div epub:type=”chapter” role=”doc-chapter”>
K. Orhan (ed.)Ultrasonography in Dentomaxillofacial Diagnosticshttps://doi.org/10.1007/978-3-030-62179-7_21
21. USG Imaging in Physiotherapy of Dentomaxillofacial Region
ElastographySonoelastographyShear-wave elastographyMusclePhysiotherapy
21.1 Introduction
Most internal derangements can be resolved with conservative treatment, and physiotherapy is increasingly being recognized as an important part of this treatment. With this feature, physiotherapy is a wide-ranging profession with opportunities to work in many different areas. The interrelationship between dentistry and physiotherapy helps in early diagnosis and improves the therapeutic interventions’ effectiveness especially in temporomandibular disorders (TMDs), sleep bruxism, and dentomaxillofacial region postural problems.
The diagnosis in these pathologies generally depends on a subjective assessment by patients and clinicians; therefore, there is a need for objective assessment for effective treatment. Soft tissues in the body have high water content and are virtually incompressible thus sophisticated equipment is necessary to detect small tissue changes. Elastography-based imaging techniques have received great interest in recent years for noninvasive assessment of musculoskeletal soft tissue mechanical properties. These techniques take advantage of changing soft tissue elasticity in different pathologies to provide qualitative and quantitative information that can be used for diagnostic purposes and follow-up evaluations during rehabilitation [1]. There are currently two main elastography methods in general clinical usage, namely compression/strain elastography and shear-wave elastography [2].
21.2 Role of Physiotherapy (Applications) in Dentomaxillofacial Region Problems
Temporomandibular disorders (TMD) are a complicated and heterogeneous group of pathologies affecting the temporomandibular joint (TMJ) and muscles of mastication, resulting in pain and disability [3]. Epidemiological studies of TMD report a prevalence of 82% in the general population with 48% of them presenting muscle tenderness and difficulty in mouth opening. TMD are thought to be the most common orofacial pain conditions of non-dental origin [4]. In connection with this problem, another pathology seen in 8–20% of the population is Sleep Bruxism (SB) [5]. Bruxism is a common clinical presence defined as “a repetitive jaw muscle activity characterized by clenching or grinding of the teeth and/or by bracing or thrusting of the mandible.” [6].
Studies claim that 85–90% of the general population experience episodes of bruxism during their lives. Therefore, it is important to find an effective treatment for these dentomaxillofacial region pathologies [5]. Because of the symptoms as muscle pain, muscle activity alterations, limitation of mouth opening, anxiety, stress, depression, poor sleep, and oral health quality in TMD and Bruxism, treatment using an interdisciplinary professional team including dentists and physiotherapists is important [5]. Physiotherapy is the preferred conservative approach for treating TMD and Bruxism, as it facilitates multimodal treatment that addresses patient-specific impairments [7].
Recently, actual treatment procedures include the framework of a conservative “multiple-P” approach (i.e., physiotherapy, plates, pep talk, pills, psychology) [5]. Applications of oral orthoses and physiotherapy modalities (such as exercises, thermal agents, or massage) are usually performed prior to medical drug regimens and invasive procedures. Among these methods, the most commonly used one is the occlusal splint (OS) application especially for Sleep Bruxism treatment. However, OS may not be effective in some patients and has limited use for patients who have elevated gag reflex or higher risk of airway obstruction (due to epilepsy, etc.) and who use dental brackets. Thus, there is a need for an alternative treatment method that can replace OS [8].
Treatment methods used in physiotherapy include electrotherapy, therapeutic exercises, muscle relaxation techniques, postural awareness, acupuncture, manual therapy, and cognitive behavioral therapy techniques. However, the effectiveness of all these approaches on the signs and symptoms of TMD and Bruxism is still questionable and continues to be studied [5].
