div epub:type=”chapter” role=”doc-chapter”>
K. Orhan (ed.)Ultrasonography in Dentomaxillofacial Diagnosticshttps://doi.org/10.1007/978-3-030-62179-7_15
15. USG Imaging in Orthodontics
UltrasonographyOrthodonticsDentistryDiagnosisImaging
15.1 Introduction
Orthodontics is the art and science that considers harmonizing crowded teeth, jaw relationships, abnormal teeth bites, and circumoral muscles and deals with jaw orthopedics in children/adults that may need surgery-assistant prosedures [1].
The understanding of the complex anatomy, correlations, and adjacent structures of the craniofacial skeleton is essential for treatment planning and management. Radiographic scanning is the complementary part of the entire evaluation of the orthodontic cases. In orthodontic practice, the aim of the imaging involves ensuring additional information for the diagnosis of skeletal/dental problems, soft tissues, and their interrelationships [2].
The radiographic findings obtained from conventional diagnostic tools (e.g., panoramic and lateral cephalometric images) have been integrated with clinical data for orthodontic diagnosis and planning, assessment of growth and development, and evaluation of treatment course and outcomes [2].
Every technique has its own advantages, disadvantages, and limitations [3]. However, the potential risks of radiography, some investigators have been directed to the new methodologies [4]. Ultrasonography (USG) has been utilized in the medical field as a diagnostic and therapeutic device [5]. Ultrasound is a recently introduced field of application in dentistry. Since the first study by Baum et al. [6], in dental practice, many different and new usages of Ultrasound imaging have been stated.
The aim of this chapter is to give an overview of the applications of USG in orthodontic clinics and broadening researchers’ horizons depending on the recent studies in the literature.
15.2 Evaluation of the Muscles of Mastication
Orthodontic treatment planning is, not to stand completely on biomechanical considerations, but it needs consideration of the maxillofacial muscular component of each case. The craniofacial muscles have assumed to be a great significance in treatment stages, including the etiology and active treatment of occlusal problems and deformation of the jaw, and the stability of treatment [7].

(a) Masseter muscle angle is defined as the angle between the FHP (Frankfort Horizontal Plane) and ABM (Anterior Border of Masseter muscle). (b–d) Schematic representation of the ultrasound transducer placement on volunteer for the (a) masseter, (b) anterior portion of temporalis, (c) sternocleidomastoid muscle, (d) levator labii superioris, (e) zygomaticus major, (f) orbicularis oris, and (g) anterior digastric muscles
Ultrasound has been represented as a reliable imaging method for accurately measuring the thickness and cross-sectional area of the masticatory muscles and for calculating changes in local cross-sectional parameters of the craniofacial muscle groups in vivo [11–21].
The thickness measurement has been utilized commonly in studies that evaluated Ultrasound imaging of masticatory muscles. Also, the crosssections, transversal areas, and the transverse dimensions have been evaluated [13, 22–24]. The images have obtained unilaterally and bilaterally, in the course of relaxation and/or contraction [25].
15.2.1 Masseter

Transverse ultrasound image of (a) relaxed and (b) contracted left masseter muscle demonstrating the measurement of length (horizontal dotted line), thickness (vertical dotted lines), and cross-sectional area (CSA)
Several studies investigated the measurements of the masseter muscle by USG within different conditions, including correlations between the thickness of masseter and facial morphology [15, 19, 20, 28–33], dental arch width [34, 35], thickness of alveolar process, mandibular symphysis, mandibular inclination [20], and integrated orthodontic treatment/functional appliances outcome [36–39].
USG has been utilized for thickness and/or cross-sections to explore the interactions with temporomandibular joint dysfunction (TMD), muscle palpation pain, facial morphology, bite force, and occlusal factors, specifically of the incisors and molars [15].
It has also evaluated the relationships between the masseter muscle volume and selected cephalometric values with USG [40].
