Abstract
Objective
Selective laser melting (SLM) is increasingly used for the fabrication of customized dental components made of metal alloys such as CoCrMo. The main aim of the present study is to elucidate the influence of the non-equilibrium microstructure obtained by SLM on corrosion susceptibility and extent of metal release (measure of biocompatibility).
Methods
A multi-analytical approach has been employed by combining microscopic and bulk compositional tools with electrochemical techniques and chemical analyses of metals in biologically relevant fluids for three differently SLM fabricated CoCrMo alloys and one cast CoCrMo alloy used for comparison.
Results
Rapid cooling and strong temperature gradients during laser melting resulted in the formation of a fine cellular structure with cell boundaries enriched in Mo (Co depleted), and suppression of carbide precipitation and formation of a martensitic ɛ (hcp) phase at the surface. These features were shown to decrease the corrosion and metal release susceptibility of the SLM alloys compared with the cast alloy. Unique textures formed in the pattern of the melting pools of the three different laser melted CoCrMo alloys predominantly explain observed small, though significant, differences. The susceptibility for corrosion and metal release increased with an increased number (area) of laser melt pool boundaries.
Significance
This study shows that integrative and interdisciplinary studies of microstructural characteristics, corrosion, and metal release are essential to assess and consider during the design and fabrication of CoCrMo dental components of optimal biocompatibility. The reason is that the extent of metal release from CoCrMo is dependent on fabrication procedures.
1
Introduction
CrCoMo alloys are nowadays commonly used for dental restorations such as customized abutments, crown and bridges in the anterior and posterior region, telescope and conical crowns, and screw-retained restorations . The use of these alloys was for a long time suppressed due to rapidly emerging full ceramic restoration concepts adopted for esthetic reasons and concern toward biocompatibility and metal release aspects.
The function and long life expectancy of dental restorations are of high priority. From this follows high demands on their mechanical properties, corrosion resistance and biocompatibility. These multiple requirements have in some cases led to failure of dental restorations, simply because recognized knowledge has been disregarded. For example, galvanic corrosion effects when coupling different metals have seriously been overlooked when Ti- and Co-alloys have been coated with gold in implants with serious consequences for the patients . The fact that the properties of a given alloy depends on the manufacturing process, surface treatments, bulk composition, etc. have recently been highlighted in the dentistry restoration community, and the importance of corrosion and metal release aspects when selecting materials for dental restoration recognized .
Recent rapid development and industrialization of selective laser sintering/melting techniques have enabled mass production of e.g. CoCrMo alloys in a cost effective manner and the fabrication of customized restorations with reliable mechanical properties and of increased geometric accuracy. Selective laser melting (SLM) belongs to a family of technologies known as additive manufacturing . SLM uses a high-energy laser beam to fuse metal/alloy powder particles directly into complex net-shaped 3D components in a layer-by-layer manner. A laser beam is repeatedly scanned over a single layer of powder particles (e.g., CoCrMo), which are fully or partly consolidated via melting . The process is accomplished using computer aided design (CAD) and manufacture (CAM). The SLM technique (sometimes referred to as laser sintering) is widely used for fabrication of medical and dental implants because of the need for complex geometries, small volumes and individualized products .
CoCrMo alloys are nowadays considered as highly corrosion resistant and biocompatible materials in dentistry . So far, primarily mechanical properties and microstructural aspects have been investigated . Metal release (biocompatibility) aspects have been less considered or addressed .
The layer-by-layer fabrication principle of the SLM technique allows for the use of different processing parameters within different sections of the prosthesis. These parameters may however influence the overall mechanical and biocompatibility properties of the product, aspects so far relatively unexplored. This study therefore aims to elucidate differences in microstructure, corrosion resistance and extent of metal release for CoCrMo alloys fabricated by means of SLM using three different sets of laser scanning parameters, in comparison with a cast CoCrMo alloy.
2
Materials and methods
2.1
Alloys and selective laser melting (SLM)
Three different SLM processing strategies were employed to fabricate fully dense (a density of approx. 8.3 g cm −3 ) CoCrMo alloys (1 cm × 1 cm × 0.1 cm) from a commercial alloy powder (CobaltChrome MP1™, EOS GmbH, Germany). The powder size was 25 μm (5–40 μm) based on scanning electron microscopy measurements. The nominal bulk alloy composition of the powder is given in Table 1 . Based on supplier information, this powder is recommended for dental applications and has a reported Young’s modulus of 200–210 GPa, a tensile strength of 1150–1400 MPa, and a yield strength of 880–980 MPa when standard SLM strategies for fully dense materials are employed .