In the literature, there are lots of studies investigating the effectiveness of physiotherapy modalities in patients with bruxism and TMDs. The majority of them investigated the effectiveness of electrotherapy techniques, one of the most used ones is Transcutaneous Electrical Nerve Stimulation (TENS). The results of studies especially showed that electrotherapeutic resources decrease masseter muscle activity and pain and improve oral health in individuals with Bruxism and TMD especially in short term [5, 7, 9, 10]. Massage therapy is another most used treatment approach. In studies, occlusal splint was generally compared with massage therapy. As a general result of studies, it was concluded that massage therapy and the use of an occlusal splint did not lead to changes in the activity of the masseter and anterior temporal muscles. However, the combination of therapies let a reduction in the intensity of the signs and the symptoms of TMD and Bruxism [11–14]. In addition, although there is limited evidence, soft tissue release and strengthening exercises for the muscles of mastication in patients with TMD, provide a reduction in pain and disability. Also, joint mobilization and manipulation to the temporomandibular joint and/or upper cervical articulations, and dry needling or acupuncture to the lateral pterygoid muscle and posterior periarticular connective tissue are considered as the most evidence-based approaches for conservatively treating TMD [7]. Apart from all of these modalities, new different techniques are also being sought. One of the new approaches for bruxism is Kinesio taping (KT) method. Keskinguzar et al.’s study demonstrated that 5 weeks of treatment with occlusal splint and KT showed similar efficacy for sleep bruxism therapy. They also concluded that KT can be used as a routine technique in patients with Sleep Bruxism as an alternative to occlusal splint especially in patients with nausea, with risk of swallowing or aspiration of the splints (such as an epileptic crisis), or with braces in the teeth for orthodontic treatment [8].
In conclusion, it can be said that physiotherapy interventions are effective for the treatment of bruxism and TMD. Most of the studies have poor methodological quality and very low-quality evidence [5]. Therefore, there is still a need for well-designed randomized controlled trials examining physiotherapy interventions for Bruxism and TMDs.
21.3 USG Imaging Usage in Musculoskeletal System Structures
Chronic or acute musculoskeletal system pathologic conditions are common in the population and generally painful and debilitating. Physiotherapy applications to these pathologies affect the injured area which is generally musculoskeletal soft tissue including muscle, tendon, ligament, and nerve. Mechanical function or physical properties of the injured area may also be affected through these physiotherapy interventions which is difficult to assess particularly under in vivo conditions.
Increased collagen content and stiffness are seen in numerous musculoskeletal pathologies [15]. Ultrasound modalities (US) are being increasingly used not only in the diagnosis of musculoskeletal injuries in an attempt to localize involved structure as well as the extent and severity of the injury but also in the evaluation of intervention effectiveness. The main advantages of US include multiplanar imaging capability, quantification of soft tissue abnormalities, high resolution, direct correlation of clinical symptoms, and imaging findings and the unique possibility of dynamic imaging allows diagnoses that cannot be made with other modalities [16]. Ultrasound represents great utility in evaluating the underlying architecture of more superficial tissues, and a variety of techniques have been used to evaluate stiffness [17, 18], but, until recently, ultrasound has been purely qualitative [15].
Ultrasound elastography (EUS) is a recently developed ultrasound-based method, which allows both the qualitative visual and quantitative assessments of the mechanical properties of tissue [19–21]. The technique was first introduced in vitro in the early 1990s and subsequently evolved into a real-time tool for in vivo imaging of the distribution of tissue strain and elastic modulus [19]. EUS provides information on tissue stiffness, which complements and is independent of the acoustic impedance and vascular flow information provided by B-mode and Doppler imaging [19–21]. It has provided a quantifiable spatial representation of elasticity (or hardness or stiffness) in the form of an elastogram [22, 23].
The basic principle that EUS is based on is that stress applied to the tissue causes tissue differentiation. Preliminary data indicate that ultrasound elastography (EUS) may even be more sensitive than MRI or gray-scale ultrasound in detecting subclinical changes of muscle and tendon, and therefore could be valuable for early diagnosis and during the rehabilitation process. EUS could be used as a research tool to provide insight into the biomechanics and pathophysiology of musculotendinous diseases [19–21]. Over the years of research on elasticity, there have been several types of EUS, resulting in different methods, depending on the way of tissue stress application and the used method to state and set an image of tissue displacement [19]. EUS can be divided into strain elastography, which measures tissue strain, and shear-wave elastography (SWE), which measures the shear-wave speed. Shear-wave elastography can be further classified into transient elastography, point SWE, and multidimensional SWE (2-dimensional [2D] SWE and 3-dimensional SWE) [24].