In the literature, we determined the significant inhomogeneity in the published studies’ data. The measurement of the masseter muscle with ultrasound imaging is offered in different analyzes as an accurate method, reliable, and reproducible. However, in future researches, to increase the value of the methodology and results of the studies, data may need to be exhibited and analyzed in new hypothesis tests, also taking into account the heterogeneity of the studies.
15.2.2 Temporalis
After the masseter muscle, the most second masticatory muscle studied [25].
A study conducted by Rasheed et al. [41], reported that patients with an open or deep bite in the mixed dentition have a statistically thicker anterior temporalis muscle than patients with normal occlusion. Castelo et al. [42] compared the normal and crossbite sides, the mixed-crossbite group presented a significantly thicker anterior temporalis muscle at rest for the crossbite side than the normal side.
15.2.3 Other Muscles of the Stomatognathic System
The medial and lateral pterygoid muscles have not been assessed with USG, as they are not superficial muscles, and thus they do not diagnose on the ultrasound imaging, clearly [25].

Gray-scale transverse (a) and longitudinal (b) USG of the right anterior digastric muscle measured using electronic cursors to instantaneously calculate the cross-sectional area. (a) Transversal ultrasound image of the left anterior digastric muscle (c, d). Image demonstrating the measurement of length (horizontal dotted line), thickness (vertical dotted line), and cross-sectional area (CSA) measurement made by manually tracing around the circumference of the muscle with an electronic caliper (d). DA Anterior belly of Digastrics Muscle, MH Mylohyoid Muscle, GH Geniohyoid muscle, GG Genioglossus muscle, SL Sublingual gland, M Mandible
Şatıroğlu et al. [45] investigated the thickness of the masseter, levator labii superioris, and zygomaticus major muscles by USG and evaluated the correlation between facial and masticatory muscle thickness and vertical facial morphology. The authors found that masseter muscle thickness was significantly associated with vertical facial measurements, but the facial muscles were not correlated with the vertical facial morphology.

(a) Scheme of application of transversal ultrasound transducer on the (a) upper and (b) lower lip. (b) Schematic drawing of the transversal image cross-section
A study by Coclici et al. [50] used ultrasound device for displaying the posttreatment muscular alterations in class II and class III malocclusion patients and measured the length, width, and cross-sectional area of the masseter and suprahyoid muscles (mylohyoid and geniohyoid muscle). Variable adaptive response to orthognathic surgery has been detected in the mandibular muscles.

Transverse USG of the left sternocleidomastoid muscle at rest (a), during dental clenching (b), flexion (c), and extension (d) of the head; the vertical dotted lines indicate the sites of muscle thickness measurements. SCM sternocleidomastoid muscle, LSM levator scapulae muscle, CA carotid artery
Future studies should standardize the methods and parameters for reducing errors and optimizing accuracy with a large-scale population. The use of ultrasound continues a promising option for the study of muscles of mastication.
In addition, Doppler sonography can be helpful for investigating the arteries in and around the masseter muscle and this method has the capability for evaluating pathological alterations in the muscles and arteries [25]. In a study conducted by Ariji et al. [52], the change of muscle thickness immediately after exercise showed a significant correlation with minimum blood-flow velocity.
15.3 Analysis of Tongue, Hyoid, and Swallowing
The tongue is a largely movable muscular organ within the orofacial region and for years, it has been theorized that the tongue size, postures, and functions must have a relationship to the surrounding oral cavity. It is assumed that the tongue size, postures, and functions have great importance for the etiology of malocclusions and dentofacial deformities [53].
Several methods are available for assessment of the tongue’s size in vivo: direct measurements [54], different impression techniques [55], and the fluid displacement method [56]. Recently, different imaging methods have been used in tongue volume assessment: cephalometrics [57], computed tomography (CT) [58], cone-beam computed tomography (CBCT) [59], and magnetic resonance imaging (MRI) [60]. However, all techniques have their own clinical indications, advantages, and disadvantages.