Co | Cr | Mo | Si | Mn | Fe | C | Ni | |
---|---|---|---|---|---|---|---|---|
CobaltChrome MP1 | 60–65 | 26–30 | 5–7 | ≤1.0 | ≤1.0 | ≤0.75 | ≤0.16 | ≤0.10 |
Cast CoCrMo | 65.3 | 28.3 | 5.4 | N/A | 0.4 | 0.2 | 0.2 | 0.2 |
Three SLM processing strategies were applied using an EOS M270 apparatus: (i) L20, a layer thickness of 0.02 mm, scanning parallel traces in each layer; (ii) R20, layer thickness of 0.02 mm, scanning traces rotated at an angle of 60° every second layer, and (iii) R40, layer thickness of 0.04 mm, scanning traces rotated at an angle of 60° every second layer, allowing a fast formation speed and yielding a increased surface roughness compared with R20 and L20. R20 is the strategy for an optimal surface, and R40 for an optimal and rapid manufacture of a fully dense alloy. Commercially available SLM manufactured dental alloys differ in laser strategies due to business secrets. Detailed SLM process parameters for this study are given in Table 2 .
Sample denotation | Angle (°) | Laser power (W) | Laser scan speed (mm s −1 ) | Scan spacing (mm) | Powder stacking thickness (μm) |
---|---|---|---|---|---|
R40 | 60 | 195 | 535 | 0.14 | 40 |
R20 | 60 | 195 | 800 | 0.1 | 20 |
L20 | 0 | 195 | 800 | 0.1 | 20 |
A cast biomedical grade high carbon CoCrMo alloy (ASTM F75 ) intended for orthopedic implants, denoted cast (Ø 14 mm, thickness 2 mm) was investigated for comparative reasons. Its nominal bulk composition is given in Table 1 , and its corrosion properties and microstructure described elsewhere . After casting, the alloy was processed by hot isostatic pressing (1200 °C, 4 h, 103 MPa, slow cooling) and subsequently heat-treated (1200 °C, 4 h, fast cooled to below 800 °C). The presence of M 23 C 6 (M = metal) carbides in the grain boundaries of the cast CoCrMo alloy and microstructural features of main importance for corrosion and dissolution are reported elsewhere .
2.2
Microstructural investigation
The microstructure was investigated on a macro-scale using optical microscopy (Olympus SZX12; reflective mode), and on a micro-scale by means of scanning electron microscopy (SEM, JEOL JSM-7000F). The SLM fabricated alloys were mechanically polished and further chemically etched (immersion etching) in 36% HCl (in water) for 8 h prior to the SEM investigation. Specimens for TEM characterization were prepared by either electrolytic polishing at room temperature using a TenuPol-5 electrolytic polishing system (90% acetic acid and 10% perchloric acid; 25 V), or by argon ion beam milling with an accelerating voltage of 6 keV. Transmission electron microscopy (TEM, JEOL FEG-2100F) with energy dispersive spectroscopy (EDS) was employed to investigate differences in composition with high lateral resolution (<1 μm) for the alloy R20. X ray powder diffraction (Panalytical X’Pert PRO) in reflection mode was used to obtain crystallographic phase information. Diffractograms were recorded from 20° to 120° (2 θ ) using monochromatic Cu Kα ( λ = 1.5405981 Å) radiation (40 mA, 45 kV) and step sizes of 0.013° (polished SLM samples) and 0.026° (unpolished SLM samples), respectively.