21.3.1 Technical Considerations and Limitations of Ultrasound Elastography
21.3.1.1 Technical Considerations and Limitations of Sonoelastography in Muscle–Tendon Evaluation
Skeletal muscle is anisotropic, nonlinearly viscoelastic, deformable and active tissue [25] which consists of a very particular and well-described arrangement of muscle fibers. These muscle fibers are also associated with connective tissue network of collagen within the extracellular matrix (ECM) [26]. Elasticity/stiffness is thought as a critical determinant of muscle performance and force; therefore, its measurement in vivo can help to increase the understanding of muscle functions [16, 25]. As skeletal muscles are active tissues, an ideal technique would be capable of the real-time measurement to quantify tissue stiffness throughout functional range. Such a technique should be sensitive to detect small changes in stiffness and capable of determining material properties of relevant and often complex skeletal muscle architecture. Also, in tendon imaging, while using conventional ultrasonography differentiation of tendon alterations might be difficult since edema, hemorrhage, mucoid degeneration, or partial tears present as isoechoic alterations [27]. Therefore, reliable, noninvasive, quantitative techniques for evaluating musculoskeletal stiffness are necessary not only to advance our understanding of the mechanism and effects of altered musculoskeletal stiffness but also to improve diagnosis and treatment following injury [15].
During sonoelastography imaging, the normal relaxed muscle appears as a heterogeneous mosaic of intermediate or increased stiffness with scattered less stiff and stiffer areas, especially at the boundaries of the muscle [19]. Strain elastography has been used to assess many muscle pathologies, including muscular dystrophy, cerebral palsy, myositis, patellofemoral pain syndrome, intramuscular hemorrhage, fibrosis, etc. [19]. In addition, for dentomaxillofacial region, both strain and shear-wave elastography have been used to evaluate masseter muscle in patients with facial pain. In these studies, patients with facial pain and temporomandibular joint dysfunction have been shown to have a greater stiffness in masseter muscle compared with healthy controls. This correlates with the clinical finding of altered stiffness of masseter muscle in symptomatic patients with facial pain thus ultrasound elastography could become a useful technique in the assessment of these patients in both diagnosis and treatment effectiveness [2]. However, there is still no consensus in stiffness imaging differences between asymptomatic and symptomatic tendons. Although suggested potential role of sonoelastography for the assessment of a variety of traumatic and degenerative disorders of tendons, there is little evidence-based published data available for these assessments.
In sonoelastography, data acquisition and interpretation are largely operator dependent, which is the major limitation of this imaging system. While using sonoelastoraphy, a wide variety of techniques could be used to display elastographic images, and therefore the findings as well as the artifacts or limitations may be highly dependent on the technique. A major issue associated with sonoelastography is determining the correct amount of pressure to be applied on the tissue. The pressure should be moderate, described as the level of pressure that maintains contact with skin and for which the association between pressure and strain is proportional. Very high or low pressure should be avoided because the elastic properties of tissue become nonlinear. Fortunately, most sonoelastography systems now provide software, which gives feedback of the amount of pressure as a visual indicator/bar displayed on the screen alongside sonographic images. During evaluation, to minimize intra-observer variation and avoid transient temporal fluctuations, measurements in the elastograms should be based on examination of entire cine loops instead of single static images [19, 28].
Another important limitation in the use of sonoelastography is the size of the elastogram. The elastogram demonstrates the elasticity of each tissue relative to the remaining tissue within it. Therefore, the amount and level of stiffness of the surrounding tissue influence the appearance of the tissue of interest. This is not a major limitation in tissues such as the breast where the surrounding tissue is fairly homogeneous (fat and glandular tissue); however, in musculoskeletal sonoelastography, the elastogram may include tissues with wide stiffness differences (fat, tendon, bone, muscle), leading to a wider distribution in the acquired elasticity data [19]. In addition, if a skeletal muscle is evaluated with this type of elastography, the examination transducer should be perpendicular to the tissue to avoid anisotropy, as the B-mode ultrasound appearance influences the quality of the elastogram. Also, although tendon images could be taken in both transverse and longitudinal planes, longitudinal images represent better quality [19].