Two-dimensional (2D) ultrasound is used for tongue function evaluation such as swallowing [61, 62] and speech [63] as well as for estimating tongue thickness, and tongue volume [64]. Three-dimensional (3D) ultrasound is already performed for the tongue posture assessment [65], as a device for the evaluation of tongue function [66].
15.3.1 Tongue Volume
Wojtczak et al. [64] examined tongue volume that was obtained from the multiplication of the midsagittal cross-sectional images of the tongue by its width in transverse scans, using 2D USG. The correlation between the tongue volume and mandibular arch size [55], vertical facial height, chin position has been demonstrated in clinical studies [67]. Hren ve Barbič [68], aimed to evaluate tongue size and it has been found that tongue volumes are significantly greater in skeletal Class III patients than normal. Also, larger tongues correlate with more severe skeletal Class III malocclusion.
Hren ve Barbic [68] and Barbič et al. [53] have utilized 3D USG for evaluating tongue volume. During USG investigation, each patient sits in upright resting positions and their heads fixed with a strap, so that the Frankfurt horizontal line was parallel to the floor. The 3D convex transducer was positioned on the skin of mouth floor in the midsagittal plane, submentally. The tongue volume evaluation was performed using 4D VIEW program software. The 3D Ultrasound technologies provide a multi-planar image of the region of interest or of certain pathology as well as perform more accurate analyses of them [53].
15.3.2 Tongue Posture
Tongue posture has been described as an etiologic factor in malocclusion development, including anterior open bite and articulation disorders as well as that plays an important role in anterior open bite treatment planning and posttreatment stability [69, 70]. It is believed that the tongue resting posture to be even more important for the dentoalveolar development and dental occlusion than the tongue function during swallowing or speaking [71].In the view of total time, swallowing and speaking is too short to affect the balance of the forces acting on dentoalveolar development.
In the orofacial region, the tone and pressure of the resting tongue is one of the most important long-acting forces on the adjacent structures represented [72, 73]. Clinical evaluation of the tongue dynamics and resting postures are important parts of functional diagnostics [65]. A study by Kravanja et al. [74], who evaluated the tongue posture in children showed that the 3D ultrasound was found to be the most objective method to identify tongue posture in growing children and it could be an important device in functional diagnostics before, during, and after orthodontic treatment.
15.3.3 Tongue Movement
Tongue movement is related to some disorders, such as dysphagia. A better understanding of tongue movement in detail is important for the diagnosis and treatment of such diseases [75]. The tongue posture and function can be evaluated by clinical examination, including observation of tongue movements with lips apart and palpation of the temporalis and masseter muscles during swallowing. Because of anatomical limitations, these methods are not enough for objective evaluation [62]. Additional techniques have been developed and used for tongue movement evaluation, such as videofluoroscopy [76–78], electromyography [79–81], electromagnetic articulography [82], palatography [83], MRI [84], scintigraphy [85], and tongue pressure measurements [86]. Between these methods, the use of videofluoroscopy, which records the dynamic movement of radiopaque barium through the upper digestive tract by conventional X-rays, is considered as the standard criterion for the deglutition and dysphagia evaluation [87–90]. However, especially the disadvantages of irradiation, repeated evaluations are often avoided.

B + M mode ultrasonogram. The left side shows the B-mode image with the scan line (SL) of the ultrasound probe set in the middle of the tongue. The M-mode image (right side) illustrates movements of various anatomic structures along the SL (a). Duration and range of tongue movement in each phase were determined graphically. In the rest phase (R), the tongue tip is usually positioned on the lingual surfaces of incisors or is touching the incisive papilla. The swallowing act starts with the shovel phase (I), in which the tongue tip moves cranially, the middle third of the tongue becomes concave and this is reflected in the down-movement of the curve in the M-mode ultrasonogram. In the early transport phase (IIa), the tongue is moving cranially and distally, the middle third of the tongue is approaching the hard palate, and therefore the concavity is disappearing. The late transport phase (IIb) is characterized by minimal vertical movement of the tongue because of the distal transportation of saliva. In the early final phase (IIIa), the curve in the M-mode ultrasonogram drops because of the lowering of the mouth floor. In the late final phase (IIIb), the tongue returns to the rest position and this is reflected as a rise in the M-mode curve [93] (b). R rest phase; d distal, m mesial, D duration, R (mm) Range
Cheng et al. [96] found that there are significant correlations between tongue movement during swallowing and dentofacial forms using B + M-Mode sonography combined with the CST, especially in the amplitude of the early final phase. They concluded that as the arch length increased, the duration of swallowing prolonged significantly in the late final phase.