2.3
Electrochemical properties and surface analysis
Corrosion susceptibility was investigated for all samples by employing electrochemical potentiodynamic polarization using a microcapillary set-up (glass capillary, Ø 159 μm, filled with 1 M NaCl). The advantage of micro-electrochemistry compared with conventional macroscopic electrochemistry is that micron-sized inhomogeneities within the material can be detected. Measurements were performed on at least three different surface areas for each material. The microcell is described in detail elsewhere . In short, the auxiliary electrode (platinum wire) is located inside the glass capillary with a reference electrode (Ag/AgCl (3 M KCl)) connected via an electrolytic bridge. The tip of the glass capillary is polished and a silicone rubber O-ring is used to seal between the capillary and the sample surface. A high resolution potentiostat (Jaissle IMP 83 PC T-BC) with a current detection limit of 10 fA was used with a sampling rate of 9 Hz. The capillary was positioned in an objective holder to enable the microscopic investigation (Leica DM LS2, lateral resolution approximately 1 μm) of the same area. One day prior to the measurements the samples were polished to 1 μm using diamond paste, ultrasonically cleaned in ethanol for 5 min, dried in air using a fan and stored in air at ambient conditions. This pre-treatment and aging process were made for all samples in the same way to allow an equal growth of the native surface oxide. The start potential was set to −600 mV (Ag/AgCl), and the samples were anodically polarized at 1 mV s −1 to 1000 mV, followed by cathodic polarization back to −600 mV. Before applying the potential, the system was allowed to equilibrate for 10 ± 5 min. After polarization, compositional surface analysis (Co, Cr, O, Fe, C, Na, Cl (Mo not included)) was made on a corroded area (250 μm × 200 μm) of one of the samples, the L20 alloy, using scanning Auger electron microscopy (SAM, Physical Electronics (PHI) 670, 10 kV). The main aim of this investigation was to identify enriched or depleted elements as a result of corrosion.
2.4
Metal release (biocompatibility) investigations
Prior to exposure, the samples were ground using 1200 grit SiC, ultrasonically cleaned in acetone and isopropyl alcohol for 7 min, respectively, dried with cold nitrogen gas, and aged (desiccator, room temperature) for 24 ± 1 h to achieve a defined and stable surface oxide, as described elsewhere . The samples were entirely immersed in a vertical position (a surface area to solution volume ratio of 1 cm 2 mL −1 ) in phosphate buffered saline (PBS, pH 7.3 ± 0.1), or PBS + 10 g L −1 bovine serum albumin (PBS + BSA, pH 7.3 ± 0.1), in closed acid-cleaned polyethylene vessels for 2 and 168 h (1 week), respectively. The vessels were positioned in a Stuart platform-rocker incubator with bilinear shaking (25 cycles min −1 ) at 37 ± 0.5 °C. Triplicate samples and one blank sample (without alloy sample) were exposed in parallel for each time period and fluid. The chemical composition of PBS was 8.77 g L −1 NaCl, 1.28 g L −1 Na 2 HPO 4 , 1.36 g L −1 KH 2 PO 4 , 370 μL L −1 50% NaOH and ultrapure water (18.2 MΩ cm). PBS of slightly varying compositions is widely used in the scientific literature as a physiological reference solution . The fluids were justified from their relevance for human blood, and previous deliberations and findings by some of the authors on protein adsorption and protein-induced metal release on chromium metal, stainless steel, and cast CoCrMo . After exposure, the solution was separated from the samples, and acidified (65 vol.% ultrapure nitric acid) to a pH < 2. All chemicals were of at least analytical grade (Sigma–Aldrich), and all equipment and vessels were acid-cleaned by 10 vol.% nitric acid for 24 h before rinsed four times with ultrapure water. Solution metal analysis (total metal) was performed using atomic absorption spectroscopy (AAnalyst 800, Perkin Elmer) with flame (for Co) and graphite furnace (for Co, Cr and Mo) modes. Calibration curves were based on at least four calibration standards and quality control samples of known concentration were analyzed regularly. Limits of detection (LOD, 3 times the highest standard deviation of blank samples) were 1 μg L −1 , for Co, Cr, and Mo, respectively, in the graphite mode. Measured metal concentrations were significantly higher than their corresponding limits of detection for all time periods, exposure conditions and analyzed metals.
2.5
Statistical significance test method
A Student t -test of unpaired data with unequal variance was employed between two different data sets to identify the significance of observed differences. Differences are counted as significant when p < 0.05, with higher significance for a smaller p -value.
2
Materials and methods
2.1
Alloys and selective laser melting (SLM)
Three different SLM processing strategies were employed to fabricate fully dense (a density of approx. 8.3 g cm −3 ) CoCrMo alloys (1 cm × 1 cm × 0.1 cm) from a commercial alloy powder (CobaltChrome MP1™, EOS GmbH, Germany). The powder size was 25 μm (5–40 μm) based on scanning electron microscopy measurements. The nominal bulk alloy composition of the powder is given in Table 1 . Based on supplier information, this powder is recommended for dental applications and has a reported Young’s modulus of 200–210 GPa, a tensile strength of 1150–1400 MPa, and a yield strength of 880–980 MPa when standard SLM strategies for fully dense materials are employed .