During measurement, another standardization problem is the distance between the probe and the tissue of interest. In many musculoskeletal applications, the tissue of interest is very superficial or even lies directly under the skin (e.g., Achilles tendon). In most ultrasound systems a minimum distance from the skin (usually 1.2 mm) is needed to place the box of the elastogram, so in thin people the use of gel pads or probe adaptors is necessary to increase the distance between the skin and probe [19]. On the contrary, the use of standoff devices for sonoelastography of the superficial structures does not influence the elastogram (a minimum necessary distance between transducer and lesion is 3 mm). However, in tendon evaluation, using too much gel within the region of interest should be avoided which may mask minimal differences [29].
In addition, there are also limitations and difficulties related to the anatomy of the area examined. Sonoelastography is especially problematic in cases of superficial protuberant masses and in areas with prominent adjacent bony structures [19, 28]. When a solid structure is delimited by an incompressible tissue, sonoelastography analysis of the internal structure is limited (the eggshell effect). Due to the tissue shifting, low stiffness lines may be seen at the interfaces between tissues, around calcifications, behind bone, or at the superficial edge of a homogeneous lesion. For instance, fluctuant changes at the borders of the Achilles tendon in an axial elastogram can be seen due to varying contact with the skin [29].
21.3.1.2 Technical Considerations and Limitations of Shear-Wave Elastography
-
(a)
Muscles
-
Although SWE provides reliable stiffness measurements under proper conditions [30], several technical parameters should be known that influence the measurements and need to be taken in account. Cortez et al. assessed intra- and inter-operator reproducibility of SWE for a specific site in normal skeletal muscles and concluded as fair to good ICC values (0.40–0.78) [47]. The wide range of these ICC values can be interpreted as there are too many factors affecting muscle stiffness measurements. Since no guideline on the use of SWE in medical practice exists yet, the anatomy of the studied muscle, the basic muscular biomechanical concepts (in particular the role of anisotropy), and the limits of the SWE method should be known before using SWE on skeletal muscle.
-
First, the major technical parameter that influences stiffness measurement is the anisotropic physical properties of the skeletal muscle. The anisotropic physical properties are responsible for the fact that shear waves travel faster along the direction of the fibers than they do when perpendicular to them. If technical challenges are understood, more effective algorithms could be developed to identify the anisotropic properties of muscles. For each technique, muscle and joint positioning, material setup, acoustic wave frequency, rheologic fit need to be clarified to harmonize measurements [25]. Stiffness measurements are sensitive to the angle between the probe axis and the orientation of the muscular fibers. Shear modulus measurements using SWE are correlated with Young’s modulus only if the probe is oriented parallel to the muscle fibers [25]; thus, it could be said that orientation of the ultrasound transducer plays a key role to obtain meaningful results. Genisson et al. found that shear waves propagate much more readily along muscle fibers longitudinally, as compared to perpendicularly or any interval of rotation therein. Same investigators reported that parallel transducer orientations obtained the most reliable measures of muscle elasticity [31]. Therefore, it can be said that SWE is partly operator-dependent especially in complex and large muscles. However, intra- and inter-observer reliabilities remain good to excellent if performed by a skilled senior and if the angle between the probe axis and the orientation of the muscular fibers is inferior to 20° [25, 32]. Another point that effect the reliability of measurements is the difficulty in assessing muscles with complex anatomy. Multipennate, conic, triangular, or fusiform anatomy causes a technical difficulty in visualizing the orientation of fibers. Therefore, this technical difficulty requires careful knowledge of muscle anatomy before using SWE. Because of the different pennation of the muscle fibers, during imaging, some elastography signal may be lost [33].