Peng et al. [97] stated that the tongue movements of mature swallowing and tongue-thrust swallowing can be differentiated with USG. Based on this study, Tongue-thrust swallowers had a longer late transport phase than mature swallowers, and the tongue speed was faster in the early final phase compared with mature swallowers.
Ardakani et al. [98] investigated the swallowing patterns of the tongue using B-mode Sonography. They concluded that the majority of abnormal or inconsistent swallowing patterns were detected in patients of mandibular prognathism.
Ovsenik et al. [71] compared the swallowing pattern and tongue function during swallowing in children with unilateral posterior crossbite (ULCB) in deciduous dentition by B + M-mode USG. The ultrasound analysis showed that duration, range, and speed of the tongue movements during swallowing significantly differ between children with and without ULCB.
In another study, Vaishnevi et al. [99], investigated the relationship between tongue movement and facial morphology in three types of malocclusion and found that the skeletal class III cases have prolonged duration of tongue movement and greater motion magnitude in the early final phase (III A) of swallowing. Also, there is a decrease in the motion range and duration of swallowing in the skeletal class II individuals.
15.3.4 Hyoid Bone Displacement
The measurements of tongue, hyoid, and laryngeal movements have been used to evaluate swallowing in USG studies [100–107]. The hyoid bone is the point of attachment for muscular and nonmuscular tissues of the floor of the mouth, tongue, and larynx and its movement functions as a marker of the integrity of the hyoid/larynx/epiglottis unit [100]. Under normal physiologic conditions, timely and adequate laryngeal elevation along with hyoid bone movement is an important part of the swallowing movement [108, 109]. The hyoid bone is easily viewed on USG in the sagittal plane as a hyperechoic area with a posterior acoustic.

Sagittal view in the submandibular position using a convex transducer between the mentum and the hyoid bone (a). The sonogram shows the tongue and the floor of the mouth (b). The insets show the transducer position on the skin. GG genioglossus, GH geniohyoid, M Mandible, H Hyoid bone, P Palate, SLF Sublingual fat, and TS Tongue surface
Yabunaka et al. [111] stated that the trajectory of the hyoid bone in the sagittal plane can be a feasible option for detecting some anomalies in swallowing. Similarly, Chen et al. [112] demonstrated that submental USG is a reliable and accurate technique for the hyoid bone movement assessment and that could be an aid in dysphagia screening and evaluation.
Effective bolus flow and pharyngeal clearing need enough hyoid bone movement during swallowing. Feng et al. [113] evaluated the association between the geniohyoid muscle size-function and hyoid bone movement during swallowing. The authors measured the cross-sectional area of the geniohyoid muscle, geniohyoid muscle contraction velocity, and the hyoid bone displacement in healthy young adults. A correlation has been found between the size of the geniohyoid muscle and hyoid bone movement.
15.4 Evaluation of the Airway
Airway obstruction and mouth breathing are among the etiological factors of malocclusion. Therefore, airway evaluation has great importance in clinical practice. The assessment is performed using lateral cephalometric radiography, commonly [114]. Cone-beam Computed Tomography scans can be utilized for assessing the morphology and mechanical behavior of the upper airway bony and soft tissue structures [115]. Also, various methods, including MRI, endoscopic procedures (e.g., fiber-optic, nasopharyngoscopy, fluoroscopy), acoustic reflection, and optical coherence tomography are feasible to display these structures [116–119].

