BP67 specimens incubated under the same conditions were used as control (BP_c). The solution was renewed after 6 h, 24 h and then every 2 days, and the solid to liquid ratio was preserved at 1 mg/1 ml. At the end of the incubation period, the samples were removed from the 1.5 × SBF solution, rinsed with acetone and distilled water and characterized by FTIR, XRD, SEM-EDS and micro-Raman techniques.
2.4
Surface characterization of the samples by means of different techniques
2.4.1
Fourier transformed infrared (FTIR) analysis
FTIR reflectance spectra were obtained by a FTIR Spectrometer (IFS113v, Bruker) in the MIR (4000–400 cm −1 ) region with a number of 64 scans per spectrum and a resolution 2 cm −1 .
2.4.2
X-ray diffraction (XRD) analysis
The XRD analysis was performed using a Philips PW1710 diffractometer with Ni-filtered CuKα radiation on the surface of the studied samples. The counting statistics were: start angle 5°, end angle 75° (2 θ ), step size 0.05° (2 θ ), time per step 1 s and scan speed 0.01°2 θ /s. The ICDD PDF-4+ (2009) database was used for the identification of the phases contained in the studied samples.
2.4.3
Micro Raman analysis
Raman measurements were conducted with a LabRAM HR (Horiba) or a T64000 (Horiba) spectrometer equipped with a Peltier and a liquid nitrogen cooled charge coupled device, respectively. Excitation was provided by a Cobolt Fandango™ diode pumped solid state laser at 515 nm with a power of ∼9 mW focused on the sample by a standard 10× objective at a spot diameter of ∼2.5 μm. Presented spectra were collected from an area of 50 × 50 μm 2 , taking advantage of the DuoScan™ scanner. It allows fast scanning of the laser beam on the sample, resulting in more representative spectra and lower effective average power.
2.4.4
Scanning electron microcopy and energy dispersive X-ray microanalysis (SEM-EDS)
For topographical evaluation and analysis of surface elemental composition the specimens were carbon coated with an average thickness of 200 Å, using a vacuum evaporator JEOL-4X and were observed using a Scanning Electron Microscope (JEOL J.S.M. 840A, Tokyo, Japan) with associated EDS (Oxford INKA-300). All analyses were done with a 20 kV accelerating voltage and 0.4 mA probe. The images were taken in magnification ×1000. The cross-sections were prepared by embedding the specimens in epoxy resin (Epofix resin, Struers) under vacuum, followed by cutting using a water-cooled, diamond rotary saw. Sections were ground by hand using 400, 600, 800 and 1000 grit silicon carbide and polished in turn with diamond paste with a grain diameter of 6, 3 and 1 μm.
2.5
Investigation of changes in chemical composition of the 1.5 × SBF before and after laser irradiation
In order to evaluate changes in chemical composition of the 1.5 × SBF solution before and after laser irradiation for 3 h, Si, P, Al and Ca concentrations were analyzed in the same reacted solutions by Inductively Coupled Plasma Atomic Emission Spectrometry (ICP-AES), using a PerkinElmer 3100XL Axial Viewing Spectrometer, equipped with a cyclonic spray chamber. The sample introduction flow rate was 2 ml/min. Detection limits were as follows: Si 4+ (0.05 ppm), Al 3+ (0.01 ppm), Ca 2+ (0.005 ppm) and for P (0.03 ppm). Potential temperature changes of the 1.5 × SBF solution were monitored after 30 min, 1 h and 3 h of LAB process by a digital platinum resistance thermometer (Guildline 9540/B), while the pH of the 1.5 × SBF solution was measured before and after 3 h of laser irradiation (B&C PH125,2 pH/ORP meter), as well as during the process of liquid renewal.
2.6
Establishment of primary cell cultures
Human primary gingival fibroblast (hGF) and human primary periodontal ligament fibroblast (PDLF) cell cultures were established from free gingiva and periodontal ligament biopsies respectively of young healthy donors after routine third molar extraction. The research protocol was approved by the Ethical committee of the Institutional review board and all patients signed an informed consent form. The gingival tissue biopsies were minced into 1–2 mm fragments and placed in 25 cm 2 culture flasks with Dulbecco modified Eagle medium (DMEM) supplemented with 10% Fetal Bovine Serum (FBS) and antibiotics/antimycotics (100 units/ml penicillin, 100 mg/ml streptomycin and 0.25 mg/ml Amphotericin B). (all from Invitrogen, Karlsruhe, Germany) The flasks were incubated at 37 °C in humidified atmosphere of 5% CO 2 . After reaching confluency, the cells were transferred to a larger 75 cm 2 flask (passage 1), and then continuously subcultured for further experiments. Cells from passages 3–6 were used for all experiments.