Co | Cr | Mo | Si | Mn | Fe | C | Ni | |
---|---|---|---|---|---|---|---|---|
CobaltChrome MP1 | 60–65 | 26–30 | 5–7 | ≤1.0 | ≤1.0 | ≤0.75 | ≤0.16 | ≤0.10 |
Cast CoCrMo | 65.3 | 28.3 | 5.4 | N/A | 0.4 | 0.2 | 0.2 | 0.2 |
Three SLM processing strategies were applied using an EOS M270 apparatus: (i) L20, a layer thickness of 0.02 mm, scanning parallel traces in each layer; (ii) R20, layer thickness of 0.02 mm, scanning traces rotated at an angle of 60° every second layer, and (iii) R40, layer thickness of 0.04 mm, scanning traces rotated at an angle of 60° every second layer, allowing a fast formation speed and yielding a increased surface roughness compared with R20 and L20. R20 is the strategy for an optimal surface, and R40 for an optimal and rapid manufacture of a fully dense alloy. Commercially available SLM manufactured dental alloys differ in laser strategies due to business secrets. Detailed SLM process parameters for this study are given in Table 2 .
Sample denotation | Angle (°) | Laser power (W) | Laser scan speed (mm s −1 ) | Scan spacing (mm) | Powder stacking thickness (μm) |
---|---|---|---|---|---|
R40 | 60 | 195 | 535 | 0.14 | 40 |
R20 | 60 | 195 | 800 | 0.1 | 20 |
L20 | 0 | 195 | 800 | 0.1 | 20 |
A cast biomedical grade high carbon CoCrMo alloy (ASTM F75 ) intended for orthopedic implants, denoted cast (Ø 14 mm, thickness 2 mm) was investigated for comparative reasons. Its nominal bulk composition is given in Table 1 , and its corrosion properties and microstructure described elsewhere . After casting, the alloy was processed by hot isostatic pressing (1200 °C, 4 h, 103 MPa, slow cooling) and subsequently heat-treated (1200 °C, 4 h, fast cooled to below 800 °C). The presence of M 23 C 6 (M = metal) carbides in the grain boundaries of the cast CoCrMo alloy and microstructural features of main importance for corrosion and dissolution are reported elsewhere .
2.2
Microstructural investigation
The microstructure was investigated on a macro-scale using optical microscopy (Olympus SZX12; reflective mode), and on a micro-scale by means of scanning electron microscopy (SEM, JEOL JSM-7000F). The SLM fabricated alloys were mechanically polished and further chemically etched (immersion etching) in 36% HCl (in water) for 8 h prior to the SEM investigation. Specimens for TEM characterization were prepared by either electrolytic polishing at room temperature using a TenuPol-5 electrolytic polishing system (90% acetic acid and 10% perchloric acid; 25 V), or by argon ion beam milling with an accelerating voltage of 6 keV. Transmission electron microscopy (TEM, JEOL FEG-2100F) with energy dispersive spectroscopy (EDS) was employed to investigate differences in composition with high lateral resolution (<1 μm) for the alloy R20. X ray powder diffraction (Panalytical X’Pert PRO) in reflection mode was used to obtain crystallographic phase information. Diffractograms were recorded from 20° to 120° (2 θ ) using monochromatic Cu Kα ( λ = 1.5405981 Å) radiation (40 mA, 45 kV) and step sizes of 0.013° (polished SLM samples) and 0.026° (unpolished SLM samples), respectively.