Schematic diagram with USG parameters to evaluate the airway. Ultrasound measurements at various levels, 1 Cross-sectional area at base of tongue. 2 Distance from hyoid bone to mentum. 3 Distance from skin to hyoid bone. 4 Soft tissue thickness at level of thyrohyoid membrane. 5 Distance from skin to epiglottis midway between thyroid cartilage and hyoid bone. 6 Distance from skin to anterior commissure of true vocal cords. 7 Soft tissue thickness at level of thyroid isthmus. 8 Soft tissue thickness at level of suprasternal notch. TB Tongue base, H Hyoid bone, M Mentum, E Epiglottis, TC Thyroid cartilage, CC Cricoid cartilage, VC vocal cords, TI Thyroid isthmus, S manubrium sterni
15.5 The Temporomandibular Joint Evaluation
Temporomandibular joint disorder (TMD) is a general term for disorders affecting the masticatory muscles, temporomandibular joint (TMJ)-related structures, or all [124]. The prevalence of TMD is ranging from 10% to 70%, among the general population [125]. There may be different causes and different specific conditions in the etiology of TMD [126]. The TMDs may cause problems in some of the orthodontic patients, therefore TMJ assessment before, during, and after orthodontic treatment have great significance [127]. There are several methods and techniques used in the diagnosis of TMD, along with the basic clinical examination [126].
Panoramic radiography, conventional (linear or complex motion) tomography, helical or multi-slice computed tomography (CT), and cone-beam computed tomography (CBCT) are used to view the bony components, and magnetic resonance imaging (MRI) is used to evaluate the soft tissue components (discs) of the TMJ [128]. Bone scintigraphy can help for diagnosis of the osteoarthritis and joint inflammation [129, 130]. However, these methods have advantages and limitations to each.

Method of using ultrasound in TMJ. (a) Horizontal positioning, transverse image of the TMJ, and transverse section of sonography of the right TMJ obtained while the patient was in the closed-mouth position. (b) Anatomical landmarks observed in transversal/axial slice. (c) Vertical positioning, coronal/sagittal image of the TMJ, and coronal section of sonography of the left TMJ obtained while the patient was in the closed-mouth position. (d) Anatomical landmarks observed in coronal view. TMJ temporomandibular joint, AD Articular Disc, MC Mandibular condyle, MM Masseter muscle, T Temporal bone (depending on the angulations of the USG probe)
15.6 Determination of Soft Tissue Thickness at Orthodontic Miniscrew Placement Sites
Loss of anchorage in orthodontic treatments is often an important problem that risks treatment outcomes. Temporary skeletal anchorage instruments have been indicated as a reliable solution for situations where anchorage is critical. The use of orthodontic miniscrews in clinical orthodontics has revolutionized anchorage control, opening a new era [132].
The stability and success of orthodontic miniscrews depend on various factors, including the screw implantation site, the miniscrew angulation, the quality and quantity of cortical bone, the insertion and removal torques, the degree of miniscrew to bone contact, inflammation degree of the peri-orthodontic miniscrew tissues, the soft tissues thickness and mobility, the patient’s craniofacial morphology, and the screw dimensions [133–139].
Risk of failure of orthodontic miniscrews surrounded by nonkeratinized mucosa is higher than for screws surrounded by keratinized mucosa, for the soft tissue component of stability [140]. In the different candidate, areas for screw placement have different soft tissue thicknesses. Therefore, evaluation of the quantitative differences in gingival thickness for miniscrew implantation is one of the significant factors affecting surgical success [141].
Measurements on the thickness of oral mucosa can be acquired by direct methods, such as using a needle or periodontal probe with an endodontic file stopper and biopsy, or indirect measurement using radiographic images. USG is an alternative method that has the potential for providing an evaluation of the soft tissue thickness [142].