For the establishment of PDLF cultures the teeth were washed with sterile PBS and disinfected with sodium hypochlorite and then placed into a falcon tube containing 0.25% trypsin/1 mM Ethylene Diamine Tetraacetic Acid-EDTA solution and kept in water bath at 37 °C for 45 min. Subsequently, complete culture medium (DMEM with 10% FBS and antibiotics/antimycotics) was added into the falcon tube to stop the trypsin action. The culture medium containing cells was transferred into a new centrifuge tube and centrifuged for 10 min at 115 × g . The supernatant was discarded and the cells were re-suspended in fresh medium and pipetted into 25 cm 2 culture flasks. The flasks were incubated at 37 °C in humidified atmosphere of 5% CO 2 . After reaching confluency, the cells were transferred to a 75 cm 2 flask (passage 1), and then continuously subcultured for further experiments. Cells from passages 2–4 were used for all experiments.
SAOS-2 osteoblast-like cell line was obtained from the American Type Culture Collection (ATCC No. HTB 85). Cells were cultured at 37 °C in humidified atmosphere of 5% CO 2 in a-Modification of Eagle’s Medium (aMEM) (Invitrogen), supplemented with 10% FBS and antibiotics/antimycotics, as described above.
2.7
Evaluation of viability by the MTT assay
For the evaluation of viability of cells seeded in direct contact with the experimental materials, the MTT [3-(4,5-dimethylthiazol-2-yl)-2,5-diphenyltetrazolium bromide] assay was performed. Three groups of ceramic specimens (6 × 1.5 mm), prepared as described above were tested: (1) feldspathic dental ceramic (P), (2) bioactive glass ceramic composite (BP) and (3) laser-treated BP specimens coated with the developed HA layer (BP/HA specimens) (laser irradiated for 3 h and subsequent aging in 1.5 × SBF solution at 60 °C for 7 days). Prior to cell culture, sintered specimens were sterilized in an autoclave for 20 min at 2 bar pressure and then placed in each well of a 48-well plate (Corning, Cambridge, MA) and conditioned in 0.5 mL of culture medium for 24 h at 37 °C in an incubator. Cells (HGFs, PDLFs or SAOS-2) were seeded at a density of 2.5 × 10 4 for HGFs and PDLFs and 5 × 10 4 for SAOS-2 on the top of the experimental specimens. Cells grown on tissue culture plastic (C) were simultaneously evaluated as control.
After 24, 48 or 72 h, MTT (Sigma Aldrich, Taufkirchen, Germany) at final concentration of 0.5 mg/ml was added in each well. Afterwards, the plates were incubated at 37 °C for an additional 3 h. The purple formazan crystals that were formed were diluted by dimethyl-sulfoxide (DMSO) (Sigma Aldrich) for 1.5 h at 37 °C . The absorbance was measured against blank (DMSO) at a wavelength of 545 nm and a reference filter of 630 nm by a microplate reader (Epock, Biotek, Biotek instruments, Inc., Vermont, U.S.A). Experiments were performed in four replicates and repeated three times. The results were expressed as the averaged absorbance as % percentage of the control value.
2.8
SEM evaluation of the cell morphology
After 72 h of cell culturing, the specimens were washed twice with PBS, fixed in 3% glutaraldehyde in 0.1 M sodium cacodylate buffer containing 0,1 M sucrose (pH 7.4), dehydrated in a graded series of alcohols (35%, 50%, 70%, 95%, and two changes of 100% ethanol) and air-dried in a hood for 20 min. Finally, the specimens were carbon coated and observed by SEM (JEOL J.S.M. 840A) in magnification ×1000.
2.9
Statistical analysis
Statistical analysis of the cell viability data was performed using two-way ANOVA analysis. Follow up comparisons between groups were carried out using Tukey’s post-hoc test. Analysis was performed with Prism 6.0 Software (GraphPad, CA, USA) and the level of statistical difference was 0.05 ( p < 0.05).