2.3
Electrochemical properties and surface analysis
Corrosion susceptibility was investigated for all samples by employing electrochemical potentiodynamic polarization using a microcapillary set-up (glass capillary, Ø 159 μm, filled with 1 M NaCl). The advantage of micro-electrochemistry compared with conventional macroscopic electrochemistry is that micron-sized inhomogeneities within the material can be detected. Measurements were performed on at least three different surface areas for each material. The microcell is described in detail elsewhere . In short, the auxiliary electrode (platinum wire) is located inside the glass capillary with a reference electrode (Ag/AgCl (3 M KCl)) connected via an electrolytic bridge. The tip of the glass capillary is polished and a silicone rubber O-ring is used to seal between the capillary and the sample surface. A high resolution potentiostat (Jaissle IMP 83 PC T-BC) with a current detection limit of 10 fA was used with a sampling rate of 9 Hz. The capillary was positioned in an objective holder to enable the microscopic investigation (Leica DM LS2, lateral resolution approximately 1 μm) of the same area. One day prior to the measurements the samples were polished to 1 μm using diamond paste, ultrasonically cleaned in ethanol for 5 min, dried in air using a fan and stored in air at ambient conditions. This pre-treatment and aging process were made for all samples in the same way to allow an equal growth of the native surface oxide. The start potential was set to −600 mV (Ag/AgCl), and the samples were anodically polarized at 1 mV s −1 to 1000 mV, followed by cathodic polarization back to −600 mV. Before applying the potential, the system was allowed to equilibrate for 10 ± 5 min. After polarization, compositional surface analysis (Co, Cr, O, Fe, C, Na, Cl (Mo not included)) was made on a corroded area (250 μm × 200 μm) of one of the samples, the L20 alloy, using scanning Auger electron microscopy (SAM, Physical Electronics (PHI) 670, 10 kV). The main aim of this investigation was to identify enriched or depleted elements as a result of corrosion.
2.4
Metal release (biocompatibility) investigations
Prior to exposure, the samples were ground using 1200 grit SiC, ultrasonically cleaned in acetone and isopropyl alcohol for 7 min, respectively, dried with cold nitrogen gas, and aged (desiccator, room temperature) for 24 ± 1 h to achieve a defined and stable surface oxide, as described elsewhere . The samples were entirely immersed in a vertical position (a surface area to solution volume ratio of 1 cm 2 mL −1 ) in phosphate buffered saline (PBS, pH 7.3 ± 0.1), or PBS + 10 g L −1 bovine serum albumin (PBS + BSA, pH 7.3 ± 0.1), in closed acid-cleaned polyethylene vessels for 2 and 168 h (1 week), respectively. The vessels were positioned in a Stuart platform-rocker incubator with bilinear shaking (25 cycles min −1 ) at 37 ± 0.5 °C. Triplicate samples and one blank sample (without alloy sample) were exposed in parallel for each time period and fluid. The chemical composition of PBS was 8.77 g L −1 NaCl, 1.28 g L −1 Na 2 HPO 4 , 1.36 g L −1 KH 2 PO 4 , 370 μL L −1 50% NaOH and ultrapure water (18.2 MΩ cm). PBS of slightly varying compositions is widely used in the scientific literature as a physiological reference solution . The fluids were justified from their relevance for human blood, and previous deliberations and findings by some of the authors on protein adsorption and protein-induced metal release on chromium metal, stainless steel, and cast CoCrMo . After exposure, the solution was separated from the samples, and acidified (65 vol.% ultrapure nitric acid) to a pH < 2. All chemicals were of at least analytical grade (Sigma–Aldrich), and all equipment and vessels were acid-cleaned by 10 vol.% nitric acid for 24 h before rinsed four times with ultrapure water. Solution metal analysis (total metal) was performed using atomic absorption spectroscopy (AAnalyst 800, Perkin Elmer) with flame (for Co) and graphite furnace (for Co, Cr and Mo) modes. Calibration curves were based on at least four calibration standards and quality control samples of known concentration were analyzed regularly. Limits of detection (LOD, 3 times the highest standard deviation of blank samples) were 1 μg L −1 , for Co, Cr, and Mo, respectively, in the graphite mode. Measured metal concentrations were significantly higher than their corresponding limits of detection for all time periods, exposure conditions and analyzed metals.
2.5
Statistical significance test method
A Student t -test of unpaired data with unequal variance was employed between two different data sets to identify the significance of observed differences. Differences are counted as significant when p < 0.05, with higher significance for a smaller p -value.