-
The second parameter concerns the viscoelastic properties of skeletal muscles. Muscle behaves as a combination of viscous and elastic properties, which together compose the shear modulus^ (G). Also, the viscoelastic properties are nonlinear, i.e., stiffness changes are relative to the characteristics of the applied stress such as the frequency of the shear wave. Moreover, given the contractile and stretching properties of muscle, muscle viscoelasticity is active.
-
Third, skeletal muscle is a deformable tissue; thus, SWE measurements are sensitive to transducer pressure. Therefore, the pressure applied to the tissue should be as low as possible. In a study, two different manually applied pressures were compared (1.5–3.0 kPa), and it was concluded that there was a significant difference in shear-wave speed values. Therefore, in the present conditions and system, an amount of couplant should be applied onto the tissue surface instead of applying the pressure manually through the probe. When the tested tissue depth is within 0.2 cm, it is not suitable to apply the couplant of more than 10 mm and also if the acquisition depth reaches 30 mm, the couplant should not be used with more than 20 mm [34]. To summarize, during SWE measurements, a generous amount of coupling gel needs to be applied onto the surface of the skin not to compress the muscles. When measuring superficial soft tissues, to give an average amount, approximately 1–5 mm of gel was generally used and thought enough for reliability. In addition, another important point in this regard is the most suitable measurement depth. When measuring the shear-wave speed of muscles, the suitable measurement depth is recommended to be within 3–5 cm that would be feasible or ideal in the present conditions and system. When depth increased to 6 cm, the shear wave failed to be detected with the use of a linear probe (SL10–2 linear probe). If more deeper tissue (>6 cm) is desired to be evaluated, lower frequency convex probe is recommended [34]. However, the subcutaneous adipose tissue also effects the depth of muscle. Thick superficial fat layers and greater BMI also effect the propagation of the shear waves and create artifacts as “holes” or areas of very high/low stiffness in the elastogram. A consequence of this is that the stiffness value might depend on the ROI size and position [25, 35].
-
Stiffness values also depend on the transducers and machines from the different vendors, presets, acoustic methods and calculation formulas use. The yield curve of increased stiffness during contraction differs between muscles and depends on the intensity of the force and torque as well as on fascicle length. Significant stiffness differences are observed between various muscles. In humans, reported shear modulus values at rest range between 3.1 kPa in the biceps brachii and 42.8 kPa in the masseter in vivo [36, 37]; thus, it can be said that stiffness values of muscles are case-specific. Furthermore, muscle contraction is one of the major factors affecting stiffness. At higher contraction levels, stiffness can not always be measured as shear waves in more rigid tissues because it may propagate too fast for some ultrasound systems to be properly tracked. Above a certain stiffness threshold, which depends on the performances of the equipment (maximal value v: 16 m/s or G = 266 kPa or E = 800 kPa), the measurement quality deteriorates and SWE cannot properly measure tissue stiffness. After an intense eccentric contraction, stiffness continues to increase for several days as a consequence of muscle damage. Especially during contraction diffuse heterogeneities were reported as well as stiffness differences along the longitudinal axis of muscles. This heterogeneous pattern of stiffness probably reflects the non-synchronization of motor units. Heterogeneity in the transversal axis of muscle have also been observed but showed some inconsistencies: both higher and lower stiffness was found in the deep part of the muscle than in the superficial. SWE measurements decrease with increasing depth and presence of bone below the area measured which makes it difficult to establish cut-off values (Table 21.1) [33].