Cha et al. [141] evaluated the gingival thickness of potential sites for miniscrew placement in the buccal-attached gingiva and the palatal masticatory mucosa. Mucosal thickness was measured intraorally with an ultrasonic gingival thickness meter (5 MHz).
A study by Parmar et al. [132] aimed to examine the soft tissue thicknesses at potential miniscrew implantation zones and to prescribe a guideline for miniscrew selection in orthodontic clinics. The measurements were performed with A-mode ultrasound device that uses the pulse-echo principle with the frequency was 10 MHz at 10%. The transducer was placed perpendicular to the most gingival surface.
Cha et al. [141] and Parmar et al. [132] concluded that evaluation of the gingival tissues could help in selecting a proper miniscrew in orthodontic practice.
Schulze et al. [142] reported that using B-mode and A-mode ultrasonography is acceptable in various clinical practices for measuring the mucosal thickness accurately. However, B-mode USG is a capable device for soft tissue diagnostics, that needs a small transducer for measurements in the oral cavity.
Although the quantity and quality of cortical bone greatly influenced the stability of mini-screws, also the width of the attached gingiva on the buccal and palatal surfaces in the interdental areas must be considered before surgery [143]. Maximum retention can be obtained when an adequate length of the screw is placed in areas of thin gingival tissue and thick cortical bone [144].
The validity and reliability of USG were shown for measuring soft tissue thickness in different anatomical locations of the oral cavity [145–149], and that offers great potential in presurgical assessment for placement of orthodontic miniscrew placement.
15.7 Determining Pubertal Growth and Bone Age
In the human beings, skeletal maturation has a great value for the detection of growth and differentiation processes [150, 151]. Bone age is an important indicator of the skeletal and biological maturity of an individual. The knowledge of the skeletal maturation and the stage of growth can provide useful information for many clinical practices as well as in orthodontic procedures such as treatment planning, the timing of treatment, and selection of the treatment method [152].
Radiological indicators have been used for bone age estimation [153]. For this, several techniques are generally utilized based on hand-wrist radiographs. In clinical practice, the Greulich-Pyle (an atlas method which compares the radiograph of the individual with the nearest standard radiograph in the atlas) and Tanner-Whitehouse (a scoring method which focuses on skeletal maturity for each patient hand and wrist bone) methods are preferred commonly [154–156].
In recent years, because of the possible side effects and damages of ionizing radiation, there is an increasing focus on the establishment of nonionizing imaging methods for age estimation [157]. Some researchers have used USG, which has many advantages and as an ionized radiation-free imaging technique, to estimate bone age [157–160].
The bone age estimation by ultrasonography is not a new method. In children, the hip [158], iliac and radius bones [159], and ossification center of the wrist [160] have been used as sonographic landmarks for determination of the skeletal age previously.
Carpenter and Lester [161] stated that there was a significant difference between chronologic age and bone age in the different regions of hand and genders. Also, in children under age 10 years, the entire hand should be taken to consider for evaluation of bone age, maybe with less interest on the carpal bones, they may cause over- and under-estimated results greatly. They concluded that bone age estimation based on the metacarpals and phalanxes more accurate than wrist and carpal bone age readings.