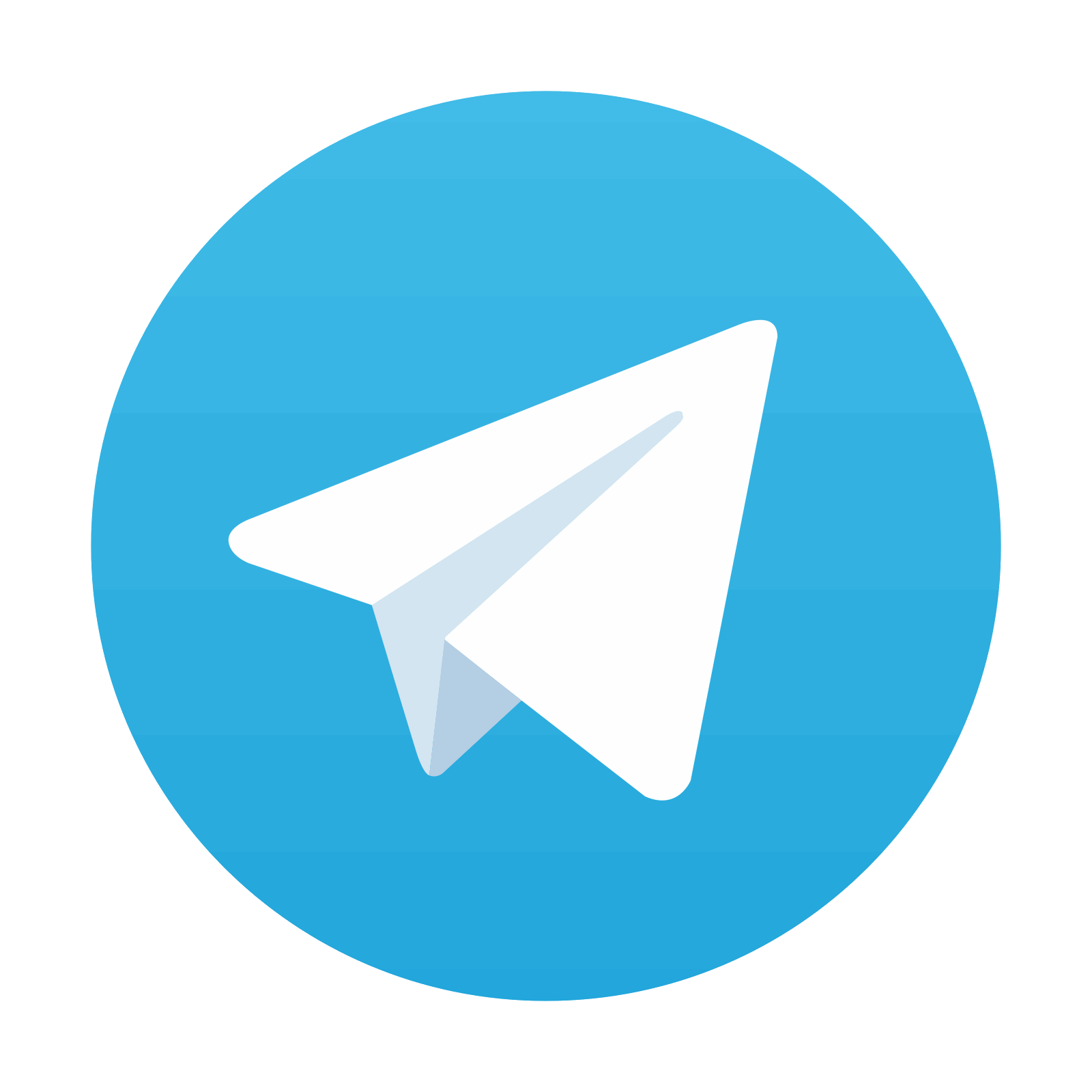
Stay updated, free dental videos. Join our Telegram channel

VIDEdental - Online dental courses
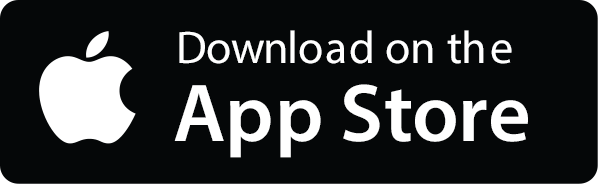
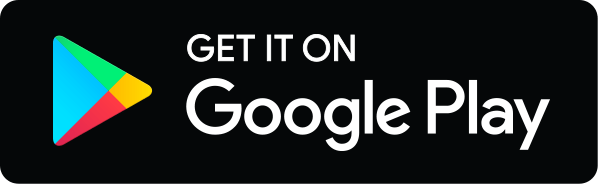