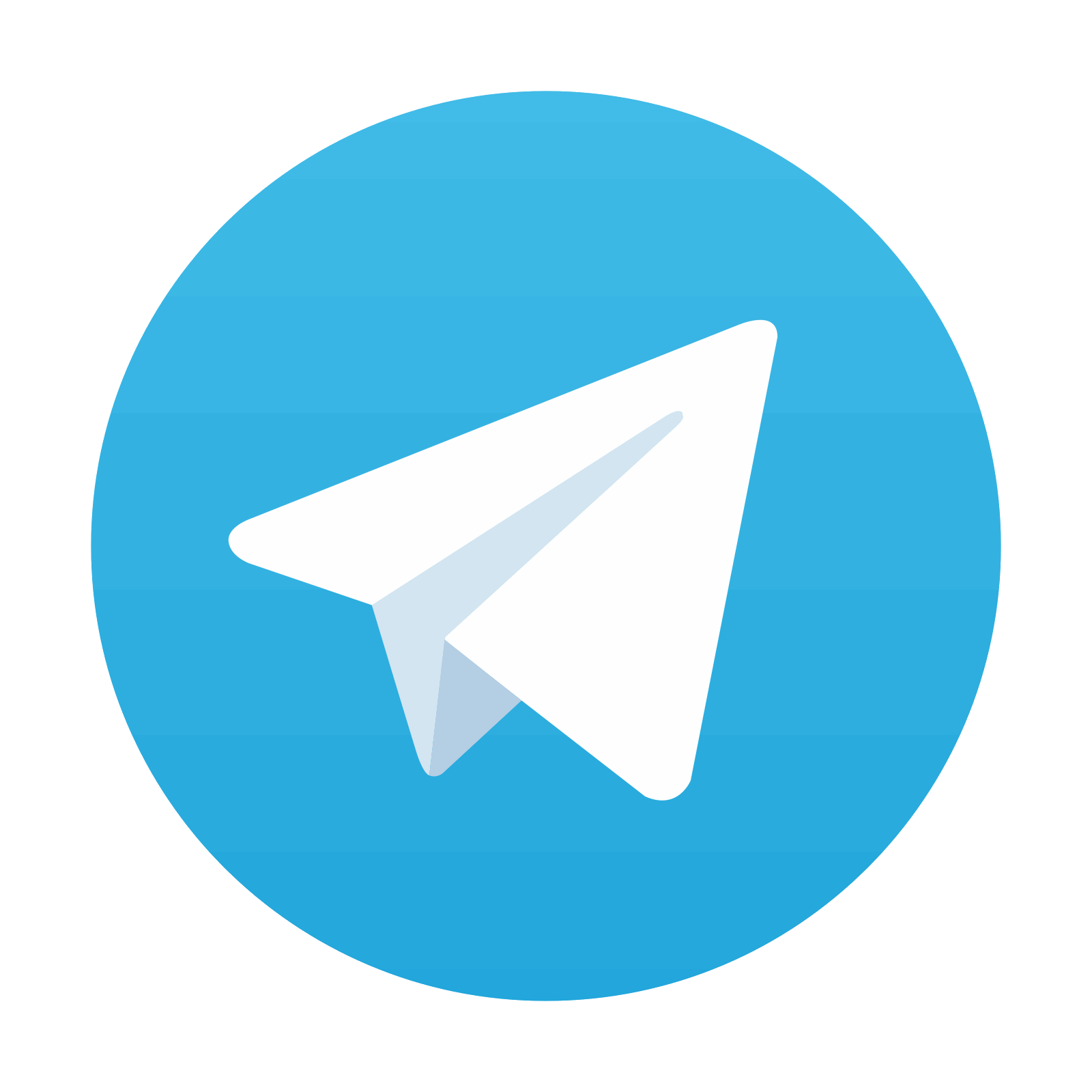
Stay updated, free dental videos. Join our Telegram channel

VIDEdental - Online dental courses
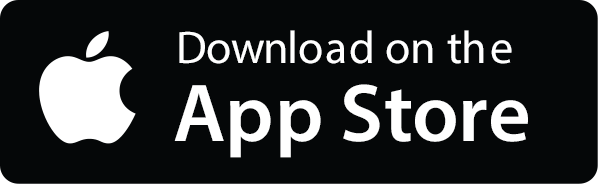
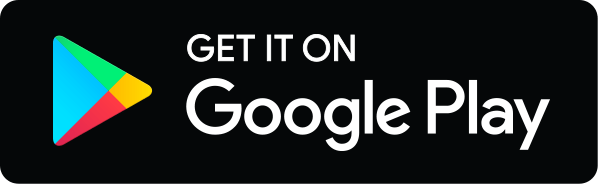