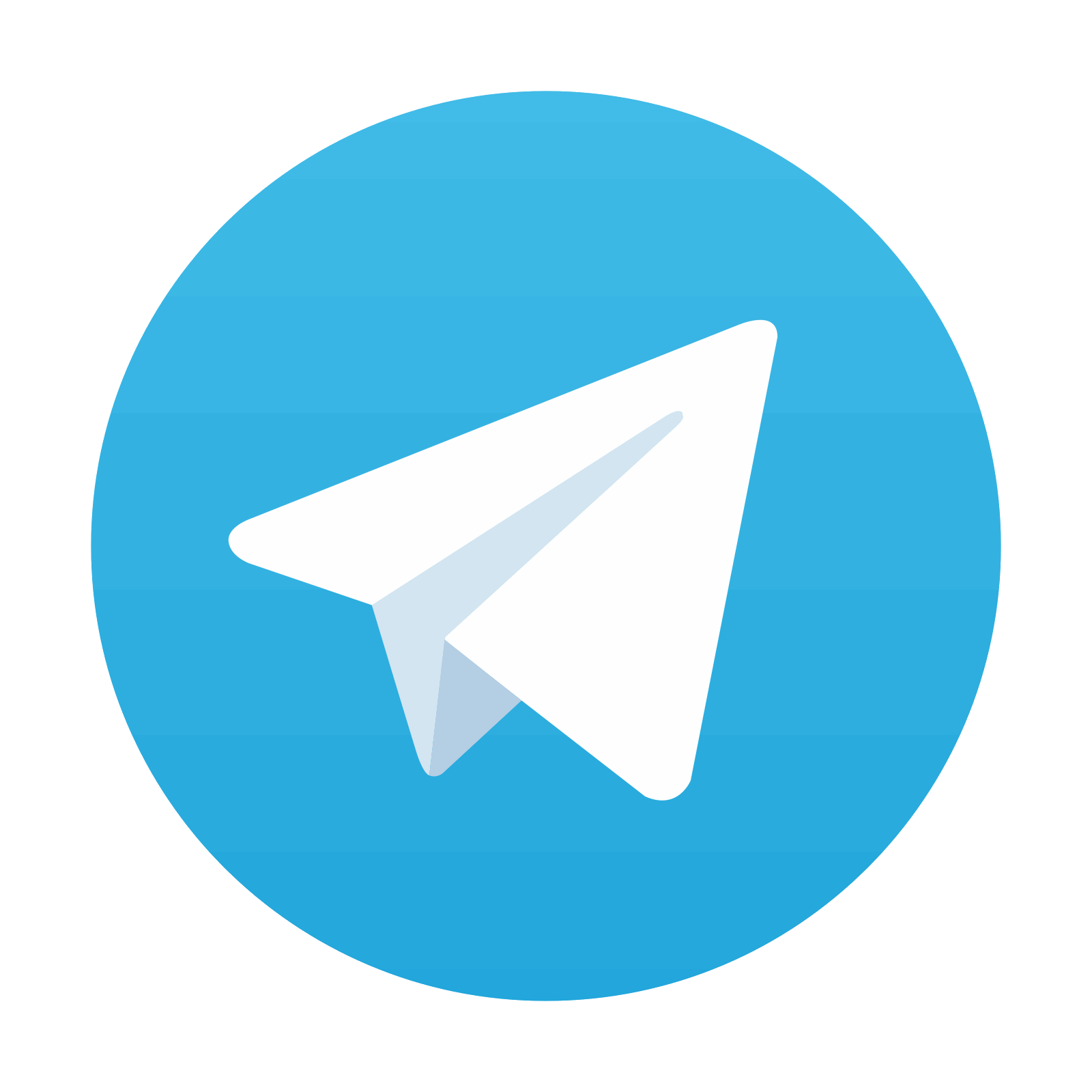
Stay updated, free dental videos. Join our Telegram channel

VIDEdental - Online dental courses
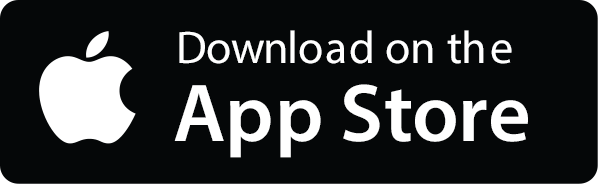
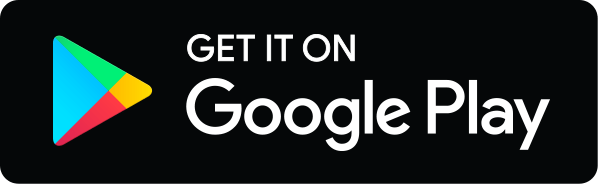