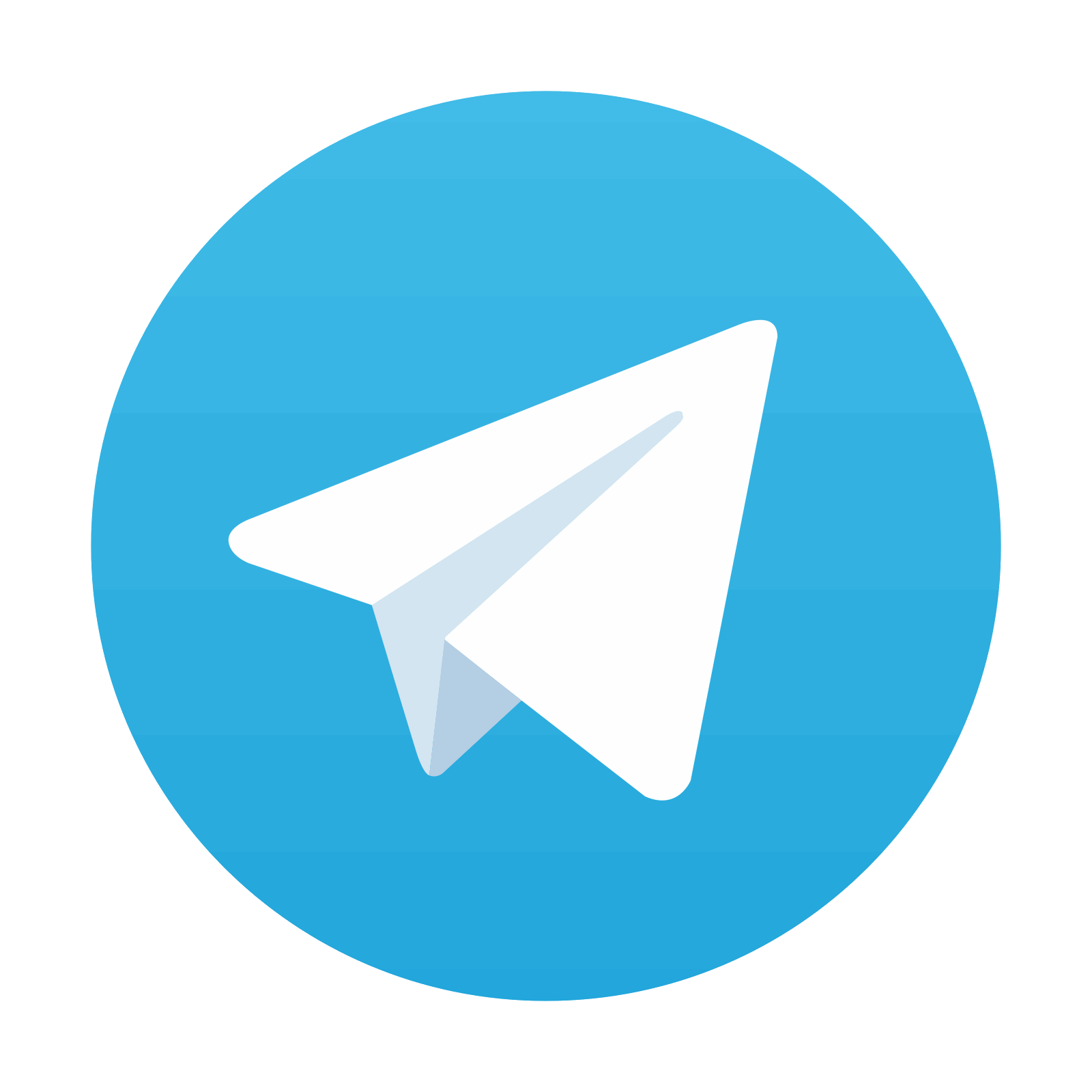
Stay updated, free dental videos. Join our Telegram channel

VIDEdental - Online dental courses
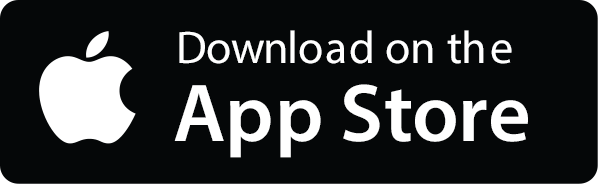
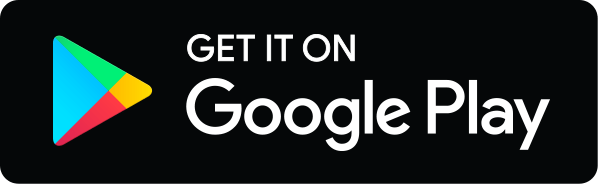